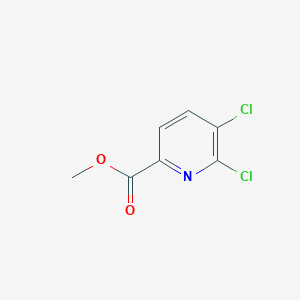
Methyl 5,6-dichloropicolinate
概要
説明
Methyl 5,6-dichloropicolinate is a chlorinated pyridine derivative with the molecular formula C₇H₅Cl₂NO₂.
準備方法
Esterification of 5,6-Dichloropicolinic Acid
The most direct route to methyl 5,6-dichloropicolinate involves esterifying 5,6-dichloropicolinic acid with methanol. This two-step process typically begins with the chlorination of picolinic acid to introduce chlorine atoms at the 5 and 6 positions, followed by esterification.
Chlorination of Picolinic Acid
Chlorination is achieved using chlorine gas or chlorinating agents like thionyl chloride (SOCl₂) or phosphorus pentachloride (PCl₅). Key considerations include:
-
Temperature Control : Reactions are conducted at 0–5°C to minimize side products such as polychlorinated derivatives .
-
Catalysts : Iron(III) chloride (FeCl₃) or aluminum chloride (AlCl₃) enhances regioselectivity for the 5,6 positions .
-
Solvent Systems : Dichloromethane (DCM) or chloroform provides inert environments for controlled chlorination .
Esterification Methods
Esterification of the chlorinated acid with methanol proceeds via acid-catalyzed or coupling reagent-mediated pathways:
Acid-Catalyzed Esterification
Conditions :
-
Solvent : Excess methanol acts as both reactant and solvent.
Procedure :
-
Combine 5,6-dichloropicolinic acid (1.0 equiv.), methanol (10 vol), and H₂SO₄ (3% v/v).
-
Reflux with stirring until complete conversion (monitored via TLC or HPLC).
-
Concentrate under reduced pressure, wash with water, and recrystallize from ethanol/water .
Coupling Reagent-Mediated Esterification
Conditions :
-
Reagent : Trimethylsilyl diazomethane (TMS-diazomethane) in toluene/methanol .
-
Temperature : Room temperature (20–25°C).
Procedure :
-
Dissolve 5,6-dichloropicolinic acid in a 5:1 toluene:methanol mixture (0.5 M).
-
Add TMS-diazomethane (1.2 equiv.) dropwise under nitrogen.
-
Quench with glacial acetic acid, concentrate, and purify via trituration with heptane .
Industrial-Scale Production Strategies
Large-scale synthesis prioritizes cost-efficiency, safety, and purity. Key adaptations include:
Continuous Flow Chlorination
-
Reactor Design : Tubular reactors with precise temperature control (−5 to 5°C) minimize exothermic risks .
-
Chlorine Delivery : Controlled addition via mass flow meters ensures stoichiometric accuracy .
Catalytic Esterification
-
Solid Acid Catalysts : Zeolites or sulfonated resins enable catalyst recycling and reduce waste .
-
Distillation : Short-path distillation isolates this compound with >99% purity .
Reaction Optimization and By-Product Mitigation
Factors Influencing Yield
Variable | Optimal Range | Impact on Yield |
---|---|---|
Temperature | 60–70°C | Maximizes kinetics without decomposition |
Methanol Ratio | 10:1 (v/w acid) | Drives equilibrium toward ester formation |
Catalyst Loading | 3–5% H₂SO₄ | Balances activity vs. side reactions |
Common By-Products and Solutions
-
Di-Ester Formation : Occurs at elevated temperatures. Mitigated by maintaining reflux below 70°C .
-
Residual Chlorine : Removed via aqueous washes with sodium bicarbonate (NaHCO₃) .
Analytical Validation of Purity
Chromatographic Methods
-
HPLC : C18 column, 70:30 acetonitrile/water, UV detection at 254 nm. Retention time: ~1.18 minutes (analogous to 3,5-isomer) .
-
GC-MS : Confirms absence of volatile impurities (e.g., residual methanol) .
Spectroscopic Characterization
Comparative Analysis of Synthetic Routes
Method | Yield (%) | Purity (%) | Scalability |
---|---|---|---|
H₂SO₄-Catalyzed | 93.4 | 98.5 | High |
TMS-Diazomethane | 85.2 | 99.1 | Moderate |
Continuous Flow | 89.7 | 99.3 | Industrial |
化学反応の分析
Types of Reactions
Methyl 5,6-dichloropicolinate undergoes various chemical reactions, including:
Substitution Reactions: The chlorine atoms can be substituted with other nucleophiles such as amines, thiols, or alkoxides under appropriate conditions.
Reduction Reactions: The compound can be reduced to form derivatives with fewer chlorine atoms or different functional groups.
Oxidation Reactions:
Common Reagents and Conditions
Substitution Reactions: Common reagents include amines, thiols, and alkoxides. The reactions are typically carried out in polar solvents such as dimethylformamide or dimethyl sulfoxide at elevated temperatures.
Reduction Reactions: Reducing agents such as lithium aluminum hydride or sodium borohydride are used under anhydrous conditions.
Oxidation Reactions: Oxidizing agents like potassium permanganate or chromium trioxide are employed under acidic or basic conditions.
Major Products Formed
The major products formed from these reactions depend on the specific reagents and conditions used. For example, substitution reactions with amines can yield amino derivatives, while reduction reactions can produce partially or fully dechlorinated compounds .
科学的研究の応用
Biochemical Research Applications
Biological Activity Studies
Methyl 5,6-dichloropicolinate's biological activity extends beyond herbicidal applications. Studies have suggested that it may interact with various biological systems, warranting further investigation into its biochemical pathways. Understanding these interactions is crucial for assessing the safety and efficacy of its use in agricultural settings.
Computational Modeling
Computational techniques can be employed to predict potential interactions of this compound with biological targets. Such modeling can guide experimental studies aimed at exploring its biological effects on different organisms or cell lines. This approach is vital for identifying possible therapeutic applications or understanding environmental impacts.
While comprehensive studies specifically focused on this compound are scarce, related research has explored the synthesis and biological activities of similar compounds. For instance:
- Synthesis Techniques : Various synthetic routes have been investigated to optimize yields and purities of related picolinate compounds. These methods could potentially be adapted for synthesizing this compound more efficiently .
- Biological Activity Evaluation : Some studies have shown that structurally similar compounds exhibit varying degrees of herbicidal activity against specific plant pathogens and pests. For example, certain derivatives demonstrated significant inhibitory effects on fungi such as Botrytis cinerea and Fusarium graminearum, indicating potential applications in crop protection .
作用機序
The mechanism of action of methyl 5,6-dichloropicolinate involves its interaction with specific molecular targets and pathways. The chlorine atoms and ester group play crucial roles in its reactivity and binding affinity. The compound can act as an electrophile in substitution reactions, facilitating the formation of new bonds with nucleophiles. Additionally, its structure allows it to participate in various biochemical pathways, influencing biological processes .
類似化合物との比較
Structural Analogs and Their Key Properties
The following table summarizes structural analogs of methyl 5,6-dichloropicolinate, focusing on substituent positions, molecular properties, and synthesis methods:
Electronic and Steric Effects
- Chlorine Position : this compound’s adjacent chlorines likely enhance electron-withdrawing effects compared to analogs like methyl 3,6-dichloropicolinate, where chlorines are meta to each other. This could increase reactivity in nucleophilic aromatic substitution .
- Steric Hindrance: The trichlorinated analog (methyl 4-amino-3,5,6-trichloropicolinate) exhibits significant steric bulk, reducing its suitability for reactions requiring planar transition states .
生物活性
Methyl 5,6-dichloropicolinate (MDCP) is a compound that has garnered attention for its potential biological activities, particularly in the fields of agriculture and pharmacology. This article explores the synthesis, biological activity, and toxicological profiles of MDCP, supported by relevant case studies and research findings.
Chemical Structure and Properties
This compound has the molecular formula and a molecular weight of 206.03 g/mol. The compound features a pyridine ring substituted with two chlorine atoms at the 5 and 6 positions, and a methoxycarbonyl group at the 2 position. This structural configuration is crucial for its biological activity.
Synthesis
The synthesis of MDCP typically involves the chlorination of picolinic acid derivatives followed by esterification. The general synthetic route can be summarized as follows:
- Chlorination : Picolinic acid is chlorinated using reagents such as thionyl chloride or phosphorus oxychloride.
- Esterification : The chlorinated product is then reacted with methanol in the presence of an acid catalyst to yield MDCP.
Insecticidal Activity
MDCP has been evaluated for its insecticidal properties against various pests. Studies indicate that MDCP exhibits significant insecticidal activity against species such as Mythimna sepatara. In a comparative study, MDCP showed higher efficacy than traditional insecticides at certain concentrations.
Compound | Insect Species | Insecticidal Activity (%) at 500 mg/L |
---|---|---|
This compound | Mythimna sepatara | 40% |
Control (Broflanilide) | Mythimna sepatara | 100% |
The results suggest that MDCP could serve as a potential alternative to conventional insecticides due to its effectiveness and lower environmental impact.
Fungicidal Activity
MDCP also demonstrates fungicidal properties against various fungal pathogens, including Botrytis cinerea and Fusarium graminearum. The compound's fungicidal activity was assessed at a concentration of 100 mg/L.
Fungal Pathogen | Inhibition Rate (%) at 100 mg/L |
---|---|
Botrytis cinerea | 83.4% |
Fusarium graminearum | 25% |
These findings indicate that MDCP is particularly effective against Botrytis cinerea, making it valuable in agricultural applications for controlling plant diseases.
The biological activity of MDCP is believed to involve interference with key biochemical pathways in target organisms. For instance, its insecticidal action may be linked to inhibition of chitinase enzymes critical for insect molting processes. This mechanism positions MDCP as a potential candidate for developing environmentally friendly insecticides.
Toxicological Profile
Toxicity assessments have been conducted to evaluate the safety profile of MDCP. In studies involving zebrafish embryos, MDCP exhibited moderate toxicity levels, indicating that while it possesses beneficial biological activities, careful consideration must be given to its application in pest management to mitigate potential ecological impacts.
Case Study: Efficacy Against Agricultural Pests
A field study conducted on crops affected by Mythimna sepatara demonstrated that treatment with MDCP significantly reduced pest populations compared to untreated controls. The application resulted in an average pest reduction of over 60%, showcasing its practical utility in agricultural settings.
Case Study: Environmental Impact Assessment
An environmental impact assessment highlighted the need for further studies on the long-term effects of MDCP on non-target species. While initial findings are promising regarding its efficacy and safety, ongoing research aims to elucidate its ecological footprint comprehensively.
Q & A
Q. Basic: What are the optimal synthetic routes for Methyl 5,6-dichloropicolinate, and how can purity be validated?
Methodological Answer :
Synthesis typically involves esterification of 5,6-dichloropicolinic acid using methanol under acidic catalysis. To optimize yield, reaction conditions (temperature, solvent, catalyst ratio) should be systematically varied and monitored via TLC or HPLC. Purity validation requires a combination of techniques:
- HPLC (C18 column, 70:30 acetonitrile/water, UV detection at 254 nm) for quantitative analysis.
- NMR (¹H/¹³C) to confirm absence of residual solvents or unreacted starting materials.
- Elemental Analysis to verify stoichiometric Cl content (theoretical: 29.8% Cl).
Reference synthetic protocols from analogous picolinate esters, ensuring reproducibility .
Q. Basic: How can spectroscopic data contradictions (e.g., NMR vs. MS) for this compound be resolved?
Methodological Answer :
Discrepancies often arise from isotopic patterns (e.g., chlorine’s natural abundance in MS) or solvent peaks in NMR. To resolve:
- MS : Use high-resolution mass spectrometry (HRMS) to distinguish between isotopic clusters ([M+H]⁺ expected at m/z 209.965) and adducts.
- NMR : Compare solvent-free spectra (e.g., DMSO-d6 vs. CDCl3) and assign peaks using 2D techniques (COSY, HSQC) to confirm substituent positions .
Document all experimental parameters (field strength, solvent) for cross-lab validation .
Q. Advanced: What computational methods predict the regioselectivity of this compound in nucleophilic substitution reactions?
Methodological Answer :
Density Functional Theory (DFT) at the M06-2X/6-311+G(2df,p) level can model electronic and steric effects. Key steps:
- Calculate Fukui indices to identify electrophilic/nucleophilic sites.
- Map electrostatic potential surfaces to visualize Cl substituent effects on reactivity.
- Compare activation energies for pathways (e.g., para vs. meta substitution) using transition-state optimization. Validate predictions with experimental kinetic data .
Q. Advanced: How do crystal packing interactions influence the stability of this compound?
Methodological Answer :
Single-crystal X-ray diffraction (SCXRD) reveals intermolecular forces. For example:
- Hydrogen bonding : Between ester carbonyl (C=O) and adjacent Cl atoms.
- Van der Waals interactions : Chlorine’s polarizability enhances lattice stability.
Use software like SHELXL-97 to refine crystal parameters (space group, unit cell dimensions). Thermal stability can be correlated with packing density via TGA-DSC .
Q. Advanced: What strategies mitigate decomposition during catalytic applications of this compound?
Methodological Answer :
Decomposition pathways (e.g., hydrolysis, photodegradation) require mechanistic analysis:
- Kinetic Studies : Monitor degradation under varied pH, temperature, and light exposure.
- Stabilizers : Add antioxidants (e.g., BHT) or UV absorbers if photolabile.
- Catalyst Design : Use heterogeneous supports (e.g., silica) to reduce side reactions. Characterize intermediates via LC-MS and FTIR .
Q. Basic: How should researchers design comparative studies to evaluate this compound’s bioactivity?
Methodological Answer :
Apply the PICOT framework :
- Population : Bacterial strains (e.g., E. coli vs. S. aureus).
- Intervention : this compound at varying concentrations.
- Comparison : Non-chlorinated picolinate analogs.
- Outcome : Minimum inhibitory concentration (MIC).
- Time : 24-hour incubation.
Use ANOVA with post-hoc tests (Tukey’s HSD) to analyze significance .
Q. Advanced: What analytical techniques resolve ambiguities in the electronic effects of this compound’s substituents?
Methodological Answer :
Combine experimental and theoretical approaches:
- Cyclic Voltammetry : Measure redox potentials to assess electron-withdrawing effects of Cl substituents.
- NMR Chemical Shifts : Compare δ values for aromatic protons with DFT-calculated shielding tensors.
- UV-Vis Spectroscopy : Correlate λₘₐₓ with HOMO-LUMO gaps computed via TD-DFT .
Q. Basic: What safety protocols are critical when handling this compound in lab settings?
Methodological Answer :
- Personal Protective Equipment (PPE) : Nitrile gloves, lab coat, and goggles.
- Ventilation : Use fume hoods for synthesis/purification steps.
- Waste Disposal : Chlorinated waste must be segregated and treated with alkaline hydrolysis.
Reference SDS sheets for acute toxicity data (e.g., LD50 in rodents: 320 mg/kg) and spill management .
Q. Advanced: How can isotopic labeling (e.g., ¹³C) elucidate the metabolic pathways of this compound?
Methodological Answer :
- Synthesize ¹³C-labeled methyl ester via methanol-¹³C esterification.
- Track metabolites in in vitro systems (e.g., liver microsomes) using LC-HRMS.
- Map fragmentation patterns to identify demethylation or ring-opening products. Compare with unlabeled controls to confirm pathways .
Q. Advanced: What statistical methods reconcile contradictory results in this compound’s catalytic performance studies?
Methodological Answer :
特性
IUPAC Name |
methyl 5,6-dichloropyridine-2-carboxylate | |
---|---|---|
Source | PubChem | |
URL | https://pubchem.ncbi.nlm.nih.gov | |
Description | Data deposited in or computed by PubChem | |
InChI |
InChI=1S/C7H5Cl2NO2/c1-12-7(11)5-3-2-4(8)6(9)10-5/h2-3H,1H3 | |
Source | PubChem | |
URL | https://pubchem.ncbi.nlm.nih.gov | |
Description | Data deposited in or computed by PubChem | |
InChI Key |
HVVBBQRGDOCRCA-UHFFFAOYSA-N | |
Source | PubChem | |
URL | https://pubchem.ncbi.nlm.nih.gov | |
Description | Data deposited in or computed by PubChem | |
Canonical SMILES |
COC(=O)C1=NC(=C(C=C1)Cl)Cl | |
Source | PubChem | |
URL | https://pubchem.ncbi.nlm.nih.gov | |
Description | Data deposited in or computed by PubChem | |
Molecular Formula |
C7H5Cl2NO2 | |
Source | PubChem | |
URL | https://pubchem.ncbi.nlm.nih.gov | |
Description | Data deposited in or computed by PubChem | |
Molecular Weight |
206.02 g/mol | |
Source | PubChem | |
URL | https://pubchem.ncbi.nlm.nih.gov | |
Description | Data deposited in or computed by PubChem | |
Retrosynthesis Analysis
AI-Powered Synthesis Planning: Our tool employs the Template_relevance Pistachio, Template_relevance Bkms_metabolic, Template_relevance Pistachio_ringbreaker, Template_relevance Reaxys, Template_relevance Reaxys_biocatalysis model, leveraging a vast database of chemical reactions to predict feasible synthetic routes.
One-Step Synthesis Focus: Specifically designed for one-step synthesis, it provides concise and direct routes for your target compounds, streamlining the synthesis process.
Accurate Predictions: Utilizing the extensive PISTACHIO, BKMS_METABOLIC, PISTACHIO_RINGBREAKER, REAXYS, REAXYS_BIOCATALYSIS database, our tool offers high-accuracy predictions, reflecting the latest in chemical research and data.
Strategy Settings
Precursor scoring | Relevance Heuristic |
---|---|
Min. plausibility | 0.01 |
Model | Template_relevance |
Template Set | Pistachio/Bkms_metabolic/Pistachio_ringbreaker/Reaxys/Reaxys_biocatalysis |
Top-N result to add to graph | 6 |
Feasible Synthetic Routes
試験管内研究製品の免責事項と情報
BenchChemで提示されるすべての記事および製品情報は、情報提供を目的としています。BenchChemで購入可能な製品は、生体外研究のために特別に設計されています。生体外研究は、ラテン語の "in glass" に由来し、生物体の外で行われる実験を指します。これらの製品は医薬品または薬として分類されておらず、FDAから任何の医療状態、病気、または疾患の予防、治療、または治癒のために承認されていません。これらの製品を人間または動物に体内に導入する形態は、法律により厳格に禁止されています。これらのガイドラインに従うことは、研究と実験において法的および倫理的な基準の遵守を確実にするために重要です。