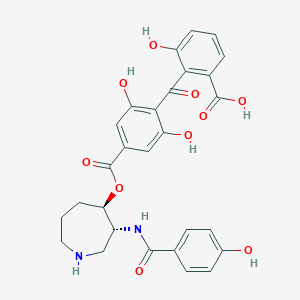
Balanol
説明
Balanol is a fungal metabolite produced by the fungus Verticillium balanoides . It is a potent inhibitor of the serine/threonine kinases protein kinase A (PKA) and protein kinase C (PKC), binding in a similar manner with that of ATP . Balanol was discovered in 1993 in the search for novel inhibitors of PKC, a member of a family of serine/threonine kinases whose overactivation is associated with numerous human diseases of signal transduction including cancer .
Synthesis Analysis
The total synthesis of Balanol has been achieved through novel synthetic routes . The hexahydroazepine fragment was prepared in racemic form through either Bu3SnH- or SmI2-promoted radical cyclization of oxime ethers . The benzophenone fragment was prepared in short steps through a biomimetic oxidative anthraquinone ring cleavage starting from commercially available natural chrysophanic acid .
Molecular Structure Analysis
The Balanol molecule consists of three regions: a benzophenone, hexahydroazepane, and 4-hydroxy benzoyl moiety . The benzophenone and hexahydroazepane moieties are connected via an ester linkage, and the azepane and benzoyl moieties are connected through an amide linkage . Balanol is a congener of ATP, with distinct regions of its molecular structure able to form bonds like the adenine ring, ribose, and phosphate groups of the ATP molecule .
Chemical Reactions Analysis
The chemical reactions involved in the synthesis of Balanol have been studied extensively . For example, modifications of the benzophenone rings, analogs of the phosphate groups of ATP, produce unusually potent and specific protein kinase inhibitors .
Physical And Chemical Properties Analysis
Balanol has a chemical formula of C28H26N2O10 and a molecular weight of 550.51 . It is a small molecule and belongs to the class of organic compounds known as benzophenones .
科学的研究の応用
Protein Kinase C Inhibitor
Balanol is a potential protein kinase C (PKC) inhibitor . PKC is a family of protein kinase enzymes that are involved in controlling the function of other proteins through the phosphorylation of hydroxyl groups of serine and threonine amino acid residues. As an inhibitor, Balanol can prevent the overactivity of PKC, which is often associated with diseases like cancer .
ATP-Competitive Inhibitor
Balanol acts as an ATP-competitive inhibitor . This means it competes with ATP (adenosine triphosphate) for the same binding site on a target protein. This property is particularly useful in the development of drugs that target kinases, as these enzymes play key roles in cellular signal transduction.
Anticancer Applications
Balanol has shown potential in anticancer applications. It has been found to inhibit PKC isozymes, which act as tumor promoters or suppressors, depending on the cancer type . In particular, PKCε is frequently implicated in cancer promotion, making it a potential target for anticancer drugs .
Biosynthetic Pathway Regulation
Research has revealed the biosynthetic pathway of Balanol through the overexpression of the cluster-situated regulator gene blnR in the Chinese herb fungus Tolypocladium ophioglossoides . Understanding this pathway could lead to more efficient production of Balanol for therapeutic applications.
Fungal Metabolite Production
Balanol is a metabolite produced by certain fungi, including Verticillium balanoides and Tolypocladium ophioglossoides . The production of Balanol in these fungi can be optimized through medium optimization and regulatory pathway manipulation .
Charge State Exploration for Drug Design
The charge states of Balanol analogues have been explored for the design of highly selective inhibitors for therapeutic applications . Understanding the effect of fluorine substitutions on the exact charge state of each Balanol analogue bound to PKA and to PKCε is crucial for designing highly selective inhibitors .
作用機序
Balanol is a fungal metabolite produced by the fungus Verticillium balanoides . It has gained significant attention due to its potential as a protein kinase C inhibitor . This article will delve into the mechanism of action of Balanol, covering its primary targets, mode of action, affected biochemical pathways, pharmacokinetics, molecular and cellular effects, and the influence of environmental factors on its action.
Target of Action
Balanol primarily targets the cAMP-dependent protein kinase catalytic subunit alpha . It also acts as a non-selective ATP-competitive inhibitor of protein kinases A, G, and C (AGC family kinases) and G protein-coupled receptor kinases (GRKs), with modest selectivity for the GRK2 family members, GRK2 and GRK3 .
Mode of Action
Balanol binds in a similar manner to ATP, acting as a potent inhibitor of the serine/threonine kinases protein kinase A (PKA) and protein kinase C (PKC) .
Biochemical Pathways
The biosynthetic pathway of Balanol has been revealed through overexpression of the cluster-situated regulator gene blnR in the Chinese herb fungus Tolypocladium ophioglossoides . BlnR positively regulates Balanol biosynthesis through binding to all promoters of bln gene members, including its own promoter .
Result of Action
The molecular and cellular effects of Balanol’s action primarily involve the inhibition of PKA and PKC. This inhibition is linked to the control of cell growth and differentiation, and the dysregulation of these kinases is associated with numerous human diseases of signal transduction, including cancer .
Action Environment
It’s worth noting that the production of balanol can be optimized through statistical optimization based on response surface methodology .
将来の方向性
特性
IUPAC Name |
2-[2,6-dihydroxy-4-[(3R,4R)-3-[(4-hydroxybenzoyl)amino]azepan-4-yl]oxycarbonylbenzoyl]-3-hydroxybenzoic acid | |
---|---|---|
Source | PubChem | |
URL | https://pubchem.ncbi.nlm.nih.gov | |
Description | Data deposited in or computed by PubChem | |
InChI |
InChI=1S/C28H26N2O10/c31-16-8-6-14(7-9-16)26(36)30-18-13-29-10-2-5-22(18)40-28(39)15-11-20(33)24(21(34)12-15)25(35)23-17(27(37)38)3-1-4-19(23)32/h1,3-4,6-9,11-12,18,22,29,31-34H,2,5,10,13H2,(H,30,36)(H,37,38)/t18-,22-/m1/s1 | |
Source | PubChem | |
URL | https://pubchem.ncbi.nlm.nih.gov | |
Description | Data deposited in or computed by PubChem | |
InChI Key |
XYUFCXJZFZPEJD-XMSQKQJNSA-N | |
Source | PubChem | |
URL | https://pubchem.ncbi.nlm.nih.gov | |
Description | Data deposited in or computed by PubChem | |
Canonical SMILES |
C1CC(C(CNC1)NC(=O)C2=CC=C(C=C2)O)OC(=O)C3=CC(=C(C(=C3)O)C(=O)C4=C(C=CC=C4O)C(=O)O)O | |
Source | PubChem | |
URL | https://pubchem.ncbi.nlm.nih.gov | |
Description | Data deposited in or computed by PubChem | |
Isomeric SMILES |
C1C[C@H]([C@@H](CNC1)NC(=O)C2=CC=C(C=C2)O)OC(=O)C3=CC(=C(C(=C3)O)C(=O)C4=C(C=CC=C4O)C(=O)O)O | |
Source | PubChem | |
URL | https://pubchem.ncbi.nlm.nih.gov | |
Description | Data deposited in or computed by PubChem | |
Molecular Formula |
C28H26N2O10 | |
Source | PubChem | |
URL | https://pubchem.ncbi.nlm.nih.gov | |
Description | Data deposited in or computed by PubChem | |
DSSTOX Substance ID |
DTXSID201318143 | |
Record name | (-)-Balanol | |
Source | EPA DSSTox | |
URL | https://comptox.epa.gov/dashboard/DTXSID201318143 | |
Description | DSSTox provides a high quality public chemistry resource for supporting improved predictive toxicology. | |
Molecular Weight |
550.5 g/mol | |
Source | PubChem | |
URL | https://pubchem.ncbi.nlm.nih.gov | |
Description | Data deposited in or computed by PubChem | |
Product Name |
Balanol | |
CAS RN |
63590-19-2 | |
Record name | (-)-Balanol | |
Source | CAS Common Chemistry | |
URL | https://commonchemistry.cas.org/detail?cas_rn=63590-19-2 | |
Description | CAS Common Chemistry is an open community resource for accessing chemical information. Nearly 500,000 chemical substances from CAS REGISTRY cover areas of community interest, including common and frequently regulated chemicals, and those relevant to high school and undergraduate chemistry classes. This chemical information, curated by our expert scientists, is provided in alignment with our mission as a division of the American Chemical Society. | |
Explanation | The data from CAS Common Chemistry is provided under a CC-BY-NC 4.0 license, unless otherwise stated. | |
Record name | (-)-Balanol | |
Source | EPA DSSTox | |
URL | https://comptox.epa.gov/dashboard/DTXSID201318143 | |
Description | DSSTox provides a high quality public chemistry resource for supporting improved predictive toxicology. | |
Record name | BALANOL | |
Source | FDA Global Substance Registration System (GSRS) | |
URL | https://gsrs.ncats.nih.gov/ginas/app/beta/substances/O0N0E1MP23 | |
Description | The FDA Global Substance Registration System (GSRS) enables the efficient and accurate exchange of information on what substances are in regulated products. Instead of relying on names, which vary across regulatory domains, countries, and regions, the GSRS knowledge base makes it possible for substances to be defined by standardized, scientific descriptions. | |
Explanation | Unless otherwise noted, the contents of the FDA website (www.fda.gov), both text and graphics, are not copyrighted. They are in the public domain and may be republished, reprinted and otherwise used freely by anyone without the need to obtain permission from FDA. Credit to the U.S. Food and Drug Administration as the source is appreciated but not required. | |
Retrosynthesis Analysis
AI-Powered Synthesis Planning: Our tool employs the Template_relevance Pistachio, Template_relevance Bkms_metabolic, Template_relevance Pistachio_ringbreaker, Template_relevance Reaxys, Template_relevance Reaxys_biocatalysis model, leveraging a vast database of chemical reactions to predict feasible synthetic routes.
One-Step Synthesis Focus: Specifically designed for one-step synthesis, it provides concise and direct routes for your target compounds, streamlining the synthesis process.
Accurate Predictions: Utilizing the extensive PISTACHIO, BKMS_METABOLIC, PISTACHIO_RINGBREAKER, REAXYS, REAXYS_BIOCATALYSIS database, our tool offers high-accuracy predictions, reflecting the latest in chemical research and data.
Strategy Settings
Precursor scoring | Relevance Heuristic |
---|---|
Min. plausibility | 0.01 |
Model | Template_relevance |
Template Set | Pistachio/Bkms_metabolic/Pistachio_ringbreaker/Reaxys/Reaxys_biocatalysis |
Top-N result to add to graph | 6 |
Feasible Synthetic Routes
試験管内研究製品の免責事項と情報
BenchChemで提示されるすべての記事および製品情報は、情報提供を目的としています。BenchChemで購入可能な製品は、生体外研究のために特別に設計されています。生体外研究は、ラテン語の "in glass" に由来し、生物体の外で行われる実験を指します。これらの製品は医薬品または薬として分類されておらず、FDAから任何の医療状態、病気、または疾患の予防、治療、または治癒のために承認されていません。これらの製品を人間または動物に体内に導入する形態は、法律により厳格に禁止されています。これらのガイドラインに従うことは、研究と実験において法的および倫理的な基準の遵守を確実にするために重要です。