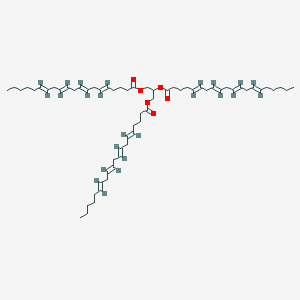
Triarachidonin
説明
Synthesis Analysis
Triarachidonin's synthesis has been explored in the context of prostaglandin formation in rabbit kidney medulla microsomes. Fujimoto et al. (1991) discovered that the addition of triarachidonin stimulates prostaglandin formation in a dose-dependent manner, suggesting its pivotal role in mediating biochemical pathways related to inflammation and kidney function (Fujimoto et al., 1991).
Molecular Structure Analysis
The molecular structure of derivatives formed from arachidonic acid, closely related to Triarachidonin, has been elucidated through studies on lipoxins, which are biologically active compounds. Serhan et al. (1984) identified and analyzed the structure of lipoxins, revealing insights into the complex molecular architecture and biological activity of arachidonic acid derivatives (Serhan et al., 1984).
Chemical Reactions and Properties
Investigations into the chemical reactions of Triarachidonin have shown its significant impact on prostaglandin synthesis, highlighting its chemical properties and reactivity. The study by Fujimoto et al. (1991) demonstrates how Triarachidonin affects the synthesis of various prostaglandins, underlining the compound's chemical versatility and its role in biological processes (Fujimoto et al., 1991).
Physical Properties Analysis
While specific studies focusing directly on the physical properties of Triarachidonin are limited, the physical characteristics of related arachidonic acid derivatives have been extensively studied. These investigations provide a foundational understanding of how similar compounds behave in biological systems, offering indirect insights into the physical properties of Triarachidonin.
Chemical Properties Analysis
The chemical properties of Triarachidonin, particularly its role in stimulating prostaglandin synthesis, have been highlighted in research. This activity is crucial for understanding the compound's biological significance and its potential applications in medical and biochemical research (Fujimoto et al., 1991).
科学的研究の応用
Triarachidonin and Prostaglandin Synthesis in Rabbit Kidney Medulla Microsomes :
- Triarachidonin stimulated the formation of prostaglandins (E2, F2α, D2) in rabbit kidney medulla microsomes in a dose-dependent manner.
- This effect was enhanced by epinephrine, suggesting the presence of epinephrine-activated triacylglycerol lipase, which could mediate arachidonic acid release for prostaglandin synthesis in the kidney medulla (Fujimoto et al., 1991).
Triarachidonin and Renal Medullary Prostaglandin Synthesis :
- Triarachidonin also enhanced prostaglandin E2 production in rabbit kidney medulla slices.
- It was shown that arachidonic acid released from medullary triacylglycerols is readily available for prostaglandin biosynthesis, and triarachidonin's stimulation of prostaglandin E2 was dose-dependent (Fujimoto et al., 1988).
将来の方向性
Triarachidonin, also referred to as TARA, represents an innovative lipid-based therapeutic agent designed to address a range of conditions, encompassing cancer, inflammation, and diabetes . TARA exhibits its efficacy by selectively targeting specific cell signaling pathways, giving rise to a multitude of advantageous outcomes .
作用機序
Target of Action
Triarachidonin is a polyunsaturated triacylglycerol
Biochemical Pathways
Triarachidonin, as a form of triacylglycerol, is involved in lipid metabolism. It can be broken down into glycerol and fatty acids, which can then be used in various biochemical pathways. For instance, the fatty acids can undergo beta-oxidation to produce ATP, the body’s main energy currency .
Result of Action
It’s known that the breakdown products of triacylglycerols, such as glycerol and fatty acids, play crucial roles in energy production and other cellular processes .
特性
IUPAC Name |
2,3-bis[[(5Z,8Z,11Z,14Z)-icosa-5,8,11,14-tetraenoyl]oxy]propyl (5Z,8Z,11Z,14Z)-icosa-5,8,11,14-tetraenoate | |
---|---|---|
Source | PubChem | |
URL | https://pubchem.ncbi.nlm.nih.gov | |
Description | Data deposited in or computed by PubChem | |
InChI |
InChI=1S/C63H98O6/c1-4-7-10-13-16-19-22-25-28-31-34-37-40-43-46-49-52-55-61(64)67-58-60(69-63(66)57-54-51-48-45-42-39-36-33-30-27-24-21-18-15-12-9-6-3)59-68-62(65)56-53-50-47-44-41-38-35-32-29-26-23-20-17-14-11-8-5-2/h16-21,25-30,34-39,43-48,60H,4-15,22-24,31-33,40-42,49-59H2,1-3H3/b19-16-,20-17-,21-18-,28-25-,29-26-,30-27-,37-34-,38-35-,39-36-,46-43-,47-44-,48-45- | |
Source | PubChem | |
URL | https://pubchem.ncbi.nlm.nih.gov | |
Description | Data deposited in or computed by PubChem | |
InChI Key |
HYVJNYYVNIYMDK-QSEXIABDSA-N | |
Source | PubChem | |
URL | https://pubchem.ncbi.nlm.nih.gov | |
Description | Data deposited in or computed by PubChem | |
Canonical SMILES |
CCCCCC=CCC=CCC=CCC=CCCCC(=O)OCC(COC(=O)CCCC=CCC=CCC=CCC=CCCCCC)OC(=O)CCCC=CCC=CCC=CCC=CCCCCC | |
Source | PubChem | |
URL | https://pubchem.ncbi.nlm.nih.gov | |
Description | Data deposited in or computed by PubChem | |
Isomeric SMILES |
CCCCC/C=C\C/C=C\C/C=C\C/C=C\CCCC(=O)OCC(OC(=O)CCC/C=C\C/C=C\C/C=C\C/C=C\CCCCC)COC(=O)CCC/C=C\C/C=C\C/C=C\C/C=C\CCCCC | |
Source | PubChem | |
URL | https://pubchem.ncbi.nlm.nih.gov | |
Description | Data deposited in or computed by PubChem | |
Molecular Formula |
C63H98O6 | |
Source | PubChem | |
URL | https://pubchem.ncbi.nlm.nih.gov | |
Description | Data deposited in or computed by PubChem | |
Molecular Weight |
951.4 g/mol | |
Source | PubChem | |
URL | https://pubchem.ncbi.nlm.nih.gov | |
Description | Data deposited in or computed by PubChem | |
Physical Description |
Solid | |
Record name | TG(20:4(5Z,8Z,11Z,14Z)/20:4(5Z,8Z,11Z,14Z)/20:4(5Z,8Z,11Z,14Z)) | |
Source | Human Metabolome Database (HMDB) | |
URL | http://www.hmdb.ca/metabolites/HMDB0005478 | |
Description | The Human Metabolome Database (HMDB) is a freely available electronic database containing detailed information about small molecule metabolites found in the human body. | |
Explanation | HMDB is offered to the public as a freely available resource. Use and re-distribution of the data, in whole or in part, for commercial purposes requires explicit permission of the authors and explicit acknowledgment of the source material (HMDB) and the original publication (see the HMDB citing page). We ask that users who download significant portions of the database cite the HMDB paper in any resulting publications. | |
Product Name |
Triarachidonin |
Retrosynthesis Analysis
AI-Powered Synthesis Planning: Our tool employs the Template_relevance Pistachio, Template_relevance Bkms_metabolic, Template_relevance Pistachio_ringbreaker, Template_relevance Reaxys, Template_relevance Reaxys_biocatalysis model, leveraging a vast database of chemical reactions to predict feasible synthetic routes.
One-Step Synthesis Focus: Specifically designed for one-step synthesis, it provides concise and direct routes for your target compounds, streamlining the synthesis process.
Accurate Predictions: Utilizing the extensive PISTACHIO, BKMS_METABOLIC, PISTACHIO_RINGBREAKER, REAXYS, REAXYS_BIOCATALYSIS database, our tool offers high-accuracy predictions, reflecting the latest in chemical research and data.
Strategy Settings
Precursor scoring | Relevance Heuristic |
---|---|
Min. plausibility | 0.01 |
Model | Template_relevance |
Template Set | Pistachio/Bkms_metabolic/Pistachio_ringbreaker/Reaxys/Reaxys_biocatalysis |
Top-N result to add to graph | 6 |
Feasible Synthetic Routes
Q & A
Q1: What is the significance of triarachidonin in biological systems?
A1: Triarachidonin is a triglyceride composed of three arachidonic acid molecules. Arachidonic acid is a polyunsaturated omega-6 fatty acid that plays a crucial role in cell signaling and inflammation. [, ] As a precursor to eicosanoids, arachidonic acid derivatives are involved in various physiological processes, including inflammation, immune response, and pain signaling. Triarachidonin serves as a storage form of arachidonic acid, and its hydrolysis can rapidly release this important fatty acid. []
Q2: How does the structure of triarachidonin relate to its potential toxicity?
A2: Triarachidonin’s structure, with its high degree of unsaturation (three arachidonic acid chains each containing four double bonds), makes it highly susceptible to oxidation. [] This oxidation process can generate free radicals and lipid peroxidation products, which are known to be toxic to cells. Studies have shown that triarachidonin exhibits significant toxicity to human monocyte-macrophages, particularly compared to less unsaturated triglycerides. [] This toxicity appears to be directly related to its susceptibility to oxidation and the generation of toxic byproducts.
Q3: Can you elaborate on the dietary requirements and metabolic fate of triarachidonin in mud crabs?
A3: Research on juvenile mud crabs (Scylla serrata) demonstrated that a dietary source of triarachidonin is essential for their growth. [] When deprived of dietary triarachidonin, the levels of arachidonic acid in the crabs’ neutral lipids decreased, while levels in polar lipids were conserved, suggesting a selective retention mechanism. [] This highlights the critical role of triarachidonin in providing the arachidonic acid necessary for the growth and development of these organisms.
Q4: Are there any alternative methods to traditional techniques for analyzing triarachidonin in biological samples?
A4: Yes, Direct Thermal Desorption (DTD) combined with gas chromatography offers a promising alternative to conventional methods for analyzing triarachidonin and other fatty acids in complex matrices like microbial cells. [] This method, when combined with Thermally assisted Hydrolysis and Methylation (THM), can efficiently convert triglycerides like triarachidonin into their corresponding fatty acid methyl esters (FAMEs), which can then be analyzed via GC. [] DTD-GC offers several advantages over traditional methods, including reduced sample preparation, minimized risk of isomerization, and the potential for automation for high-throughput analysis. []
Q5: How does triarachidonin contribute to our understanding of cancer cachexia?
A5: Studies using a mouse model of cancer cachexia have linked triarachidonin to the muscle wasting characteristic of the disease. [] Researchers observed that the action of a proteolysis-inducing factor found in cachectic animals could be mimicked by triarachidonin. [] This finding suggests that elevated arachidonic acid levels, potentially derived from triarachidonin, could contribute to the increased muscle protein degradation observed in cancer cachexia. Further research into this mechanism might lead to novel therapeutic approaches for managing this debilitating condition.
試験管内研究製品の免責事項と情報
BenchChemで提示されるすべての記事および製品情報は、情報提供を目的としています。BenchChemで購入可能な製品は、生体外研究のために特別に設計されています。生体外研究は、ラテン語の "in glass" に由来し、生物体の外で行われる実験を指します。これらの製品は医薬品または薬として分類されておらず、FDAから任何の医療状態、病気、または疾患の予防、治療、または治癒のために承認されていません。これらの製品を人間または動物に体内に導入する形態は、法律により厳格に禁止されています。これらのガイドラインに従うことは、研究と実験において法的および倫理的な基準の遵守を確実にするために重要です。