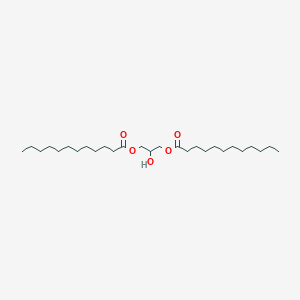
α,α'-ジラウリン
説明
1,3-Dilauroyl-glycerol is a diacylglycerol that contains lauric acid at the sn-1 and sn-3 positions. It has been found in palm-based diacylglycerol oils produced from palm stearin, palm mid fraction, palm oil, and palm olein.
DG(12:0/0:0/12:0), also known as diacylglycerol or diglyceride, belongs to the class of organic compounds known as 1, 3-diacylglycerols. These are diacylglycerols containing a glycerol acylated at positions 1 and 3. DG(12:0/0:0/12:0) is considered to be a practically insoluble (in water) and relatively neutral molecule. Within the cell, DG(12:0/0:0/12:0) is primarily located in the membrane (predicted from logP).
科学的研究の応用
腫瘍学研究
α,α'-ジラウリンは、腫瘍学の分野で研究されています。 α線を放出する放射性核種は、高い線エネルギー付与(LET)を持つα粒子を放出する放射性核種であり、がん治療に適した薬理学的プロファイルを有することが明らかになっています 。これは、α,α'-ジラウリンが、がん治療のための標的α線療法に使用できる可能性を示唆しています。
ナノテクノロジーと医療
核医学におけるナノテクノロジーの応用は、がんを含む様々な疾患の治療において魅力的な治療機会を提供します 。 α,α'-ジラウリンは、ナノ粒子に結合した標的α線療法(TAT)に使用できる可能性があり、これは、α線放出体の高いLETと短い飛程による局所的な細胞死のために理想的です .
薬物送達システム
生体適合性があるため、1,3-ジラウリンは薬物送達システムのキャリアとして研究されています。これは、薬物送達の有効性を高め、副作用を軽減する可能性があります。
抗菌特性
研究によると、1,3-ジラウリンは、特定の細菌や真菌に対して抗菌活性を示す可能性があります。これは、新しい抗菌治療薬の開発に使用できる可能性があります。
バイオ燃料生産における高速熱分解
1,3-ジラウリンは、バイオマスをバイオ燃料に変換するために使用されるプロセスである高速熱分解の文脈で研究されています 。 この研究は、トリグリセリドから脂肪酸、エステル、または炭化水素への変換中のジグリセリド中間体化合物の高速熱分解に焦点を当てています .
プロテオミクス研究
1,3-ジラウリンは、プロテオミクス研究で使用されています 。プロテオミクスは、特に構造と機能に着目したタンパク質の大規模な研究です。この化合物は、タンパク質の構造、機能、および相互作用の研究に使用できる可能性があります。
作用機序
Alpha,Alpha’-Dilaurin, also known as 1,3-Dilauroylglycerol or 1,3-Dilaurin, is a chemical compound with the molecular formula C27H52O5 . This article will discuss its mechanism of action, including its targets, mode of action, biochemical pathways, pharmacokinetics, results of action, and the influence of environmental factors on its action.
Target of Action
It is suggested to be a possible antimicrobial agent against gram-positive organisms .
Mode of Action
It is suggested that it interacts with its targets, possibly microbial cell membranes, leading to their disruption and subsequent antimicrobial effects .
Biochemical Pathways
Given its potential antimicrobial activity, it may interfere with essential biochemical processes in microbial cells, leading to their death .
Pharmacokinetics
Its solubility in acetonitrile and chloroform suggests that it may be absorbed and distributed in the body following oral or dermal administration
Result of Action
The molecular and cellular effects of Alpha,Alpha’-Dilaurin’s action are likely related to its potential antimicrobial activity. It may disrupt the integrity of microbial cell membranes, leading to cell death . .
Action Environment
The action, efficacy, and stability of Alpha,Alpha’-Dilaurin may be influenced by various environmental factors. For instance, its antimicrobial activity may be affected by the presence of other substances, pH, temperature, and the specific strain of microorganism. Its stability may be affected by storage conditions, with a recommended storage temperature of -20°C .
生物活性
1,3-Dilaurin is a diacylglycerol (DAG) characterized by the attachment of two lauric acid chains to the glycerol backbone at the 1 and 3 positions. This unique structure imparts distinct biological activities, particularly in antimicrobial and antiviral contexts. This article explores the biological activity of 1,3-Dilaurin, highlighting its synthesis, mechanisms of action, and applications based on diverse research findings.
1,3-Dilaurin has the chemical formula and a molecular weight of approximately 452.69 g/mol. It is typically synthesized through enzymatic methods involving lipases that catalyze the reaction between glycerol and lauric acid under controlled conditions. Optimal synthesis conditions have been reported to achieve high yields, with studies indicating up to 95.3% lauric acid conversion and 80.3% 1,3-Dilaurin content using specific lipases like Lipozyme RM IM .
Synthesis Parameters
The following table summarizes key parameters for synthesizing 1,3-Dilaurin:
Parameter | Optimal Condition |
---|---|
Temperature | 50°C |
Catalyst | Lipozyme RM IM |
Reaction Time | 3 hours |
Lauric Acid Conversion | Up to 95.3% |
1,3-Dilaurin Content | Up to 80.3% |
Antimicrobial Properties
1,3-Dilaurin exhibits significant antibacterial activity against various pathogens, including Staphylococcus aureus and Escherichia coli . The mechanism of action is believed to involve the disruption of microbial membranes due to its amphiphilic nature, which allows it to integrate into lipid bilayers and compromise membrane integrity .
- Case Study : A study demonstrated that 1,3-Dilaurin enhanced the effectiveness of other antimicrobial agents when used in combination, suggesting its potential as an adjuvant in antimicrobial therapies.
Antiviral Effects
Research has also indicated potential antiviral properties of 1,3-Dilaurin against viruses such as HIV and influenza. The compound's ability to disrupt viral envelopes may contribute to its antiviral efficacy .
- Mechanism : The amphiphilic structure allows for interaction with lipid membranes of viruses, leading to their destabilization and subsequent inactivation .
Comparative Analysis with Similar Compounds
The following table compares 1,3-Dilaurin with other diacylglycerols:
Compound Name | Structure Characteristics | Unique Features |
---|---|---|
1,2-Dilaurin | Lauric acid at positions 1 and 2 | Higher water solubility |
2,3-Dilaurin | Lauric acid at positions 2 and 3 | Optically active antipode |
Monolaurin | Single lauric acid chain | Strong antiviral properties |
Tristearin | Three stearic acid chains | Higher melting point; less soluble |
The unique positioning of lauric acid chains in 1,3-Dilaurin contributes to its distinct biological activities compared to these similar compounds.
Applications
Given its biological properties, 1,3-Dilaurin has potential applications in various fields:
- Pharmaceuticals : As a component in drug delivery systems due to its micelle-forming properties.
- Food Industry : As a natural preservative due to its antimicrobial effects.
- Cosmetics : As an emulsifier or skin-conditioning agent.
特性
IUPAC Name |
(3-dodecanoyloxy-2-hydroxypropyl) dodecanoate | |
---|---|---|
Source | PubChem | |
URL | https://pubchem.ncbi.nlm.nih.gov | |
Description | Data deposited in or computed by PubChem | |
InChI |
InChI=1S/C27H52O5/c1-3-5-7-9-11-13-15-17-19-21-26(29)31-23-25(28)24-32-27(30)22-20-18-16-14-12-10-8-6-4-2/h25,28H,3-24H2,1-2H3 | |
Source | PubChem | |
URL | https://pubchem.ncbi.nlm.nih.gov | |
Description | Data deposited in or computed by PubChem | |
InChI Key |
KUVAEMGNHJQSMH-UHFFFAOYSA-N | |
Source | PubChem | |
URL | https://pubchem.ncbi.nlm.nih.gov | |
Description | Data deposited in or computed by PubChem | |
Canonical SMILES |
CCCCCCCCCCCC(=O)OCC(COC(=O)CCCCCCCCCCC)O | |
Source | PubChem | |
URL | https://pubchem.ncbi.nlm.nih.gov | |
Description | Data deposited in or computed by PubChem | |
Molecular Formula |
C27H52O5 | |
Source | PubChem | |
URL | https://pubchem.ncbi.nlm.nih.gov | |
Description | Data deposited in or computed by PubChem | |
DSSTOX Substance ID |
DTXSID9060237 | |
Record name | Glyceryl 1,3-dilaurate | |
Source | EPA DSSTox | |
URL | https://comptox.epa.gov/dashboard/DTXSID9060237 | |
Description | DSSTox provides a high quality public chemistry resource for supporting improved predictive toxicology. | |
Molecular Weight |
456.7 g/mol | |
Source | PubChem | |
URL | https://pubchem.ncbi.nlm.nih.gov | |
Description | Data deposited in or computed by PubChem | |
Physical Description |
Solid | |
Record name | DG(12:0/0:0/12:0) | |
Source | Human Metabolome Database (HMDB) | |
URL | http://www.hmdb.ca/metabolites/HMDB0093028 | |
Description | The Human Metabolome Database (HMDB) is a freely available electronic database containing detailed information about small molecule metabolites found in the human body. | |
Explanation | HMDB is offered to the public as a freely available resource. Use and re-distribution of the data, in whole or in part, for commercial purposes requires explicit permission of the authors and explicit acknowledgment of the source material (HMDB) and the original publication (see the HMDB citing page). We ask that users who download significant portions of the database cite the HMDB paper in any resulting publications. | |
CAS No. |
539-93-5 | |
Record name | 1,3-Dilaurin | |
Source | CAS Common Chemistry | |
URL | https://commonchemistry.cas.org/detail?cas_rn=539-93-5 | |
Description | CAS Common Chemistry is an open community resource for accessing chemical information. Nearly 500,000 chemical substances from CAS REGISTRY cover areas of community interest, including common and frequently regulated chemicals, and those relevant to high school and undergraduate chemistry classes. This chemical information, curated by our expert scientists, is provided in alignment with our mission as a division of the American Chemical Society. | |
Explanation | The data from CAS Common Chemistry is provided under a CC-BY-NC 4.0 license, unless otherwise stated. | |
Record name | Glyceryl 1,3-dilaurate | |
Source | ChemIDplus | |
URL | https://pubchem.ncbi.nlm.nih.gov/substance/?source=chemidplus&sourceid=0000539935 | |
Description | ChemIDplus is a free, web search system that provides access to the structure and nomenclature authority files used for the identification of chemical substances cited in National Library of Medicine (NLM) databases, including the TOXNET system. | |
Record name | Dodecanoic acid, 1,1'-(2-hydroxy-1,3-propanediyl) ester | |
Source | EPA Chemicals under the TSCA | |
URL | https://www.epa.gov/chemicals-under-tsca | |
Description | EPA Chemicals under the Toxic Substances Control Act (TSCA) collection contains information on chemicals and their regulations under TSCA, including non-confidential content from the TSCA Chemical Substance Inventory and Chemical Data Reporting. | |
Record name | Glyceryl 1,3-dilaurate | |
Source | EPA DSSTox | |
URL | https://comptox.epa.gov/dashboard/DTXSID9060237 | |
Description | DSSTox provides a high quality public chemistry resource for supporting improved predictive toxicology. | |
Record name | 2-hydroxypropane-1,3-diyl dilaurate | |
Source | European Chemicals Agency (ECHA) | |
URL | https://echa.europa.eu/substance-information/-/substanceinfo/100.007.939 | |
Description | The European Chemicals Agency (ECHA) is an agency of the European Union which is the driving force among regulatory authorities in implementing the EU's groundbreaking chemicals legislation for the benefit of human health and the environment as well as for innovation and competitiveness. | |
Explanation | Use of the information, documents and data from the ECHA website is subject to the terms and conditions of this Legal Notice, and subject to other binding limitations provided for under applicable law, the information, documents and data made available on the ECHA website may be reproduced, distributed and/or used, totally or in part, for non-commercial purposes provided that ECHA is acknowledged as the source: "Source: European Chemicals Agency, http://echa.europa.eu/". Such acknowledgement must be included in each copy of the material. ECHA permits and encourages organisations and individuals to create links to the ECHA website under the following cumulative conditions: Links can only be made to webpages that provide a link to the Legal Notice page. | |
Record name | GLYCERYL 1,3-DILAURATE | |
Source | FDA Global Substance Registration System (GSRS) | |
URL | https://gsrs.ncats.nih.gov/ginas/app/beta/substances/D29X1BO64V | |
Description | The FDA Global Substance Registration System (GSRS) enables the efficient and accurate exchange of information on what substances are in regulated products. Instead of relying on names, which vary across regulatory domains, countries, and regions, the GSRS knowledge base makes it possible for substances to be defined by standardized, scientific descriptions. | |
Explanation | Unless otherwise noted, the contents of the FDA website (www.fda.gov), both text and graphics, are not copyrighted. They are in the public domain and may be republished, reprinted and otherwise used freely by anyone without the need to obtain permission from FDA. Credit to the U.S. Food and Drug Administration as the source is appreciated but not required. | |
Retrosynthesis Analysis
AI-Powered Synthesis Planning: Our tool employs the Template_relevance Pistachio, Template_relevance Bkms_metabolic, Template_relevance Pistachio_ringbreaker, Template_relevance Reaxys, Template_relevance Reaxys_biocatalysis model, leveraging a vast database of chemical reactions to predict feasible synthetic routes.
One-Step Synthesis Focus: Specifically designed for one-step synthesis, it provides concise and direct routes for your target compounds, streamlining the synthesis process.
Accurate Predictions: Utilizing the extensive PISTACHIO, BKMS_METABOLIC, PISTACHIO_RINGBREAKER, REAXYS, REAXYS_BIOCATALYSIS database, our tool offers high-accuracy predictions, reflecting the latest in chemical research and data.
Strategy Settings
Precursor scoring | Relevance Heuristic |
---|---|
Min. plausibility | 0.01 |
Model | Template_relevance |
Template Set | Pistachio/Bkms_metabolic/Pistachio_ringbreaker/Reaxys/Reaxys_biocatalysis |
Top-N result to add to graph | 6 |
Feasible Synthetic Routes
試験管内研究製品の免責事項と情報
BenchChemで提示されるすべての記事および製品情報は、情報提供を目的としています。BenchChemで購入可能な製品は、生体外研究のために特別に設計されています。生体外研究は、ラテン語の "in glass" に由来し、生物体の外で行われる実験を指します。これらの製品は医薬品または薬として分類されておらず、FDAから任何の医療状態、病気、または疾患の予防、治療、または治癒のために承認されていません。これらの製品を人間または動物に体内に導入する形態は、法律により厳格に禁止されています。これらのガイドラインに従うことは、研究と実験において法的および倫理的な基準の遵守を確実にするために重要です。