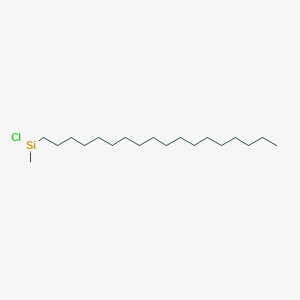
Octadecylmethylchlorosilane
説明
Octadecylmethylchlorosilane (CAS 18643-08-8), also known as dimethyloctadecylchlorosilane, is a long-chain organosilane with the molecular formula C₂₀H₄₃ClSi. It consists of an octadecyl (C₁₈H₃₇) group, two methyl groups, and a reactive chlorine atom bonded to a silicon center . This compound is primarily used in the synthesis of silicone oils, silane-modified polymers, and as a derivatization agent in chromatography due to its ability to functionalize surfaces with hydrophobic alkyl chains . It appears as a colorless clear liquid or white crystalline solid with a melting point of 28–30°C and a boiling point of 145–155°C under reduced pressure (0.005 mmHg) .
準備方法
Redistribution Reactions Using Quaternary Ammonium Catalysts
The redistribution of chlorosilanes in the presence of functionalized catalysts represents a primary industrial route for synthesizing mixed-substituent chlorosilanes. As detailed in US5252768A, dimethylchlorosilane production involves the rearrangement of dimethyldichlorosilane (Me₂SiCl₂) with methylchlorosilanes or methylsilanes over catalysts bearing quaternary ammonium groups . For octadecylmethylchlorosilane, this methodology is adapted by introducing octadecyl-containing silanes or catalysts.
Catalyst Design for Selective Alkyl Transfer
Key to this process is the use of catalysts with covalently bonded N,N-dimethyloctadecylammonium functionalities, such as trimethoxysilyl-3-propyl-N,N,N-dimethyloctadecylammonium chloride [(MeO)₃SiCH₂CH₂CH₂NMe₂C₁₈H₃₇⁺Cl⁻] . These catalysts facilitate Si–Cl and Si–H bond redistribution while preventing scrambling of Si–C bonds, ensuring retention of the methyl and octadecyl groups. The catalyst’s octadecyl chain enhances lipophilicity, improving compatibility with long-chain silane reactants.
Reaction Conditions and Mechanistic Insights
The redistribution occurs in inert solvents (e.g., toluene, chlorobenzene) at 50–150°C under 3–8 bar pressure . A representative reaction pathway involves:
2\text{SiCl}2 + \text{C}{18}\text{H}{37}\text{SiCl}3 \xrightarrow{\text{Catalyst}} \text{Me(C}{18}\text{H}{37})\text{SiCl}2 + \text{by-products}
Gas-phase reactions in fixed-bed reactors achieve higher selectivity due to minimized side reactions. Fractional distillation separates this compound (boiling point: ~250°C) from higher-boiling octadecyltrichlorosilane and lower-boiling methylsilanes .
Disproportionation of Methyloctadecyldichlorosilane
CN102093402B discloses a solvent-assisted disproportionation strategy for dimethylchlorosilane synthesis , which can be extrapolated to this compound. Methylhydrogenosiloxanes serve as hydrogen donors, reacting with methyloctadecyldichlorosilane in the presence of tetrabutylammonium chloride:
{18}\text{H}{37})\text{SiCl}2 \rightleftharpoons \text{Me}2\text{SiCl}2 + \text{C}{18}\text{H}{37}\text{SiCl}3
Using hexamethylphosphoramide (HMPA) as a solvent enhances reaction rates by stabilizing ionic intermediates . Continuous fractional distillation shifts equilibrium toward this compound, achieving 45–58% yields .
Comparative Analysis of Synthetic Routes
Method | Catalyst System | Temperature Range | Yield (%) | Selectivity Challenges |
---|---|---|---|---|
Redistribution | Quaternary ammonium chlorides | 50–150°C | 60–75 | By-product separation |
Hydrosilylation | Pt complexes | 80–120°C | 70–85 | Isomer control |
Disproportionation | Tetrabutylammonium chloride | 100–150°C | 45–58 | Equilibrium limitations |
Hydrosilylation offers superior yields but requires costly platinum catalysts. Redistribution reactions, while scalable, demand rigorous purification due to co-produced silanes.
Industrial-Scale Process Optimization
Catalyst Recycling and Stability
Quaternary ammonium catalysts in redistribution reactions exhibit gradual deactivation due to chloride leaching. Regeneration via treatment with HCl-saturated solvents restores activity to 90% of initial levels . Fluidized-bed reactors mitigate catalyst sintering, extending operational lifetimes to >1,000 hours .
Solvent Selection and Environmental Impact
Chlorobenzene and HMPA, though effective, pose toxicity concerns. Recent advances employ ionic liquids like 1-butyl-3-methylimidazolium chloride, which improve catalyst retention and reduce volatile organic compound emissions .
化学反応の分析
Types of Reactions: Octadecylmethylchlorosilane undergoes various chemical reactions, including:
Substitution Reactions: The chlorine atom can be substituted with other functional groups, such as hydroxyl or amino groups, under appropriate conditions.
Hydrolysis: In the presence of water or moisture, this compound can hydrolyze to form silanols and hydrochloric acid.
Common Reagents and Conditions:
Substitution Reactions: Common reagents include alcohols, amines, and other nucleophiles. These reactions typically occur under mild conditions with the use of a catalyst.
Hydrolysis: This reaction occurs readily in the presence of water or moisture, often at room temperature.
Major Products Formed:
Substitution Reactions: Depending on the nucleophile used, the major products can include octadecylmethylsilanol, octadecylmethylamine, etc.
Hydrolysis: The primary products are octadecylmethylsilanol and hydrochloric acid.
科学的研究の応用
Surface Modification
One of the primary applications of ODMCS is in surface modification. By forming self-assembled monolayers (SAMs) on substrates, ODMCS can significantly alter the surface properties of materials:
- Hydrophobic Coatings : ODMCS is used to create hydrophobic surfaces on glass, metals, and polymers. This is particularly beneficial in applications requiring water-repellent properties, such as in coatings for outdoor equipment or in microfluidic devices .
- Biocompatibility : The hydrophobic nature of ODMCS can enhance the biocompatibility of medical devices by reducing protein adsorption and cell adhesion, making it suitable for implants and drug delivery systems .
Materials Science
In materials science, ODMCS plays a crucial role in enhancing the mechanical and thermal properties of composites:
- Composite Materials : The incorporation of ODMCS into polymer matrices improves the dispersion of fillers and enhances the mechanical strength of composites. This is particularly useful in creating lightweight yet strong materials for automotive and aerospace applications .
- Nanotechnology : ODMCS is utilized in the synthesis of nanoparticles and nanocomposites. Its ability to modify the surface properties of nanoparticles enhances their stability and functionality in various applications, including catalysis and drug delivery .
Nanofabrication
The use of ODMCS in nanofabrication techniques has opened new avenues for creating advanced materials:
- Template-Assisted Fabrication : ODMCS can be used to modify templates that guide the growth of nanostructures. This technique is crucial in developing nanoscale devices for electronics and photonics .
- Functionalization of Nanomaterials : The ability to functionalize nanoparticles with ODMCS allows for tailored interactions with biological systems or specific chemical environments, enhancing their applicability in sensing and imaging technologies .
Biomedical Applications
In the biomedical field, ODMCS has shown promise in various applications:
- Drug Delivery Systems : By modifying nanoparticles with ODMCS, researchers have developed drug delivery systems that exhibit improved release profiles and targeted delivery capabilities .
- Tissue Engineering : The hydrophobicity imparted by ODMCS can be beneficial in creating scaffolds that promote cell growth while minimizing non-specific protein adsorption, thus enhancing tissue engineering strategies .
Case Study 1: Hydrophobic Coating Development
A study demonstrated the effectiveness of ODMCS in creating hydrophobic coatings on glass substrates. The resulting surfaces exhibited a contact angle greater than 100 degrees, indicating excellent water repellency. This property was leveraged to improve the durability of outdoor glass installations.
Case Study 2: Drug Delivery Enhancement
Research involving ODMCS-modified nanoparticles showed a significant increase in drug loading capacity and controlled release rates compared to unmodified counterparts. This advancement highlights its potential in improving therapeutic outcomes for cancer treatments.
Summary Table of Applications
Application Area | Specific Use Cases | Benefits |
---|---|---|
Surface Modification | Hydrophobic coatings on glass and metals | Enhanced water repellency |
Materials Science | Composite materials for automotive applications | Improved mechanical strength |
Nanotechnology | Template-assisted fabrication | Creation of nanoscale devices |
Biomedical Applications | Drug delivery systems and tissue engineering | Targeted delivery and enhanced biocompatibility |
作用機序
The mechanism of action of octadecylmethylchlorosilane primarily involves its ability to form strong covalent bonds with various substrates. The chlorine atom in the compound can react with hydroxyl groups on surfaces, leading to the formation of stable siloxane bonds. This reaction imparts hydrophobic properties to the surface, making it water-repellent .
類似化合物との比較
Octadecyltrichlorosilane (C₁₈H₃₇Cl₃Si)
- Structure and Reactivity: Contains three reactive chlorine atoms on silicon, enabling strong covalent bonding to hydroxylated surfaces (e.g., glass, silica). This promotes the formation of self-assembled monolayers (SAMs) for hydrophobic coatings .
- Applications: Widely used in surface modification, nanotechnology, and anti-corrosion coatings. Its high reactivity allows dense surface coverage compared to mono- or dichlorosilanes .
- Safety: Classified as corrosive (UN 1800) with Protective Action Criteria (PAC) values of 0.6 ppm (PAC-1), 7.3 ppm (PAC-2), and 33 ppm (PAC-3).
Dichloromethyloctadecylsilane (C₁₉H₃₉Cl₂Si)
- Structure and Reactivity: Features two chlorine atoms, offering intermediate reactivity between trichloro- and monochloro-silanes. The formula is CH₃(C₁₈H₃₇)SiCl₂ .
- Applications : Used in polymer cross-linking and specialty coatings where controlled reactivity is required.
Dimethyl Dichlorosilane (C₂H₆Cl₂Si)
- Structure and Reactivity : A smaller molecule with two chlorines and two methyl groups. Highly reactive due to multiple leaving groups, leading to rapid hydrolysis .
- Applications: Precursor for silicone resins and sealants. No occupational exposure limits are established, but it requires stringent handling due to corrosive and irritant properties .
Trimethylchlorosilane (C₃H₉ClSi)
- Structure and Reactivity : Contains three methyl groups and one chlorine, reducing reactivity compared to di- or trichlorosilanes. Formula: (CH₃)₃SiCl .
- Applications: Used in end-capping silicone polymers to terminate chain growth.
Comparative Data Tables
Table 1: Structural and Physical Properties
Compound | Molecular Formula | Molecular Weight (g/mol) | Chlorine Atoms | Physical State (25°C) | Boiling Point (°C) |
---|---|---|---|---|---|
Octadecylmethylchlorosilane | C₂₀H₄₃ClSi | 347.08 | 1 | Solid/Liquid | 145–155 (0.005 mmHg) |
Octadecyltrichlorosilane | C₁₈H₃₇Cl₃Si | 387.93 | 3 | Liquid | 220–230 (lit.) |
Dichloromethyloctadecylsilane | C₁₉H₃₉Cl₂Si | 340.46 | 2 | Liquid | Not reported |
Dimethyl Dichlorosilane | C₂H₆Cl₂Si | 129.06 | 2 | Liquid | 70–72 |
Trimethylchlorosilane | C₃H₉ClSi | 108.64 | 1 | Liquid | 57–58 |
生物活性
Octadecylmethylchlorosilane (OMCS) is an organosilicon compound characterized by its long hydrocarbon chain and unique chemical properties. Its structure comprises a silicon atom bonded to two methyl groups and a chlorine atom, with an octadecyl group (C18H37) attached. This configuration imparts specific biological activities and applications, particularly in surface modification, drug delivery systems, and as a coupling agent in various biochemical processes.
- Molecular Formula : C19H41ClSi
- Molecular Weight : 348.06 g/mol
- Structure : Features a hydrophobic octadecyl chain that enhances its interaction with biological membranes.
OMCS functions primarily through its ability to modify surface properties. The chlorine atom reacts with hydroxyl groups on surfaces, facilitating covalent bonding. This interaction alters the wettability and hydrophobicity of the surfaces, making them more resistant to water and other polar solvents, which can influence biological interactions.
Biological Activity
The biological activity of OMCS is significant in several areas:
1. Cytotoxicity
Studies indicate that OMCS exhibits cytotoxic effects on various cell lines, attributed to its hydrophobic nature and chlorine content. The interaction with cell membranes can disrupt cellular integrity, leading to apoptosis or necrosis in sensitive cell types .
2. Surface Modification
OMCS is widely used in surface modification due to its ability to create hydrophobic surfaces. This property is beneficial in biomedical applications where reduced protein adsorption is desired, such as in the development of blood-contacting devices .
3. Drug Delivery Systems
Research has shown that OMCS can enhance the delivery efficiency of drugs by modifying the surface characteristics of nanoparticles or drug carriers. The hydrophobic nature of OMCS can improve the stability and bioavailability of hydrophobic drugs .
Case Studies
Several studies have explored the biological activity and applications of OMCS:
- Surface Modification for Drug Delivery : In a study investigating the use of OMCS-coated nanoparticles for drug delivery, it was found that the modified surfaces significantly improved drug loading and release profiles compared to unmodified carriers .
- Cytotoxicity Assessment : A cytotoxicity study on mammalian cell lines revealed that exposure to OMCS resulted in dose-dependent cell death, highlighting its potential risks in biomedical applications .
Comparison of Biological Activities
Property | This compound | Other Silanes |
---|---|---|
Cytotoxicity | Moderate to High | Varies (generally lower) |
Hydrophobicity | High | Moderate |
Surface Modification Efficacy | High | Varies |
Grafting Efficiency
Grafting Agent | Water Contact Angle (°) | Sorption Capacity (g/g) |
---|---|---|
This compound | 150 | 6.5–10.8 |
Octadecyltrichlorosilane | 169 | 3.86–5.27 |
Dimethylchlorosilane | 142 | 2.0 |
Q & A
Basic Research Questions
Q. What experimental protocols are recommended for synthesizing and purifying octadecylmethylchlorosilane to achieve high purity?
Methodological Answer:
- Synthesis Optimization : Use inert atmosphere techniques (e.g., nitrogen or argon) during silane reactions to prevent hydrolysis. Reflux conditions should be controlled at temperatures between 80–120°C, depending on solvent stability (e.g., toluene or THF) .
- Purification : Distillation under reduced pressure or column chromatography with non-polar solvents (e.g., hexane) is advised. Monitor purity via thin-layer chromatography (TLC) and confirm using ¹H/¹³C NMR and FTIR spectroscopy to detect residual hydroxyl groups or byproducts .
- Critical Step : Detailed documentation of reaction times, solvent ratios, and catalyst concentrations (if applicable) is essential for reproducibility .
Q. What safety protocols should be followed when handling this compound in laboratory settings?
Methodological Answer:
- Exposure Limits : While specific ERPGs (Emergency Response Planning Guidelines) for this compound are unavailable, analogous chlorosilanes like octadecyltrichlorosilane have PAC-2 (7.3 ppm) and PAC-3 (33 ppm) thresholds for airborne exposure .
- Handling : Use fume hoods, chemical-resistant gloves (e.g., nitrile), and safety goggles. Neutralize spills with dry sand or sodium bicarbonate, avoiding water due to exothermic hydrolysis .
- First Aid : Immediate rinsing with water for skin/eye contact and medical consultation are mandatory. Provide safety data sheets (SDS) to healthcare providers .
Q. How can researchers characterize the structural integrity of this compound post-synthesis?
Methodological Answer:
- Spectroscopic Techniques :
- NMR : Analyze ¹H (δ 0.5–1.5 ppm for Si-CH₃ and alkyl chains) and ²⁹Si NMR (δ 10–20 ppm for Si-Cl bonds) to confirm substitution patterns .
- FTIR : Identify Si-C (~1250 cm⁻¹) and Si-Cl (~500 cm⁻¹) vibrational bands. Absence of O-H stretches (~3400 cm⁻¹) indicates successful moisture avoidance .
- Elemental Analysis : Validate C, H, and Cl content to assess stoichiometric accuracy .
Q. What are common side reactions during this compound synthesis, and how can they be mitigated?
Methodological Answer:
- Hydrolysis : Rapid reaction with ambient moisture generates HCl and silanols. Use anhydrous solvents, molecular sieves, and inert atmospheres .
- Disproportionation : Elevated temperatures may cause Si-Cl bond redistribution. Monitor reaction kinetics via gas chromatography (GC) or HPLC to detect intermediates .
Advanced Research Questions
Q. How can this compound be utilized in surface modification, and what analytical methods validate coating efficiency?
Methodological Answer:
- Application : Form self-assembled monolayers (SAMs) on hydroxylated substrates (e.g., SiO₂). Optimize deposition time (1–24 hours) and solvent polarity (e.g., toluene vs. hexane) to control monolayer density .
- Validation Techniques :
- X-ray Photoelectron Spectroscopy (XPS) : Quantify Si and Cl atomic percentages to confirm covalent bonding.
- Atomic Force Microscopy (AFM) : Measure surface roughness (<1 nm RMS for high-quality SAMs) .
- Contact Angle Goniometry : Hydrophobic surfaces should exhibit water contact angles >100° .
Q. How does the stability of this compound-based coatings vary under extreme pH or thermal conditions?
Methodological Answer:
- Thermal Stability : Use thermogravimetric analysis (TGA) to assess decomposition temperatures (typically >200°C for alkylsilanes).
- Chemical Stability : Immerse coatings in acidic (pH 2) or basic (pH 12) solutions for 24–72 hours. Monitor degradation via ellipsometry (thickness loss) or FTIR (Si-O-Si network formation) .
Q. What computational models are effective for predicting the reactivity of this compound with different substrates?
Methodological Answer:
- Density Functional Theory (DFT) : Simulate Si-Cl bond cleavage energetics and adsorption mechanisms on metal oxides (e.g., Al₂O₃, TiO₂). Focus on charge transfer and bond angles .
- Molecular Dynamics (MD) : Model SAM formation kinetics and packing density variations with alkyl chain length .
Q. How should researchers resolve contradictions in experimental data, such as inconsistent surface coating efficiencies?
Methodological Answer:
- Root-Cause Analysis : Apply decision logic trees to isolate variables (e.g., solvent purity, substrate pretreatment). Prioritize data using environmental fate and physicochemical property databases .
- Statistical Validation : Use multivariate analysis (e.g., ANOVA) to assess the significance of factors like temperature or humidity. Replicate experiments ≥3 times with controls .
特性
InChI |
InChI=1S/C19H40ClSi/c1-3-4-5-6-7-8-9-10-11-12-13-14-15-16-17-18-19-21(2)20/h3-19H2,1-2H3 | |
---|---|---|
Source | PubChem | |
URL | https://pubchem.ncbi.nlm.nih.gov | |
Description | Data deposited in or computed by PubChem | |
InChI Key |
NLHXJCBRDQLLMA-UHFFFAOYSA-N | |
Source | PubChem | |
URL | https://pubchem.ncbi.nlm.nih.gov | |
Description | Data deposited in or computed by PubChem | |
Canonical SMILES |
CCCCCCCCCCCCCCCCCC[Si](C)Cl | |
Source | PubChem | |
URL | https://pubchem.ncbi.nlm.nih.gov | |
Description | Data deposited in or computed by PubChem | |
Molecular Formula |
C19H40ClSi | |
Source | PubChem | |
URL | https://pubchem.ncbi.nlm.nih.gov | |
Description | Data deposited in or computed by PubChem | |
Molecular Weight |
332.1 g/mol | |
Source | PubChem | |
URL | https://pubchem.ncbi.nlm.nih.gov | |
Description | Data deposited in or computed by PubChem | |
試験管内研究製品の免責事項と情報
BenchChemで提示されるすべての記事および製品情報は、情報提供を目的としています。BenchChemで購入可能な製品は、生体外研究のために特別に設計されています。生体外研究は、ラテン語の "in glass" に由来し、生物体の外で行われる実験を指します。これらの製品は医薬品または薬として分類されておらず、FDAから任何の医療状態、病気、または疾患の予防、治療、または治癒のために承認されていません。これらの製品を人間または動物に体内に導入する形態は、法律により厳格に禁止されています。これらのガイドラインに従うことは、研究と実験において法的および倫理的な基準の遵守を確実にするために重要です。