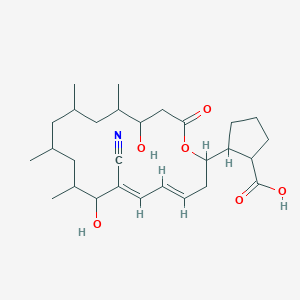
Borrelidin
概要
説明
Borrelidin is an 18-membered nitrile-containing macrolide antibiotic first isolated from Streptomyces rochei in 1949 . It exhibits diverse biological activities, including antibacterial, antifungal, antimalarial, and anticancer properties. Its primary mechanism of action involves noncompetitive inhibition of threonyl-tRNA synthetase (ThrRS), a critical enzyme in protein synthesis . By binding to ThrRS, this compound disrupts aminoacylation of tRNA, leading to amino acid starvation, activation of stress-response pathways like the unfolded protein response (UPR), and apoptosis in cancer cells .
This compound also demonstrates unique off-target effects, such as dissociating amyloid-β (Aβ) and tau fibrils in Alzheimer’s disease models and inhibiting tumor cell migration . Its production can be enhanced through Streptomyces-fungus co-culture, which increases yields of this compound and its derivatives by over fourfold .
準備方法
Synthetic Routes and Reaction Conditions: Borrelidin is biosynthesized by type I polyketide synthase (PKS), followed by post-PKS modifications. The core structure is formed through a series of extension modules involving ketosynthase, acyl transferase, ketoreductase, dehydratase, and enoyl reductase enzymes . The polyketide intermediate undergoes cyclization by thioesterase to release the final product .
Industrial Production Methods: Industrial production of this compound involves the cultivation of Streptomyces rochei strains, followed by isolation and purification using solid-phase extraction and high-performance liquid chromatography . This method ensures the production of this compound with high purity and yield.
化学反応の分析
Types of Reactions: Borrelidin undergoes various chemical reactions, including oxidation, reduction, and substitution. It is known for its ability to inhibit protein synthesis by blocking threonyl-tRNA synthetase .
Common Reagents and Conditions: Common reagents used in this compound reactions include malonyl-CoA, methylmalonyl-CoA, and various enzymes such as ketoreductase and dehydratase . The reactions typically occur under mild conditions, ensuring the stability of the compound.
Major Products Formed: The major products formed from this compound reactions include its analogs and derivatives, which are studied for their enhanced biological activities .
科学的研究の応用
Antibacterial Properties
Borrelidin exhibits potent antibacterial activity, particularly against antibiotic-resistant strains such as methicillin-resistant Staphylococcus aureus (MRSA). Research indicates that it functions by inhibiting threonyl-tRNA synthetase, an essential enzyme in protein synthesis. This inhibition disrupts the translation process in bacteria, effectively halting their growth and proliferation .
Table 1: Antibacterial Efficacy of this compound Against Resistant Strains
Bacterial Strain | Minimum Inhibitory Concentration (MIC) |
---|---|
Methicillin-resistant Staphylococcus aureus | 0.5 µg/mL |
Escherichia coli | 1 µg/mL |
Pseudomonas aeruginosa | 2 µg/mL |
Antifungal Activity
In addition to its antibacterial properties, this compound has demonstrated antifungal activity. It is effective against various fungal pathogens, making it a candidate for treating fungal infections. The compound's mechanism involves similar pathways as those observed in bacterial inhibition, suggesting a broad-spectrum application against both bacteria and fungi .
Antimalarial Effects
This compound has also been investigated for its antimalarial properties. Studies indicate that it can inhibit the growth of Plasmodium falciparum, the parasite responsible for malaria, by disrupting protein synthesis within the parasite cells . This discovery positions this compound as a potential lead compound for developing new antimalarial drugs.
Anticancer Potential
Recent research highlights this compound's anticancer properties, particularly in its ability to dissociate amyloid-beta (Aβ) aggregates and regulate tau pathology, which are critical factors in neurodegenerative diseases like Alzheimer's . Its cytotoxic effects on cancer cell lines have prompted further investigation into its mechanisms and potential applications in oncology.
Table 2: Summary of this compound's Biological Activities
Implications for Drug Discovery
The insights gained from studying this compound's mechanisms have significant implications for drug discovery. As researchers explore tRNA synthetase inhibitors further, this compound serves as a model compound for designing more selective and effective drugs against bacterial infections, cancers, and other diseases .
Case Studies and Future Directions
Recent studies have employed genome mining techniques to identify additional biosynthetic pathways for compounds similar to this compound in other Streptomyces species. This approach not only enhances the understanding of this compound's biosynthesis but also aids in discovering novel compounds with similar or enhanced biological activities .
Case Study: Genome Mining for this compound Analogues
作用機序
Borrelidin exerts its effects by inhibiting threonyl-tRNA synthetase, preventing the amino acid L-threonine from linking to its tRNA. This inhibition occurs at the enzyme’s active site and involves the energy molecule ATP . Additionally, this compound impedes angiogenesis and induces apoptosis in certain types of leukemia .
類似化合物との比較
Borrelidin belongs to a family of macrolactone polyketides with structural analogs that exhibit varied biological activities. Below is a detailed comparison:
Structural Analogs and Modifications
Table 1: Key this compound Derivatives and Their Structural Features
Key Structural-Activity Relationships (SAR):
- The nitrile group at C1 is critical for ThrRS binding and bioactivity. Substitution with bulkier groups (e.g., N-methylacetylamide) reduces UPR activation and antiproliferative effects .
- Hydroxyl group placement (e.g., at C7 or C20) influences selectivity. This compound H (148), with a cis C14–C15 olefin and α-OH, shows enhanced selectivity for cancer cells over nonmalignant cells .
Table 2: Comparative Bioactivity Profiles
Key Findings:
- Antifungal Potency: this compound A is 62.5-fold more potent than metalaxyl against Phytophthora sojae (EC₅₀ = 0.0056 mg/L vs. 0.35 mg/L) . It also inhibits Phytophthora infestans and Botrytis cinerea, expanding its antifungal spectrum .
- Anticancer Selectivity: this compound H (148) shows low micromolar cytotoxicity (IC₅₀ = 0.12–2.19 µM) against A549, HeLa, and HepG2 cells, with minimal effects on nonmalignant Huvec-12 cells . This selectivity is attributed to its cis-olefin geometry and α-OH configuration.
- Neuroprotective Effects: this compound C dissociates preformed Aβ and tau aggregates, reducing neurotoxicity in Alzheimer’s models .
Mechanistic Divergence from Other Macrolides
While this compound targets ThrRS, other macrolides like macrolones (e.g., erythromycin derivatives) inhibit bacterial ribosomes and DNA gyrase . Additionally, this compound’s antifungal action is independent of ergosterol biosynthesis (a common target for azoles), making it effective against resistant strains .
Toxicity and Therapeutic Window
- In Vivo Safety: this compound at 0.25 mg/kg/day cured malaria-infected mice without toxicity, suggesting a favorable therapeutic window .
- Cellular Toxicity: Normal human keratinocytes (nHEK) and cancer cells show similar IC₅₀ values in vitro, but structural analogs like this compound H mitigate toxicity in nonmalignant cells .
生物活性
Borrelidin is a naturally occurring macrolide antibiotic produced by Streptomyces rochei, known for its diverse biological activities, particularly its potent antimalarial, anticancer, and antibacterial properties. This article delves into the biological activity of this compound, focusing on its mechanisms of action, efficacy in various biological systems, and potential therapeutic applications.
The primary mechanism through which this compound exerts its biological effects is the inhibition of threonyl-tRNA synthetase (ThrRS), an essential enzyme in protein synthesis. By binding to ThrRS, this compound disrupts protein synthesis in both prokaryotic and eukaryotic cells .
Key Mechanisms:
- Inhibition of Protein Synthesis : this compound acts as a competitive inhibitor of ThrRS, leading to impaired translation processes in cells. This inhibition has been shown to result in apoptosis in various cell types, including endothelial cells and acute lymphoblastic leukemia cells .
- Induction of the Unfolded Protein Response (UPR) : this compound activates UPR pathways, which are cellular stress responses triggered by the accumulation of misfolded proteins. This activation is associated with increased phosphorylation of eIF2α and splicing of XBP1 mRNA, leading to cell death in cancerous cells .
Antimalarial Activity
This compound has demonstrated significant antimalarial activity against Plasmodium species, particularly P. falciparum and P. berghei. Research indicates that this compound exhibits a stage-specific inhibitory effect on malaria parasites, particularly during the trophozoite stage.
Efficacy Data:
- In vitro studies revealed that this compound is more potent than traditional antimalarials like chloroquine and artemisinin against drug-resistant strains of P. yoelii.
- The compound's effects on parasite growth were monitored using p-LDH activity assays, showing that this compound significantly reduces p-LDH activity in trophozoites while having less impact on ring-stage parasites .
Anticancer Activity
This compound's anticancer properties have been explored across various cancer cell lines. It has been shown to induce apoptosis through several pathways:
Case Studies:
- Oral Squamous Cell Carcinoma (OSCC) : this compound treatment led to increased splicing of XBP1 and activation of UPR-associated genes, resulting in cell death in OSCC models .
- Acute Lymphoblastic Leukemia : In these cells, this compound caused amino acid deprivation-induced stress responses that led to apoptosis .
Biological Activity Summary Table
Q & A
Basic Research Questions
Q. 1.1. What are the primary molecular targets of borrelidin, and how can researchers validate their role in its anti-angiogenic activity?
this compound inhibits threonyl-tRNA synthetase (ThrRS), disrupting protein synthesis in both prokaryotic and eukaryotic cells . To validate its anti-angiogenic mechanism (IC50 = 0.8 nM), researchers should:
- Use in vitro assays like endothelial tube formation assays (e.g., human umbilical vein endothelial cells [HUVECs]) with ThrRS activity measured via ATP-PPi exchange assays .
- Confirm target engagement via fluorescence quenching or circular dichroism (CD) spectroscopy to study ThrRS-borrelidin binding .
- Compare results with threonine rescue experiments, where exogenous threonine reverses this compound’s growth-inhibitory effects .
Q. 1.2. How should researchers design dose-response experiments to assess this compound’s cytotoxicity in cancer cell lines?
- Use standardized assays (e.g., MTT or CellTiter-Glo) across multiple time points (24–72 hours) to capture time-dependence .
- Include IC50 calculations for HCC cells (e.g., HepG2: 6.7 ± 0.3 mM; SMMC7721: 1.5 ± 0.2 mM) .
- Control for off-target effects by co-administering ThrRS substrates (e.g., threonine) or using ThrRS-knockout cell models .
Q. 1.3. What methodologies are recommended to study this compound’s impact on cell cycle arrest and apoptosis?
- Flow cytometry with propidium iodide staining for cell cycle analysis (e.g., G0/G1 arrest in HCC cells) .
- Western blotting for apoptosis markers (cleaved caspase-3/9, Bax/Bcl-2 ratio) and cell cycle regulators (p21, cyclin D1/D3/E1) .
- RNA-seq or qPCR to identify downstream pathways (e.g., MAPK signaling) .
Advanced Research Questions
Q. 2.1. How can researchers resolve contradictions between this compound’s potent in vitro anti-tumor activity and its poor pharmacokinetic properties in vivo?
- Conduct structure-activity relationship (SAR) studies to modify this compound’s macrocyclic structure, focusing on metabolic stability .
- Use pharmacokinetic (PK) modeling to optimize dosing regimens, leveraging in vivo xenograft data (e.g., SMMC7721 models with tumor volume reduction metrics) .
- Explore prodrug strategies or nanoparticle delivery systems to enhance bioavailability .
Q. 2.2. What experimental approaches can clarify this compound’s dual role in inducing ER stress and apoptosis via the unfolded protein response (UPR)?
- Employ CRISPR-Cas9 knockout models for UPR sensors (PERK, GCN2) to dissect their contributions to CHOP-dependent apoptosis .
- Monitor XBP1 splicing via RT-PCR as a marker of ER stress .
- Compare transcriptomic profiles (RNA-seq) of this compound-treated vs. untreated cells to map UPR pathway activation .
Q. 2.3. How can researchers address the selectivity challenge of this compound, which inhibits both bacterial and human ThrRS, leading to toxicity?
- Perform cryo-EM or X-ray crystallography to identify species-specific binding residues (e.g., Tyr313 in human ThrRS) .
- Develop this compound analogs using computational docking to minimize human ThrRS affinity while retaining antimicrobial activity .
- Validate selectivity in co-culture models (e.g., human cells + bacterial pathogens) to assess therapeutic windows .
Q. 2.4. What strategies are effective for reconciling divergent IC50 values reported for this compound across studies (e.g., anti-malarial vs. anti-angiogenic)?
- Standardize assay conditions (e.g., threonine concentration, cell density) to minimize variability .
- Use orthogonal validation methods (e.g., isothermal titration calorimetry for ThrRS inhibition vs. phenotypic assays for angiogenesis) .
- Publish raw datasets and statistical analyses to facilitate meta-analyses .
Q. Methodological Best Practices
Q. 3.1. How should researchers optimize this compound’s solubility and stability in cell culture media?
- Prepare stock solutions in DMSO (≤0.1% final concentration) to avoid precipitation .
- Validate stability via HPLC at 24-hour intervals under culture conditions (37°C, 5% CO2) .
- Use freshly dissolved this compound for time-course experiments to mitigate degradation .
Q. 3.2. What controls are essential when studying this compound’s anti-metastatic effects in migration/invasion assays?
特性
CAS番号 |
7184-60-3 |
---|---|
分子式 |
C28H43NO6 |
分子量 |
489.6 g/mol |
IUPAC名 |
2-[(4E,6E)-7-cyano-8,16-dihydroxy-9,11,13,15-tetramethyl-18-oxo-1-oxacyclooctadeca-4,6-dien-2-yl]cyclopentane-1-carboxylic acid |
InChI |
InChI=1S/C28H43NO6/c1-17-12-18(2)14-20(4)27(32)21(16-29)8-5-6-11-25(22-9-7-10-23(22)28(33)34)35-26(31)15-24(30)19(3)13-17/h5-6,8,17-20,22-25,27,30,32H,7,9-15H2,1-4H3,(H,33,34)/b6-5+,21-8+ |
InChIキー |
OJCKRNPLOZHAOU-SLNPHPKOSA-N |
SMILES |
CC1CC(CC(C(C(=CC=CCC(OC(=O)CC(C(C1)C)O)C2CCCC2C(=O)O)C#N)O)C)C |
異性体SMILES |
CC1CC(CC(C(/C(=C/C=C/CC(OC(=O)CC(C(C1)C)O)C2CCCC2C(=O)O)/C#N)O)C)C |
正規SMILES |
CC1CC(CC(C(C(=CC=CCC(OC(=O)CC(C(C1)C)O)C2CCCC2C(=O)O)C#N)O)C)C |
外観 |
White Lyophilisate |
ピクトグラム |
Irritant |
同義語 |
(1R,2R)-2-[(2S,4E,6Z,8R,9S,11R,13S,15S,16S)-7-Cyano-8,16-dihydroxy-9,11,13,15-tetramethyl-18-oxooxacyclooctadeca-4,6-dien-2-yl]-Cyclopentanecarboxylic Acid; [2S-[2R*(1S*,2S*),4E,6Z,8S*,9R*,11S*,13R*,15R*,16R*]]-2-(7-Cyano-8,16-dihydroxy-9,11,13,15-te |
製品の起源 |
United States |
Retrosynthesis Analysis
AI-Powered Synthesis Planning: Our tool employs the Template_relevance Pistachio, Template_relevance Bkms_metabolic, Template_relevance Pistachio_ringbreaker, Template_relevance Reaxys, Template_relevance Reaxys_biocatalysis model, leveraging a vast database of chemical reactions to predict feasible synthetic routes.
One-Step Synthesis Focus: Specifically designed for one-step synthesis, it provides concise and direct routes for your target compounds, streamlining the synthesis process.
Accurate Predictions: Utilizing the extensive PISTACHIO, BKMS_METABOLIC, PISTACHIO_RINGBREAKER, REAXYS, REAXYS_BIOCATALYSIS database, our tool offers high-accuracy predictions, reflecting the latest in chemical research and data.
Strategy Settings
Precursor scoring | Relevance Heuristic |
---|---|
Min. plausibility | 0.01 |
Model | Template_relevance |
Template Set | Pistachio/Bkms_metabolic/Pistachio_ringbreaker/Reaxys/Reaxys_biocatalysis |
Top-N result to add to graph | 6 |
Feasible Synthetic Routes
試験管内研究製品の免責事項と情報
BenchChemで提示されるすべての記事および製品情報は、情報提供を目的としています。BenchChemで購入可能な製品は、生体外研究のために特別に設計されています。生体外研究は、ラテン語の "in glass" に由来し、生物体の外で行われる実験を指します。これらの製品は医薬品または薬として分類されておらず、FDAから任何の医療状態、病気、または疾患の予防、治療、または治癒のために承認されていません。これらの製品を人間または動物に体内に導入する形態は、法律により厳格に禁止されています。これらのガイドラインに従うことは、研究と実験において法的および倫理的な基準の遵守を確実にするために重要です。