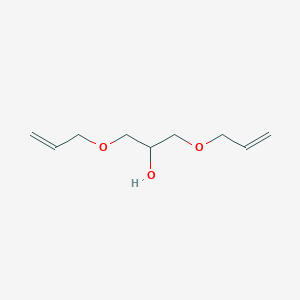
グリセロール α,α'-ジアリルエーテル
説明
Synthesis Analysis
Synthesis of "2-Propanol, 1,3-bis(2-propenyloxy)-" and related compounds involves complex chemical reactions, often aiming to develop materials with specific desirable properties, such as enhanced solubility or improved mechanical strength. For example, the preparation of bis-GMA free dental resin systems through the synthesis of dimethacrylate monomers illustrates the innovation in creating alternatives to conventional materials, highlighting the chemical versatility and potential of such compounds (Mei Yin, Fang Liu, Jingwei He, 2016).
Molecular Structure Analysis
The molecular structure of "2-Propanol, 1,3-bis(2-propenyloxy)-" and its derivatives plays a crucial role in their reactivity and the properties of the materials they compose. Studies on the crystal structures of related compounds provide insight into their molecular arrangements and the potential for engineering materials with tailored features. For instance, the crystal structures of linked-prophyrin precursors and their implications for material design offer valuable information for the development of novel materials (P. G. Jene, J. Ibers, 1999).
Chemical Reactions and Properties
The chemical reactivity of "2-Propanol, 1,3-bis(2-propenyloxy)-" is central to its applications in synthesis and material science. The compound's ability to undergo various chemical reactions enables the development of materials with specific characteristics. Research into the reaction of thiolates with dibromo-1-propanol, for example, demonstrates the compound's versatility in synthesizing hydrophobic analogs of lung surfactant phosphatidylcholines, underscoring its potential in biomedical applications (M. Gernon et al., 1999).
Physical Properties Analysis
The physical properties of "2-Propanol, 1,3-bis(2-propenyloxy)-" and its analogs, such as solubility, glass-transition temperatures, and molecular weight, are critical for their application in various domains, including the development of polymers and resins. For instance, the synthesis and characterization of highly organosoluble poly(ether imide)s based on specific dianhydrides reveal the importance of molecular design in achieving desired physical properties (D. Liaw et al., 2002).
Chemical Properties Analysis
Understanding the chemical properties of "2-Propanol, 1,3-bis(2-propenyloxy)-" is essential for its effective utilization in material science and engineering. The compound's interaction with other chemicals, its stability under various conditions, and its reactivity profile are crucial for synthesizing materials with specific functionalities. The exploration of its redox behavior, as seen in the study of highly conjugated bis(benzo-1,3-dithiole) systems, provides insights into its potential applications in the development of organic metals and other advanced materials (M. Bryce et al., 1990).
科学的研究の応用
液晶配向層
GDAE は液晶配向特性を示します。これは、液晶分子を特定の方向に配向させ、LCD(液晶ディスプレイ)などのディスプレイ技術に不可欠です。GDAE の薄層を適用することで、研究者は液晶配向を正確に制御し、ディスプレイ性能を向上させることができます。
要約すると、グリセロール α,α'-ジアリルエーテルは、高分子化学、光重合、生体医用材料、表面改質、接着剤、および液晶技術において重要な役割を果たします。 その汎用性と独自の特性は、これらの多様な分野における革新的な研究を刺激し続けています . さらに詳しい情報が必要な場合やご質問がある場合は、お気軽にお問い合わせください!
特性
IUPAC Name |
1,3-bis(prop-2-enoxy)propan-2-ol | |
---|---|---|
Source | PubChem | |
URL | https://pubchem.ncbi.nlm.nih.gov | |
Description | Data deposited in or computed by PubChem | |
InChI |
InChI=1S/C9H16O3/c1-3-5-11-7-9(10)8-12-6-4-2/h3-4,9-10H,1-2,5-8H2 | |
Source | PubChem | |
URL | https://pubchem.ncbi.nlm.nih.gov | |
Description | Data deposited in or computed by PubChem | |
InChI Key |
OFLGYLDXUIXHQY-UHFFFAOYSA-N | |
Source | PubChem | |
URL | https://pubchem.ncbi.nlm.nih.gov | |
Description | Data deposited in or computed by PubChem | |
Canonical SMILES |
C=CCOCC(COCC=C)O | |
Source | PubChem | |
URL | https://pubchem.ncbi.nlm.nih.gov | |
Description | Data deposited in or computed by PubChem | |
Molecular Formula |
C9H16O3 | |
Source | PubChem | |
URL | https://pubchem.ncbi.nlm.nih.gov | |
Description | Data deposited in or computed by PubChem | |
DSSTOX Substance ID |
DTXSID2066145 | |
Record name | 2-Propanol, 1,3-bis(2-propenyloxy)- | |
Source | EPA DSSTox | |
URL | https://comptox.epa.gov/dashboard/DTXSID2066145 | |
Description | DSSTox provides a high quality public chemistry resource for supporting improved predictive toxicology. | |
Molecular Weight |
172.22 g/mol | |
Source | PubChem | |
URL | https://pubchem.ncbi.nlm.nih.gov | |
Description | Data deposited in or computed by PubChem | |
CAS RN |
17018-07-4 | |
Record name | 1,3-Bis(2-propen-1-yloxy)-2-propanol | |
Source | CAS Common Chemistry | |
URL | https://commonchemistry.cas.org/detail?cas_rn=17018-07-4 | |
Description | CAS Common Chemistry is an open community resource for accessing chemical information. Nearly 500,000 chemical substances from CAS REGISTRY cover areas of community interest, including common and frequently regulated chemicals, and those relevant to high school and undergraduate chemistry classes. This chemical information, curated by our expert scientists, is provided in alignment with our mission as a division of the American Chemical Society. | |
Explanation | The data from CAS Common Chemistry is provided under a CC-BY-NC 4.0 license, unless otherwise stated. | |
Record name | 2-Propanol, 1,3-bis(2-propen-1-yloxy)- | |
Source | ChemIDplus | |
URL | https://pubchem.ncbi.nlm.nih.gov/substance/?source=chemidplus&sourceid=0017018074 | |
Description | ChemIDplus is a free, web search system that provides access to the structure and nomenclature authority files used for the identification of chemical substances cited in National Library of Medicine (NLM) databases, including the TOXNET system. | |
Record name | 2-Propanol, 1,3-bis(2-propen-1-yloxy)- | |
Source | EPA Chemicals under the TSCA | |
URL | https://www.epa.gov/chemicals-under-tsca | |
Description | EPA Chemicals under the Toxic Substances Control Act (TSCA) collection contains information on chemicals and their regulations under TSCA, including non-confidential content from the TSCA Chemical Substance Inventory and Chemical Data Reporting. | |
Record name | 2-Propanol, 1,3-bis(2-propenyloxy)- | |
Source | EPA DSSTox | |
URL | https://comptox.epa.gov/dashboard/DTXSID2066145 | |
Description | DSSTox provides a high quality public chemistry resource for supporting improved predictive toxicology. | |
Record name | Glycerol alpha,alpha'-Diallyl Ether | |
Source | European Chemicals Agency (ECHA) | |
URL | https://echa.europa.eu/information-on-chemicals | |
Description | The European Chemicals Agency (ECHA) is an agency of the European Union which is the driving force among regulatory authorities in implementing the EU's groundbreaking chemicals legislation for the benefit of human health and the environment as well as for innovation and competitiveness. | |
Explanation | Use of the information, documents and data from the ECHA website is subject to the terms and conditions of this Legal Notice, and subject to other binding limitations provided for under applicable law, the information, documents and data made available on the ECHA website may be reproduced, distributed and/or used, totally or in part, for non-commercial purposes provided that ECHA is acknowledged as the source: "Source: European Chemicals Agency, http://echa.europa.eu/". Such acknowledgement must be included in each copy of the material. ECHA permits and encourages organisations and individuals to create links to the ECHA website under the following cumulative conditions: Links can only be made to webpages that provide a link to the Legal Notice page. | |
試験管内研究製品の免責事項と情報
BenchChemで提示されるすべての記事および製品情報は、情報提供を目的としています。BenchChemで購入可能な製品は、生体外研究のために特別に設計されています。生体外研究は、ラテン語の "in glass" に由来し、生物体の外で行われる実験を指します。これらの製品は医薬品または薬として分類されておらず、FDAから任何の医療状態、病気、または疾患の予防、治療、または治癒のために承認されていません。これらの製品を人間または動物に体内に導入する形態は、法律により厳格に禁止されています。これらのガイドラインに従うことは、研究と実験において法的および倫理的な基準の遵守を確実にするために重要です。