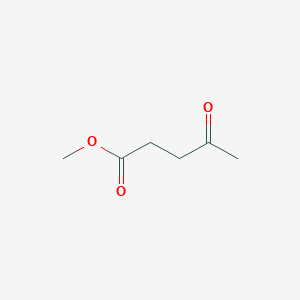
レブリン酸メチル
概要
説明
Methyl levulinate is a short-chain fatty acid ester derived from levulinic acid. It is an important green and high value-added chemical, often produced from biomass. This compound is recognized for its potential as a building block in the synthesis of various chemicals and materials due to its unique chemical structure, which includes both ester and ketone functional groups.
科学的研究の応用
Methyl levulinate has a wide range of applications in scientific research:
Chemistry: It serves as a precursor for the synthesis of various chemicals, including solvents, plasticizers, and pharmaceuticals.
Biology: It is used in the study of metabolic pathways and enzyme reactions.
Industry: It is used in the production of biofuels, biodegradable plastics, and as an additive in lubricants and coatings .
作用機序
Target of Action
Methyl levulinate (ML) is a short-chain fatty acid ester derived from biomass . It is primarily targeted at the conversion of biomass into value-added chemicals . The primary targets of ML are the cellulose components of biomass, which are transformed into ML through a process known as alcoholysis .
Mode of Action
The alcoholysis of cellulose into ML occurs in methanol media, facilitated by several kinds of acid catalysts . One of the synthesized solid niobium-based phosphate catalysts has been found to be highly efficient for the generation of ML . The process involves the interaction of the catalyst with cellulose, leading to the formation of ML. The reaction mechanism is affected by the Brönsted/Lewis acid ratio (known as B/L acid ratio) of solid catalysts .
Biochemical Pathways
The biochemical pathway involved in the formation of ML from cellulose is complex and involves several steps. The alcoholysis of cellulose in methanol leads to the formation of ML . This process is different from the alcoholysis of cellulose in water . The reaction mechanism and type of intermediates of cellulose alcoholysis in methanol are different from those in water .
Pharmacokinetics
Its lower boiling point (around 190 °C) compared to levulinic acid indicates lower energy consumption in separation, which could potentially impact its bioavailability .
Result of Action
The result of the action of ML is the efficient conversion of biomass into a value-added chemical. The yield of ML can be as high as 56%, which is higher than the yield of levulinic acid under the same reaction conditions . The formation of ML is enhanced by Brönsted acid in methanol .
Action Environment
The action of ML is influenced by environmental factors. For instance, the presence of water can reduce the formation of by-products, namely, methyl lactate and 1,1,2-trimethoxyethane . Additionally, the carbon footprint analysis of the supply chain of bio-based ML production shows that the entire life cycle of ML could reduce by approximately 24% of carbon emissions compared with fossil diesel of equal quality . This suggests that the environmental impact of ML production is significantly lower than that of traditional fossil fuels.
将来の方向性
Methyl levulinate is a promising building block which can be derived from cellulosic biomass . This review summarized a variety of process methods for preparing methyl levulinate, pointed out the best reaction conditions and parameters in the current research progress, and explained the key reaction mechanism for methyl levulinate formation . The research directions in this field were prospected .
生化学分析
Biochemical Properties
Methyl levulinate plays a significant role in biochemical reactions. It interacts with various enzymes, proteins, and other biomolecules. For instance, it has been found that a combination of aluminum compounds and organic sulfonic acids can catalyze the synthesis of methyl levulinate from both microcrystalline cellulose and wood powder .
Cellular Effects
It is known that it influences cell function, including impacts on cell signaling pathways, gene expression, and cellular metabolism .
Molecular Mechanism
The molecular mechanism of action of methyl levulinate involves its interactions with biomolecules, enzyme inhibition or activation, and changes in gene expression . It exerts its effects at the molecular level, including binding interactions with biomolecules .
Temporal Effects in Laboratory Settings
In laboratory settings, the effects of methyl levulinate change over time. This includes information on the product’s stability, degradation, and any long-term effects on cellular function observed in in vitro or in vivo studies .
Metabolic Pathways
Methyl levulinate is involved in various metabolic pathways. It interacts with enzymes or cofactors, and it can also affect metabolic flux or metabolite levels .
Transport and Distribution
The transport and distribution of methyl levulinate within cells and tissues are complex processes that involve various transporters or binding proteins
準備方法
Synthetic Routes and Reaction Conditions: Methyl levulinate can be synthesized through the esterification of levulinic acid with methanol. This reaction typically requires an acid catalyst, such as sulfuric acid, to proceed efficiently. The reaction conditions often involve heating the mixture to reflux temperatures to ensure complete conversion.
Industrial Production Methods: On an industrial scale, methyl levulinate is produced from lignocellulosic biomass. The process involves three main steps:
- Hydrolysis of cellulose to produce glucose or 5-hydroxymethylfurfural.
- Dehydration and rearrangement of glucose to form levulinic acid.
- Esterification of levulinic acid with methanol to produce methyl levulinate .
化学反応の分析
Types of Reactions: Methyl levulinate undergoes various chemical reactions, including:
Oxidation: It can be oxidized to produce levulinic acid.
Reduction: It can be reduced to form γ-valerolactone.
Substitution: It can participate in nucleophilic substitution reactions due to the presence of the ester group.
Common Reagents and Conditions:
Oxidation: Common oxidizing agents include potassium permanganate and chromium trioxide.
Reduction: Catalysts such as palladium on carbon or nickel are used in hydrogenation reactions.
Substitution: Nucleophiles like amines or alcohols can react with methyl levulinate under acidic or basic conditions.
Major Products:
Oxidation: Levulinic acid.
Reduction: γ-Valerolactone.
Substitution: Various substituted esters and amides
類似化合物との比較
Methyl levulinate is often compared with other levulinate esters, such as ethyl levulinate and butyl levulinate. These compounds share similar chemical properties but differ in their physical properties and applications. For instance:
Ethyl Levulinate: Has a higher boiling point and is used as a fuel additive.
Butyl Levulinate: Has better solubility in organic solvents and is used in the synthesis of polymers and resins.
Methyl levulinate is unique due to its balance of reactivity and stability, making it a versatile building block in various chemical processes .
特性
IUPAC Name |
methyl 4-oxopentanoate | |
---|---|---|
Source | PubChem | |
URL | https://pubchem.ncbi.nlm.nih.gov | |
Description | Data deposited in or computed by PubChem | |
InChI |
InChI=1S/C6H10O3/c1-5(7)3-4-6(8)9-2/h3-4H2,1-2H3 | |
Source | PubChem | |
URL | https://pubchem.ncbi.nlm.nih.gov | |
Description | Data deposited in or computed by PubChem | |
InChI Key |
UAGJVSRUFNSIHR-UHFFFAOYSA-N | |
Source | PubChem | |
URL | https://pubchem.ncbi.nlm.nih.gov | |
Description | Data deposited in or computed by PubChem | |
Canonical SMILES |
CC(=O)CCC(=O)OC | |
Source | PubChem | |
URL | https://pubchem.ncbi.nlm.nih.gov | |
Description | Data deposited in or computed by PubChem | |
Molecular Formula |
C6H10O3 | |
Source | PubChem | |
URL | https://pubchem.ncbi.nlm.nih.gov | |
Description | Data deposited in or computed by PubChem | |
DSSTOX Substance ID |
DTXSID80211455 | |
Record name | Methyl levulinate | |
Source | EPA DSSTox | |
URL | https://comptox.epa.gov/dashboard/DTXSID80211455 | |
Description | DSSTox provides a high quality public chemistry resource for supporting improved predictive toxicology. | |
Molecular Weight |
130.14 g/mol | |
Source | PubChem | |
URL | https://pubchem.ncbi.nlm.nih.gov | |
Description | Data deposited in or computed by PubChem | |
Physical Description |
Clear colourless liquid; Mild carmellic aroma | |
Record name | Methyl levulinate | |
Source | Joint FAO/WHO Expert Committee on Food Additives (JECFA) | |
URL | https://www.fao.org/food/food-safety-quality/scientific-advice/jecfa/jecfa-flav/details/en/c/1948/ | |
Description | The flavoring agent databse provides the most recent specifications for flavorings evaluated by JECFA. | |
Explanation | Permission from WHO is not required for the use of WHO materials issued under the Creative Commons Attribution-NonCommercial-ShareAlike 3.0 Intergovernmental Organization (CC BY-NC-SA 3.0 IGO) licence. | |
Boiling Point |
196.00 °C. @ 760.00 mm Hg | |
Record name | Methyl levulinate | |
Source | Human Metabolome Database (HMDB) | |
URL | http://www.hmdb.ca/metabolites/HMDB0041602 | |
Description | The Human Metabolome Database (HMDB) is a freely available electronic database containing detailed information about small molecule metabolites found in the human body. | |
Explanation | HMDB is offered to the public as a freely available resource. Use and re-distribution of the data, in whole or in part, for commercial purposes requires explicit permission of the authors and explicit acknowledgment of the source material (HMDB) and the original publication (see the HMDB citing page). We ask that users who download significant portions of the database cite the HMDB paper in any resulting publications. | |
Solubility |
Sparingly soluble in water, Soluble (in ethanol) | |
Record name | Methyl levulinate | |
Source | Joint FAO/WHO Expert Committee on Food Additives (JECFA) | |
URL | https://www.fao.org/food/food-safety-quality/scientific-advice/jecfa/jecfa-flav/details/en/c/1948/ | |
Description | The flavoring agent databse provides the most recent specifications for flavorings evaluated by JECFA. | |
Explanation | Permission from WHO is not required for the use of WHO materials issued under the Creative Commons Attribution-NonCommercial-ShareAlike 3.0 Intergovernmental Organization (CC BY-NC-SA 3.0 IGO) licence. | |
Density |
1.049-1.055 (20°) | |
Record name | Methyl levulinate | |
Source | Joint FAO/WHO Expert Committee on Food Additives (JECFA) | |
URL | https://www.fao.org/food/food-safety-quality/scientific-advice/jecfa/jecfa-flav/details/en/c/1948/ | |
Description | The flavoring agent databse provides the most recent specifications for flavorings evaluated by JECFA. | |
Explanation | Permission from WHO is not required for the use of WHO materials issued under the Creative Commons Attribution-NonCommercial-ShareAlike 3.0 Intergovernmental Organization (CC BY-NC-SA 3.0 IGO) licence. | |
CAS No. |
624-45-3 | |
Record name | Methyl levulinate | |
Source | CAS Common Chemistry | |
URL | https://commonchemistry.cas.org/detail?cas_rn=624-45-3 | |
Description | CAS Common Chemistry is an open community resource for accessing chemical information. Nearly 500,000 chemical substances from CAS REGISTRY cover areas of community interest, including common and frequently regulated chemicals, and those relevant to high school and undergraduate chemistry classes. This chemical information, curated by our expert scientists, is provided in alignment with our mission as a division of the American Chemical Society. | |
Explanation | The data from CAS Common Chemistry is provided under a CC-BY-NC 4.0 license, unless otherwise stated. | |
Record name | Methyl levulinate | |
Source | ChemIDplus | |
URL | https://pubchem.ncbi.nlm.nih.gov/substance/?source=chemidplus&sourceid=0000624453 | |
Description | ChemIDplus is a free, web search system that provides access to the structure and nomenclature authority files used for the identification of chemical substances cited in National Library of Medicine (NLM) databases, including the TOXNET system. | |
Record name | Methyl levulinate | |
Source | DTP/NCI | |
URL | https://dtp.cancer.gov/dtpstandard/servlet/dwindex?searchtype=NSC&outputformat=html&searchlist=24874 | |
Description | The NCI Development Therapeutics Program (DTP) provides services and resources to the academic and private-sector research communities worldwide to facilitate the discovery and development of new cancer therapeutic agents. | |
Explanation | Unless otherwise indicated, all text within NCI products is free of copyright and may be reused without our permission. Credit the National Cancer Institute as the source. | |
Record name | Methyl levulinate | |
Source | EPA DSSTox | |
URL | https://comptox.epa.gov/dashboard/DTXSID80211455 | |
Description | DSSTox provides a high quality public chemistry resource for supporting improved predictive toxicology. | |
Record name | Methyl 4-oxovalerate | |
Source | European Chemicals Agency (ECHA) | |
URL | https://echa.europa.eu/substance-information/-/substanceinfo/100.009.861 | |
Description | The European Chemicals Agency (ECHA) is an agency of the European Union which is the driving force among regulatory authorities in implementing the EU's groundbreaking chemicals legislation for the benefit of human health and the environment as well as for innovation and competitiveness. | |
Explanation | Use of the information, documents and data from the ECHA website is subject to the terms and conditions of this Legal Notice, and subject to other binding limitations provided for under applicable law, the information, documents and data made available on the ECHA website may be reproduced, distributed and/or used, totally or in part, for non-commercial purposes provided that ECHA is acknowledged as the source: "Source: European Chemicals Agency, http://echa.europa.eu/". Such acknowledgement must be included in each copy of the material. ECHA permits and encourages organisations and individuals to create links to the ECHA website under the following cumulative conditions: Links can only be made to webpages that provide a link to the Legal Notice page. | |
Record name | METHYL LEVULINATE | |
Source | FDA Global Substance Registration System (GSRS) | |
URL | https://gsrs.ncats.nih.gov/ginas/app/beta/substances/3Q95S830W6 | |
Description | The FDA Global Substance Registration System (GSRS) enables the efficient and accurate exchange of information on what substances are in regulated products. Instead of relying on names, which vary across regulatory domains, countries, and regions, the GSRS knowledge base makes it possible for substances to be defined by standardized, scientific descriptions. | |
Explanation | Unless otherwise noted, the contents of the FDA website (www.fda.gov), both text and graphics, are not copyrighted. They are in the public domain and may be republished, reprinted and otherwise used freely by anyone without the need to obtain permission from FDA. Credit to the U.S. Food and Drug Administration as the source is appreciated but not required. | |
Record name | Methyl levulinate | |
Source | Human Metabolome Database (HMDB) | |
URL | http://www.hmdb.ca/metabolites/HMDB0041602 | |
Description | The Human Metabolome Database (HMDB) is a freely available electronic database containing detailed information about small molecule metabolites found in the human body. | |
Explanation | HMDB is offered to the public as a freely available resource. Use and re-distribution of the data, in whole or in part, for commercial purposes requires explicit permission of the authors and explicit acknowledgment of the source material (HMDB) and the original publication (see the HMDB citing page). We ask that users who download significant portions of the database cite the HMDB paper in any resulting publications. | |
Synthesis routes and methods I
Procedure details
Synthesis routes and methods II
Procedure details
Synthesis routes and methods III
Procedure details
Synthesis routes and methods IV
Procedure details
Synthesis routes and methods V
Procedure details
Retrosynthesis Analysis
AI-Powered Synthesis Planning: Our tool employs the Template_relevance Pistachio, Template_relevance Bkms_metabolic, Template_relevance Pistachio_ringbreaker, Template_relevance Reaxys, Template_relevance Reaxys_biocatalysis model, leveraging a vast database of chemical reactions to predict feasible synthetic routes.
One-Step Synthesis Focus: Specifically designed for one-step synthesis, it provides concise and direct routes for your target compounds, streamlining the synthesis process.
Accurate Predictions: Utilizing the extensive PISTACHIO, BKMS_METABOLIC, PISTACHIO_RINGBREAKER, REAXYS, REAXYS_BIOCATALYSIS database, our tool offers high-accuracy predictions, reflecting the latest in chemical research and data.
Strategy Settings
Precursor scoring | Relevance Heuristic |
---|---|
Min. plausibility | 0.01 |
Model | Template_relevance |
Template Set | Pistachio/Bkms_metabolic/Pistachio_ringbreaker/Reaxys/Reaxys_biocatalysis |
Top-N result to add to graph | 6 |
Feasible Synthetic Routes
試験管内研究製品の免責事項と情報
BenchChemで提示されるすべての記事および製品情報は、情報提供を目的としています。BenchChemで購入可能な製品は、生体外研究のために特別に設計されています。生体外研究は、ラテン語の "in glass" に由来し、生物体の外で行われる実験を指します。これらの製品は医薬品または薬として分類されておらず、FDAから任何の医療状態、病気、または疾患の予防、治療、または治癒のために承認されていません。これらの製品を人間または動物に体内に導入する形態は、法律により厳格に禁止されています。これらのガイドラインに従うことは、研究と実験において法的および倫理的な基準の遵守を確実にするために重要です。