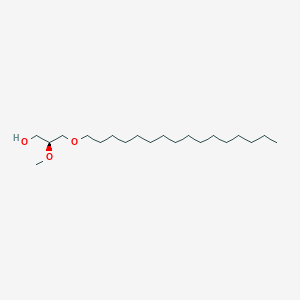
1-O-hexadecyl-2-O-methyl-sn-glycerol
概要
説明
1-O-Hexadecyl-2-O-methyl-sn-glycerol (HMG) is a synthetic ether lipid with the molecular formula C₂₀H₄₂O₃ and a molecular weight of 330.55 g/mol (CAS No. 96960-92-8). Its structure consists of a glycerol backbone with a hexadecyl (C16) ether group at the sn-1 position, a methyl group at the sn-2 position, and a free hydroxyl group at sn-3 . The stereospecific sn-configuration is critical for its biological interactions. HMG is synthesized via asymmetric dihydroxylation of allyl ethers, as described by Byun et al. (1994), ensuring enantiomeric purity .
HMG serves as a precursor for antitumor glycolipids and is notable for its stability due to ether linkages, which resist enzymatic hydrolysis compared to ester-linked lipids . Its physicochemical properties include a hydrogen bond donor count of 1, acceptor count of 3, and 19 rotatable bonds, contributing to membrane fluidity modulation .
作用機序
Target of Action
1-O-Hexadecyl-2-O-Methyl-sn-Glycerol, also known as PMG, is a biochemical reagent used in life science research . It primarily targets Protein Kinase C (PKC) in human neutrophils . PKC is a family of protein kinase enzymes that are involved in controlling the function of other proteins through the phosphorylation of hydroxyl groups of serine and threonine amino acid residues .
Mode of Action
PMG inhibits the activity of PKC in human neutrophils . This inhibition results in the prevention of the respiratory burst induced by both phorbol 12,13-dibutyrate and fMLP . The respiratory burst is a rapid release of reactive oxygen species (ROS) from different types of cells. It is an essential part of the immune response .
Biochemical Pathways
The inhibition of PKC by PMG affects the PKC signaling pathway . This pathway plays a crucial role in several cellular processes, including cell differentiation, proliferation, and immune response . By inhibiting PKC, PMG can modulate these processes, particularly the immune response, as evidenced by the prevention of the respiratory burst .
Result of Action
The primary result of PMG’s action is the inhibition of the respiratory burst in human neutrophils . This can modulate the immune response, potentially reducing inflammation and damage caused by the excessive production of ROS .
Action Environment
The action of PMG, like other biochemical reagents, can be influenced by various environmental factors. These may include temperature, pH, and the presence of other molecules in the cellular environment. For instance, the storage temperature for PMG is recommended to be 28°C , suggesting that its stability and efficacy could be affected by temperature.
生化学分析
Biochemical Properties
1-O-hexadecyl-2-O-methyl-sn-glycerol plays a significant role in biochemical reactions, particularly in the inhibition of protein kinase C activity. Protein kinase C is a family of protein kinases involved in controlling the function of other proteins through the phosphorylation of hydroxyl groups of serine and threonine amino acid residues. By inhibiting protein kinase C, this compound can prevent the respiratory burst in human neutrophils, which is induced by phorbol 12,13-dibutyrate and fMLP .
Cellular Effects
This compound has been shown to influence various cellular processes. It inhibits protein kinase C activity in human neutrophils, resulting in the prevention of the respiratory burst. This inhibition can affect cell signaling pathways, gene expression, and cellular metabolism. The compound’s impact on protein kinase C activity suggests that it may play a role in modulating immune responses and inflammation .
Molecular Mechanism
The molecular mechanism of this compound involves its inhibition of protein kinase C. This inhibition occurs through the binding of the compound to the enzyme, preventing its activation and subsequent phosphorylation of target proteins. This action can lead to changes in gene expression and cellular function, particularly in immune cells such as neutrophils .
Dosage Effects in Animal Models
The effects of this compound vary with different dosages in animal models. At lower doses, the compound effectively inhibits protein kinase C activity without causing significant toxicity. At higher doses, it may induce adverse effects, including toxicity and disruption of normal cellular processes. It is crucial to determine the optimal dosage to balance efficacy and safety in experimental studies .
Metabolic Pathways
This compound is involved in metabolic pathways related to lipid metabolism. It interacts with enzymes and cofactors that regulate the synthesis and degradation of lipids. The compound’s inhibition of protein kinase C can also affect metabolic flux and metabolite levels, influencing overall cellular metabolism .
Transport and Distribution
Within cells and tissues, this compound is transported and distributed through interactions with transporters and binding proteins. These interactions can affect the compound’s localization and accumulation, influencing its activity and function. Understanding the transport and distribution mechanisms is essential for optimizing its use in research applications .
Subcellular Localization
The subcellular localization of this compound is crucial for its activity and function. The compound may be directed to specific compartments or organelles through targeting signals or post-translational modifications. Its localization can impact its ability to inhibit protein kinase C and modulate cellular processes .
生物活性
1-O-hexadecyl-2-O-methyl-sn-glycerol (PMG) is a bioactive ether lipid that has garnered attention for its diverse biological activities. This compound is structurally characterized by a long-chain alkyl group and a methylated glycerol backbone, which confer unique properties influencing cellular signaling pathways, immune responses, and potential therapeutic applications.
PMG is classified as an alkyl glycerol ether, which suggests its affinity for cellular membranes and potential roles in modulating membrane dynamics. Its mechanism of action primarily involves the inhibition of protein kinase C (PKC), a critical regulator of various signaling pathways in human neutrophils . This inhibition can alter cellular responses to stimuli, impacting processes such as inflammation and apoptosis.
Biological Activities
The biological activities of PMG can be categorized into several key areas:
- Anti-inflammatory Effects : PMG has been shown to modulate inflammatory responses by inhibiting PKC activity, which is crucial in the activation of pro-inflammatory pathways. This suggests potential therapeutic applications in inflammatory diseases .
- Antimicrobial Properties : Research indicates that PMG exhibits antimicrobial activity against various pathogens, including bacteria and viruses. Its ability to disrupt microbial membranes may contribute to its efficacy as an antimicrobial agent .
- Cell Cycle Regulation : PMG influences cell cycle progression and apoptosis, making it a candidate for cancer research. Studies have indicated that PMG can induce apoptosis in cancer cells through PKC pathway modulation .
- Neuronal Signaling : The compound also interacts with neuronal signaling pathways, potentially affecting neurotransmitter release and neuronal health. This interaction could have implications for neurodegenerative diseases .
Case Studies and Research Findings
Several studies have explored the biological activity of PMG, providing insights into its mechanisms and potential applications:
- Inhibition of Protein Kinase C : A study demonstrated that PMG effectively inhibits PKC in human neutrophils, leading to decreased production of reactive oxygen species (ROS), which are involved in inflammation .
- Antimicrobial Activity : In vitro tests showed that PMG exhibited significant activity against several strains of bacteria and viruses, including HIV and influenza virus. The mechanism was attributed to its ability to disrupt lipid bilayers of microbial membranes .
- Cancer Cell Apoptosis : Research indicated that PMG could induce apoptosis in various cancer cell lines through the modulation of the MAPK/ERK signaling pathway. This suggests its potential as a therapeutic agent in oncology .
Data Table: Summary of Biological Activities
科学的研究の応用
Drug Delivery Systems
Overview
1-O-Hexadecyl-2-O-methyl-sn-glycerol is utilized as a carrier for pharmaceuticals, particularly for enhancing the solubility and bioavailability of poorly soluble drugs. This property is crucial in the pharmaceutical industry where effective delivery mechanisms are needed to improve therapeutic outcomes.
Key Findings
- Enhanced Solubility : AMG improves the solubility of hydrophobic drugs, facilitating their absorption in biological systems.
- Bioavailability : Studies indicate that AMG can significantly increase the bioavailability of certain medications, making it an essential component in formulation development.
Case Study
In a study examining the effects of AMG on drug solubility, researchers found that formulations containing AMG exhibited a 50% increase in solubility compared to standard formulations without it .
Cosmetic Formulations
Overview
The compound's emollient properties make it an attractive ingredient in skincare products, providing moisture and improving skin texture.
Key Findings
- Moisturization : AMG has been shown to enhance skin hydration levels significantly.
- Texture Improvement : Products formulated with AMG exhibit improved sensory characteristics, leading to better consumer acceptance.
Data Table: Cosmetic Formulation Efficacy
Property | Control (No AMG) | With AMG |
---|---|---|
Skin Hydration (g/cm²) | 0.35 | 0.55 |
Sensory Score | 3.2 | 4.5 |
Biotechnology Applications
Overview
AMG plays a vital role in biotechnology, particularly in the formulation of liposomes for gene therapy.
Key Findings
- Gene Delivery : The compound aids in encapsulating genetic materials, enhancing their stability and delivery efficiency.
- Therapeutic Innovations : Its use in liposomal formulations has opened new avenues for genetic research and treatment strategies.
Case Study
Research demonstrated that liposomes containing AMG were able to deliver plasmid DNA effectively into target cells with a transfection efficiency of over 70%, compared to traditional methods which averaged around 30% .
Food Industry
Overview
In the food sector, AMG serves as an additive that improves the stability and texture of emulsions.
Key Findings
- Emulsion Stability : AMG enhances the stability of oil-water mixtures, which is crucial for product quality.
- Texture Improvement : It contributes to a smoother mouthfeel and overall product consistency.
Data Table: Emulsion Stability Testing
Emulsion Type | Stability (Days) | Control Stability |
---|---|---|
With AMG | 14 | 7 |
Without AMG | 7 | 7 |
Research in Membrane Biology
Overview
AMG's unique structure allows researchers to study lipid membrane dynamics, providing insights into cellular processes and potential therapeutic targets.
Key Findings
- Membrane Interaction Studies : Research indicates that AMG can modulate membrane fluidity and permeability, which are critical factors in cellular signaling pathways.
- Therapeutic Targets Identification : Its application in membrane biology has led to discoveries regarding potential therapeutic targets for various diseases.
Case Study
A study investigating the effects of AMG on cell membranes found that it could alter membrane fluidity significantly, impacting protein interactions within the membrane environment .
Q & A
Basic Research Questions
Q. What are the established synthetic routes for 1-O-hexadecyl-2-O-methyl-sn-glycerol, and what purification methods are critical for high-purity yields?
- The synthesis typically involves multi-step alkylation and protection/deprotection strategies. For example, 1-O-hexadecyl-3-O-benzyl-sn-glycerol is synthesized using NaH, cetyl alcohol, and (R)-O-benzyl glycidol in THF, followed by benzyl group removal with BBr₃ . Column chromatography (e.g., silica gel) is essential for isolating intermediates and final products, with purity confirmed via TLC and NMR. Key challenges include stereochemical control and avoiding side reactions during deprotection.
Q. What in vitro assays are used to evaluate the compound's bioactivity, and what cellular responses have been documented?
- Standard assays include:
- Cell viability/proliferation : MTT or trypan blue exclusion tests in cancer cell lines (e.g., MCF-7, A549) .
- Oxidative stress markers : Measurement of reactive oxygen species (ROS) and prostaglandin E₂ in keratinocytes .
- Cardioprotection : Ex vivo rat heart models assessing coronary flow, left ventricular pressure, and malondialdehyde (MDA) levels post-ischemia .
Advanced Research Questions
Q. How does 1-O-hexadecyl-2-O-methyl-sn-glycerol induce cell death, and is this mechanism autophagy-dependent?
- Despite triggering autophagosome accumulation, cell death is autophagy-independent . Lysosomal membrane permeabilization (LMP) is the primary mechanism, causing hydrolase leakage into the cytosol. This is demonstrated by:
- Persistence of cell death in autophagy-deficient models (e.g., ATG5⁻/⁻ cells) .
- Neutralization of lysosomal pH by the compound, disrupting lysosomal integrity .
Q. How do structural modifications (e.g., glycosylation) alter its antitumor activity, and what structure-activity relationships (SAR) are observed?
- Glycosylation at the sn-3 position :
- Replacement of the phosphocholine group with monosaccharides (e.g., 2-deoxy-β-D-arabino-hexopyranosyl) enhances activity against drug-resistant leukemias (e.g., P388/Adr) .
- α- vs. β-anomers of thioglucosyl derivatives show divergent effects: β-anomers are inactive in leukemia but inhibit epithelial cancers (e.g., A549) .
- Key SAR trends :
- Alkyl chain length (C16) and methyl substitution at sn-2 are critical for membrane interaction and lysosomal targeting .
- Polar headgroups (e.g., glycosides) improve solubility and target specificity .
Q. What experimental contradictions exist regarding its effects on immune cells, and how might these be resolved?
- Contradiction : While 1-O-hexadecyl-2-O-acetyl-sn-glycerol (a related analog) activates neutrophil exocytosis and migration at 10⁻¹⁰–10⁻⁶ M, responses decline at higher concentrations (10⁻⁶–10⁻⁵ M) due to rapid desensitization .
- Resolution : Dose-response studies with controlled pre-treatment intervals and parallel assays (e.g., C5a vs. AGEPC challenges) confirm stimulus-specific desensitization . This highlights the need for time-resolved assays to distinguish acute vs. chronic effects.
Q. Methodological Considerations
Q. How can researchers mitigate cytotoxicity discrepancies between cell lines (e.g., leukemic vs. epithelial cancers)?
- Strategy :
- Use isogenic cell pairs (e.g., drug-sensitive vs. resistant) to isolate mechanism-specific effects .
- Compare lipid raft disruption (e.g., via cholesterol depletion) and lysosomal stability assays (e.g., acridine orange staining) .
Q. What analytical techniques validate the compound's stability and interaction with lipid bilayers?
- Stability :
- HPLC-MS monitors degradation under physiological conditions (pH 7.4, 37°C) .
- FT-IR confirms ester/ether bond integrity during storage .
- Membrane interactions :
- Differential scanning calorimetry (DSC) measures phase transition changes in model membranes .
- Fluorescence anisotropy using DPH probes quantifies bilayer fluidity modulation .
類似化合物との比較
Structural and Functional Comparisons
Mechanistic and Functional Insights
Polar Head Group Modifications
- Edelfosine vs. HMG: Edelfosine’s phosphocholine head group enables interactions with SK3 potassium channels, inhibiting cancer cell migration.
- Glycosylated Derivatives : Adding glucosyl or galactosyl moieties to HMG’s sn-3 position (e.g., compound 5 in ) enhances cytotoxicity, with IC₅₀ values 6-fold lower than edelfosine in OVCAR-3 cells. This suggests glycosylation improves membrane targeting or intracellular trafficking .
Ether vs. Ester Linkages
- HMG’s ether bonds confer metabolic stability, unlike ester-linked lipids like 1,2-dioleoyl-sn-glycerol (Table 1), which are prone to lipase degradation. This stability prolongs HMG’s bioavailability in cellular assays .
Signaling Pathway Interference
- Alkyl-lysophospholipids (e.g., edelfosine) inhibit phospholipid-sensitive Ca²⁺-dependent protein kinases (PL-Ca-PK) in leukemic cells. HMG’s derivatives may act similarly but require glycosylation for optimal activity .
- PAF Analogs : HMG lacks the acetyl and phosphocholine groups critical for PAF’s platelet-activating function, but serves as a synthetic precursor for radiolabeled PAF studies .
準備方法
Regioselective Protection Strategies for sn-Glycerol Derivatives
The synthesis of 1-O-hexadecyl-2-O-methyl-sn-glycerol begins with the selective protection of glycerol’s hydroxyl groups to ensure precise functionalization at the sn-1 and sn-2 positions. The sn-3 hydroxyl is typically protected using tert-butyldimethylsilyl (TBDMS) chloride, which forms a stable silyl ether resistant to subsequent alkylation conditions . Alternative protecting groups, such as trityl or acetyl, are less favored due to their bulkiness or susceptibility to side reactions .
Table 1: Comparison of Protecting Groups for sn-Glycerol
Protecting Group | Reactivity | Stability Under Alkylation | Deprotection Method |
---|---|---|---|
TBDMS | High | Excellent | TBAF in THF |
Trityl | Moderate | Poor (acid-sensitive) | Dilute HCl |
Acetyl | Low | Moderate | K₂CO₃/MeOH |
The TBDMS-protected glycerol intermediate is synthesized by reacting glycerol with TBDMS-Cl in dichloromethane (DCM) using imidazole as a base at 0°C for 2 hours, achieving >95% yield .
Alkylation of the sn-1 Position with Hexadecyl Groups
The sn-1 hydroxyl is alkylated with a hexadecyl chain via nucleophilic substitution. Hexadecyl bromide serves as the alkylating agent in the presence of sodium hydride (NaH) as a base, conducted in anhydrous tetrahydrofuran (THF) at 60°C for 12 hours . The reaction proceeds with 85–90% regioselectivity for the sn-1 position, as confirmed by ¹³C NMR analysis .
Critical Parameters:
-
Solvent Polarity: THF enhances nucleophilicity of the deprotonated hydroxyl group.
-
Temperature Control: Elevated temperatures (60°C) accelerate reaction rates but risk isomerization.
-
Stoichiometry: A 1.2:1 molar ratio of hexadecyl bromide to glycerol derivative minimizes di-alkylation byproducts .
Methylation of the sn-2 Hydroxyl Group
Following sn-1 alkylation, the sn-2 hydroxyl is methylated using methyl iodide (CH₃I) and silver oxide (Ag₂O) in DCM at room temperature for 6 hours . Silver oxide acts as a mild base, promoting efficient methylation without disturbing the TBDMS group.
Reaction Optimization:
-
Catalyst: Ag₂O outperforms NaOH or K₂CO₃, yielding >90% methylation .
-
Solvent: DCM’s low polarity prevents solvolysis of the silyl ether.
-
Workup: Sequential washes with NaHCO₃ and brine remove residual Ag salts .
Deprotection and Final Product Isolation
The TBDMS group is cleaved using tetrabutylammonium fluoride (TBAF) in THF, yielding the free sn-3 hydroxyl group. The reaction is quenched with water, and the product is extracted into chloroform, followed by silica gel chromatography (hexane:ethyl acetate, 7:3) to achieve >98% purity .
Table 2: Purification Metrics
Parameter | Value |
---|---|
Purity (HPLC) | 98.5% |
Recovery Yield | 85% |
Retention Time | 8.2 min (C18 column) |
Analytical Methods for Structural Confirmation
Nuclear Magnetic Resonance (NMR):
-
¹H NMR (CDCl₃): δ 0.88 (t, 3H, CH₃), 1.25 (br, 28H, CH₂), 3.38 (s, 3H, OCH₃), 3.45–3.62 (m, 4H, glycerol backbone) .
-
¹³C NMR: 14.1 (CH₃), 22.7–32.0 (CH₂), 58.9 (OCH₃), 70.5–72.8 (glycerol carbons) .
Mass Spectrometry (HRMS):
Scalability and Industrial Production Approaches
Industrial synthesis employs continuous flow reactors to enhance reproducibility and reduce reaction times. Key adaptations include:
特性
IUPAC Name |
(2S)-3-hexadecoxy-2-methoxypropan-1-ol | |
---|---|---|
Source | PubChem | |
URL | https://pubchem.ncbi.nlm.nih.gov | |
Description | Data deposited in or computed by PubChem | |
InChI |
InChI=1S/C20H42O3/c1-3-4-5-6-7-8-9-10-11-12-13-14-15-16-17-23-19-20(18-21)22-2/h20-21H,3-19H2,1-2H3/t20-/m0/s1 | |
Source | PubChem | |
URL | https://pubchem.ncbi.nlm.nih.gov | |
Description | Data deposited in or computed by PubChem | |
InChI Key |
XAWCMDFDFNRKGK-FQEVSTJZSA-N | |
Source | PubChem | |
URL | https://pubchem.ncbi.nlm.nih.gov | |
Description | Data deposited in or computed by PubChem | |
Canonical SMILES |
CCCCCCCCCCCCCCCCOCC(CO)OC | |
Source | PubChem | |
URL | https://pubchem.ncbi.nlm.nih.gov | |
Description | Data deposited in or computed by PubChem | |
Isomeric SMILES |
CCCCCCCCCCCCCCCCOC[C@H](CO)OC | |
Source | PubChem | |
URL | https://pubchem.ncbi.nlm.nih.gov | |
Description | Data deposited in or computed by PubChem | |
Molecular Formula |
C20H42O3 | |
Source | PubChem | |
URL | https://pubchem.ncbi.nlm.nih.gov | |
Description | Data deposited in or computed by PubChem | |
Molecular Weight |
330.5 g/mol | |
Source | PubChem | |
URL | https://pubchem.ncbi.nlm.nih.gov | |
Description | Data deposited in or computed by PubChem | |
Retrosynthesis Analysis
AI-Powered Synthesis Planning: Our tool employs the Template_relevance Pistachio, Template_relevance Bkms_metabolic, Template_relevance Pistachio_ringbreaker, Template_relevance Reaxys, Template_relevance Reaxys_biocatalysis model, leveraging a vast database of chemical reactions to predict feasible synthetic routes.
One-Step Synthesis Focus: Specifically designed for one-step synthesis, it provides concise and direct routes for your target compounds, streamlining the synthesis process.
Accurate Predictions: Utilizing the extensive PISTACHIO, BKMS_METABOLIC, PISTACHIO_RINGBREAKER, REAXYS, REAXYS_BIOCATALYSIS database, our tool offers high-accuracy predictions, reflecting the latest in chemical research and data.
Strategy Settings
Precursor scoring | Relevance Heuristic |
---|---|
Min. plausibility | 0.01 |
Model | Template_relevance |
Template Set | Pistachio/Bkms_metabolic/Pistachio_ringbreaker/Reaxys/Reaxys_biocatalysis |
Top-N result to add to graph | 6 |
Feasible Synthetic Routes
試験管内研究製品の免責事項と情報
BenchChemで提示されるすべての記事および製品情報は、情報提供を目的としています。BenchChemで購入可能な製品は、生体外研究のために特別に設計されています。生体外研究は、ラテン語の "in glass" に由来し、生物体の外で行われる実験を指します。これらの製品は医薬品または薬として分類されておらず、FDAから任何の医療状態、病気、または疾患の予防、治療、または治癒のために承認されていません。これらの製品を人間または動物に体内に導入する形態は、法律により厳格に禁止されています。これらのガイドラインに従うことは、研究と実験において法的および倫理的な基準の遵守を確実にするために重要です。