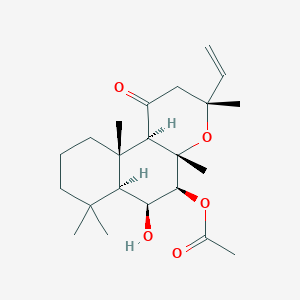
1,9-Dideoxyforskolin
概要
説明
準備方法
合成経路と反応条件
1,9-ジデオキシフォルスコリンは、フォルスコリンから1位と9位のヒドロキシル基を除去することで合成できます。特定の合成経路と反応条件は、文献では広く報告されていません。 フォルスコリンアナログは、さまざまなヒドロキシル基で修飾して、さまざまな誘導体を生成することが知られています .
工業生産方法
1,9-ジデオキシフォルスコリンの工業生産方法に関する情報は限られています。 通常、大規模な工業生産ではなく、研究目的のために少量生産されます .
化学反応の分析
反応の種類
1,9-ジデオキシフォルスコリンは、以下を含むさまざまな化学反応を起こします。
酸化: この化合物は、酸化されてさまざまな誘導体を形成することができます。
還元: 還元反応は、化合物の官能基を修飾できます。
一般的な試薬と条件
1,9-ジデオキシフォルスコリンの反応で一般的に使用される試薬には、酸化剤、還元剤、さまざまな触媒などがあります。 特定の条件は、目的の反応と目的の生成物によって異なります .
生成される主な生成物
1,9-ジデオキシフォルスコリンの反応から生成される主な生成物には、官能基が修飾されたさまざまなフォルスコリン誘導体があります。 これらの誘導体は、しばしばフォルスコリンとそのアナログの生物活性を研究するために研究で使用されます .
4. 科学研究での応用
1,9-ジデオキシフォルスコリンは、以下を含むさまざまな科学研究に応用されています。
科学的研究の応用
1,9-Dideoxyforskolin is used in a variety of scientific research applications, including:
作用機序
1,9-ジデオキシフォルスコリンは、フォルスコリンとは異なり、アデニル酸シクラーゼを刺激しません . それは生物学的に不活性なアナログとして作用するため、実験でネガティブコントロールとして役立ちます。 この化合物の作用機序には、酵素を活性化せずにフォルスコリン結合部位との相互作用が含まれます . これにより、研究者は、アデニル酸シクラーゼ活性化の混雑する影響なしに、フォルスコリンの影響を研究することができます .
6. 類似化合物の比較
類似化合物
フォルスコリン: 親化合物であり、アデニル酸シクラーゼの活性化剤としての役割で知られています.
イソフォルスコリン: 同様の性質を持つ別のフォルスコリンアナログ.
デオキシニバレノール: 構造的に関連する化合物であり、同様の研究コンテキストで使用されます.
1,9-ジデオキシフォルスコリンの独自性
1,9-ジデオキシフォルスコリンは、アデニル酸シクラーゼの刺激における生物学的活性が欠如しているため、独特です。 これは、フォルスコリン関連の研究で理想的なネガティブコントロールとなり、科学者はアデニル酸シクラーゼの活性化なしに、フォルスコリンの特定の影響を分離することができます .
類似化合物との比較
Similar Compounds
Forskolin: The parent compound, known for its role as an activator of adenylyl cyclase.
Isoforskolin: Another forskolin analog with similar properties.
Deoxynivalenol: A structurally related compound used in similar research contexts.
Uniqueness of 1,9-Dideoxyforskolin
This compound is unique due to its lack of biological activity in stimulating adenylyl cyclase. This makes it an ideal negative control in forskolin-related research, allowing scientists to isolate the specific effects of forskolin without the activation of adenylyl cyclase .
生物活性
1,9-Dideoxyforskolin is a structural analogue of forskolin, a compound known for its ability to activate adenylate cyclase, thereby increasing cyclic AMP (cAMP) levels in cells. Unlike forskolin, this compound does not stimulate adenylate cyclase but exhibits significant biological activities, particularly as an inhibitor of cyclic AMP phosphodiesterase and modulator of calcium channels.
This compound primarily acts through the inhibition of cyclic AMP phosphodiesterase (PDE), making it a more potent inhibitor compared to forskolin. This inhibition leads to increased levels of cAMP within cells, which can have various downstream effects on cellular signaling pathways. Furthermore, it has been shown to stimulate protein kinase C (PKC) activity in renal epithelial cells, suggesting a role in modulating various signaling pathways independent of cAMP accumulation .
Table 1: Comparison of Biological Activities
Activity Type | Forskolin | This compound |
---|---|---|
Adenylate Cyclase Activation | Yes | No |
Inhibition of PDE | Less potent | More potent |
Calcium Channel Modulation | Minimal effect | Significant inhibition |
Protein Kinase C Stimulation | Yes | Yes |
Effects on Calcium Channels
Research indicates that this compound significantly inhibits voltage-activated calcium currents in rat cerebellar granule cells. In experiments using patch-clamp techniques, it was found that at a concentration of 100 µM, this compound inhibited these currents by approximately 63%, while forskolin showed no significant effect under the same conditions . This suggests that this compound may have potential therapeutic applications in conditions where modulation of calcium signaling is beneficial.
Neuroplasticity and GABA Receptor Interaction
This compound has also been implicated in the modulation of GABA receptor function. In studies involving synaptoneurosomes from rat cerebral cortex, it was observed that this compound inhibited muscimol-induced chloride uptake in a concentration-dependent manner. This effect is notable because it occurs independently of cAMP activation, indicating a direct interaction with GABA receptors . The IC50 for this inhibitory effect was reported to be around 1.3 mM.
Case Studies and Research Findings
Several studies have highlighted the potential applications of this compound in neuropharmacology and cellular signaling:
- Neuroprotection : In vitro studies suggest that the compound may protect against excitotoxicity in neuronal cultures by modulating intracellular calcium levels.
- Mood Stabilizers : Investigations into mood stabilizers revealed that treatments affecting neuroplasticity also influence the phosphorylation state of proteins involved in synaptic function. The role of this compound in such contexts warrants further exploration .
特性
IUPAC Name |
[(3R,4aS,5S,6S,6aS,10aS,10bR)-3-ethenyl-6-hydroxy-3,4a,7,7,10a-pentamethyl-1-oxo-2,5,6,6a,8,9,10,10b-octahydrobenzo[f]chromen-5-yl] acetate | |
---|---|---|
Source | PubChem | |
URL | https://pubchem.ncbi.nlm.nih.gov | |
Description | Data deposited in or computed by PubChem | |
InChI |
InChI=1S/C22H34O5/c1-8-20(5)12-14(24)16-21(6)11-9-10-19(3,4)17(21)15(25)18(26-13(2)23)22(16,7)27-20/h8,15-18,25H,1,9-12H2,2-7H3/t15-,16+,17-,18-,20-,21+,22-/m0/s1 | |
Source | PubChem | |
URL | https://pubchem.ncbi.nlm.nih.gov | |
Description | Data deposited in or computed by PubChem | |
InChI Key |
ZKZMDXUDDJYAIB-SUCLLAFCSA-N | |
Source | PubChem | |
URL | https://pubchem.ncbi.nlm.nih.gov | |
Description | Data deposited in or computed by PubChem | |
Canonical SMILES |
CC(=O)OC1C(C2C(CCCC2(C3C1(OC(CC3=O)(C)C=C)C)C)(C)C)O | |
Source | PubChem | |
URL | https://pubchem.ncbi.nlm.nih.gov | |
Description | Data deposited in or computed by PubChem | |
Isomeric SMILES |
CC(=O)O[C@H]1[C@H]([C@@H]2[C@](CCCC2(C)C)([C@@H]3[C@@]1(O[C@@](CC3=O)(C)C=C)C)C)O | |
Source | PubChem | |
URL | https://pubchem.ncbi.nlm.nih.gov | |
Description | Data deposited in or computed by PubChem | |
Molecular Formula |
C22H34O5 | |
Source | PubChem | |
URL | https://pubchem.ncbi.nlm.nih.gov | |
Description | Data deposited in or computed by PubChem | |
DSSTOX Substance ID |
DTXSID5040384 | |
Record name | 1,9-Dideoxyforskolin | |
Source | EPA DSSTox | |
URL | https://comptox.epa.gov/dashboard/DTXSID5040384 | |
Description | DSSTox provides a high quality public chemistry resource for supporting improved predictive toxicology. | |
Molecular Weight |
378.5 g/mol | |
Source | PubChem | |
URL | https://pubchem.ncbi.nlm.nih.gov | |
Description | Data deposited in or computed by PubChem | |
CAS No. |
64657-18-7 | |
Record name | (-)-1,9-Dideoxyforskolin | |
Source | CAS Common Chemistry | |
URL | https://commonchemistry.cas.org/detail?cas_rn=64657-18-7 | |
Description | CAS Common Chemistry is an open community resource for accessing chemical information. Nearly 500,000 chemical substances from CAS REGISTRY cover areas of community interest, including common and frequently regulated chemicals, and those relevant to high school and undergraduate chemistry classes. This chemical information, curated by our expert scientists, is provided in alignment with our mission as a division of the American Chemical Society. | |
Explanation | The data from CAS Common Chemistry is provided under a CC-BY-NC 4.0 license, unless otherwise stated. | |
Record name | 1,9-Dideoxyforskolin | |
Source | ChemIDplus | |
URL | https://pubchem.ncbi.nlm.nih.gov/substance/?source=chemidplus&sourceid=0064657187 | |
Description | ChemIDplus is a free, web search system that provides access to the structure and nomenclature authority files used for the identification of chemical substances cited in National Library of Medicine (NLM) databases, including the TOXNET system. | |
Record name | 1,9-Dideoxyforskolin | |
Source | EPA DSSTox | |
URL | https://comptox.epa.gov/dashboard/DTXSID5040384 | |
Description | DSSTox provides a high quality public chemistry resource for supporting improved predictive toxicology. | |
Record name | (3R,4aR,5S,6S,6aS,10S,10aR,10bS)-dodecahydro-5,6,10,10b-tetrahydroxy-3,4a,7,7,10a-pentamethyl-1-oxo-3-vinyl-1H-naphtho[2,1-b]pyran-5-yl acetat | |
Source | European Chemicals Agency (ECHA) | |
URL | https://echa.europa.eu/information-on-chemicals | |
Description | The European Chemicals Agency (ECHA) is an agency of the European Union which is the driving force among regulatory authorities in implementing the EU's groundbreaking chemicals legislation for the benefit of human health and the environment as well as for innovation and competitiveness. | |
Explanation | Use of the information, documents and data from the ECHA website is subject to the terms and conditions of this Legal Notice, and subject to other binding limitations provided for under applicable law, the information, documents and data made available on the ECHA website may be reproduced, distributed and/or used, totally or in part, for non-commercial purposes provided that ECHA is acknowledged as the source: "Source: European Chemicals Agency, http://echa.europa.eu/". Such acknowledgement must be included in each copy of the material. ECHA permits and encourages organisations and individuals to create links to the ECHA website under the following cumulative conditions: Links can only be made to webpages that provide a link to the Legal Notice page. | |
Record name | 1,9-DIDEOXYFORSKOLIN | |
Source | FDA Global Substance Registration System (GSRS) | |
URL | https://gsrs.ncats.nih.gov/ginas/app/beta/substances/OAW710HWIX | |
Description | The FDA Global Substance Registration System (GSRS) enables the efficient and accurate exchange of information on what substances are in regulated products. Instead of relying on names, which vary across regulatory domains, countries, and regions, the GSRS knowledge base makes it possible for substances to be defined by standardized, scientific descriptions. | |
Explanation | Unless otherwise noted, the contents of the FDA website (www.fda.gov), both text and graphics, are not copyrighted. They are in the public domain and may be republished, reprinted and otherwise used freely by anyone without the need to obtain permission from FDA. Credit to the U.S. Food and Drug Administration as the source is appreciated but not required. | |
Retrosynthesis Analysis
AI-Powered Synthesis Planning: Our tool employs the Template_relevance Pistachio, Template_relevance Bkms_metabolic, Template_relevance Pistachio_ringbreaker, Template_relevance Reaxys, Template_relevance Reaxys_biocatalysis model, leveraging a vast database of chemical reactions to predict feasible synthetic routes.
One-Step Synthesis Focus: Specifically designed for one-step synthesis, it provides concise and direct routes for your target compounds, streamlining the synthesis process.
Accurate Predictions: Utilizing the extensive PISTACHIO, BKMS_METABOLIC, PISTACHIO_RINGBREAKER, REAXYS, REAXYS_BIOCATALYSIS database, our tool offers high-accuracy predictions, reflecting the latest in chemical research and data.
Strategy Settings
Precursor scoring | Relevance Heuristic |
---|---|
Min. plausibility | 0.01 |
Model | Template_relevance |
Template Set | Pistachio/Bkms_metabolic/Pistachio_ringbreaker/Reaxys/Reaxys_biocatalysis |
Top-N result to add to graph | 6 |
Feasible Synthetic Routes
- Glucose Transporter Inhibition: 1,9-DDF potently inhibits glucose transport and cytochalasin B binding in rat adipocyte membranes, suggesting a direct interaction with glucose transporters []. This inhibition can lead to a reduction in testosterone production due to the accumulation of androstenedione, highlighting the essential role of glucose uptake in this metabolic step [, ].
- Potassium Channel Modulation: 1,9-DDF appears to interact with potassium channels, particularly calcium-dependent potassium channels, contributing to the inhibition of uterine contractions in rats []. It also inhibits volume-activated chloride currents in HeLa and KB3 cells, although the exact mechanism remains unclear [].
- Calcium Channel Blocker-like Action: 1,9-DDF exhibits calcium channel blocker-like effects, inhibiting vascular smooth muscle contraction by reducing cytosolic calcium levels [].
- P-Glycoprotein Interaction: Research suggests 1,9-DDF binds to P-glycoprotein, a multidrug transporter, potentially explaining its ability to enhance the cytotoxic effects of adriamycin in multidrug-resistant ovarian carcinoma cells [].
A:
ANone: There's limited information available regarding the material compatibility and stability of 1,9-DDF under various conditions. Further studies are needed to explore its performance and potential applications.
ANone: 1,9-DDF is not known to possess catalytic properties. It primarily acts by interacting with specific protein targets rather than catalyzing chemical reactions.
A: While computational studies specifically on 1,9-DDF are limited in the provided research, QSAR models for forskolin and its analogs, including 1,9-DDF, have been developed to understand the relationship between their structure and activity [].
ANone: The structure of 1,9-DDF plays a crucial role in its activity and interactions:
- Adenylate Cyclase Inactivity: The absence of hydroxyl groups at positions 1 and 9 in 1,9-DDF renders it incapable of activating adenylate cyclase, unlike forskolin [, ].
- Glucose Transport Inhibition: The structural features responsible for 1,9-DDF's potent inhibition of glucose transport are distinct from those required for adenylate cyclase activation [].
- P-Glycoprotein Binding: 1,9-DDF exhibits higher affinity for P-glycoprotein compared to forskolin, indicating that modifications at positions 1 and 9 contribute to this interaction [].
ANone: Detailed information regarding the stability of 1,9-DDF under various conditions and specific formulation strategies is not extensively discussed in the provided research.
ANone: Information about SHE regulations specific to 1,9-DDF is not available in the provided research articles.
ANone: Specific details about the absorption, distribution, metabolism, and excretion of 1,9-DDF are not elaborated in the research provided. Further investigation is needed to determine its in vivo activity and efficacy profile.
ANone: 1,9-DDF has shown efficacy in various in vitro and ex vivo models:
- Rat Adipocytes: Inhibits glucose transport and cytochalasin B binding [].
- Rat Uterus: Reduces contractility, potentially by modulating potassium channels [].
- Rat Aorta: Inhibits vascular smooth muscle contraction, exhibiting calcium channel blocker-like effects [].
- Ovarian Carcinoma Cells: Enhances the cytotoxic effects of adriamycin in multidrug-resistant cell lines, likely through P-glycoprotein interaction [].
A: While the provided research doesn't directly address 1,9-DDF resistance, its interaction with P-glycoprotein [] suggests a potential role in overcoming multidrug resistance in cancer cells.
ANone: Comprehensive data on the toxicology and long-term safety profile of 1,9-DDF is not included in the provided research.
A: 1,9-DDF research emerged alongside the investigation of forskolin, a natural product known to activate adenylate cyclase. Scientists synthesized 1,9-DDF to dissect the different pharmacological effects of forskolin, particularly those independent of cAMP elevation [, , ].
試験管内研究製品の免責事項と情報
BenchChemで提示されるすべての記事および製品情報は、情報提供を目的としています。BenchChemで購入可能な製品は、生体外研究のために特別に設計されています。生体外研究は、ラテン語の "in glass" に由来し、生物体の外で行われる実験を指します。これらの製品は医薬品または薬として分類されておらず、FDAから任何の医療状態、病気、または疾患の予防、治療、または治癒のために承認されていません。これらの製品を人間または動物に体内に導入する形態は、法律により厳格に禁止されています。これらのガイドラインに従うことは、研究と実験において法的および倫理的な基準の遵守を確実にするために重要です。