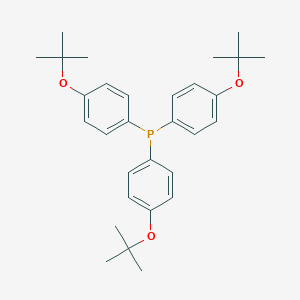
Tris(p-tert-butoxyphenyl)phosphine
- 専門家チームからの見積もりを受け取るには、QUICK INQUIRYをクリックしてください。
- 品質商品を競争力のある価格で提供し、研究に集中できます。
説明
Tris(p-tert-butoxyphenyl)phosphine is a triarylphosphine derivative, a class of organophosphorus compounds known for their interesting structural and electronic properties. These compounds are significant in various chemical reactions and have diverse applications in catalysis and material science.
Synthesis Analysis
The synthesis of triarylphosphines like Tris(p-tert-butoxyphenyl)phosphine generally involves the reaction of aryl groups with phosphorus compounds. For instance, Sasaki et al. (2002) synthesized extremely crowded triarylpnictogens, including triarylphosphines, by reacting 2,4,6-triisopropylphenylcopper(I) with pnictogen trichlorides, demonstrating the influence of bulky aryl groups on the structure and properties of the resulting compounds (Sasaki, Sutoh, Murakami, & Yoshifuji, 2002).
Molecular Structure Analysis
The molecular structure of triarylphosphines like Tris(p-tert-butoxyphenyl)phosphine is influenced significantly by the substituents on the aryl rings. Steinberger, Ziemer, and Meisel (2001) studied tris(tert-butyl)phosphine selenide, revealing how bulky tert-butyl ligands influence bond angles and lengths, indicative of distorted tetrahedral geometry around the phosphorus atom (Steinberger, Ziemer, & Meisel, 2001).
Chemical Reactions and Properties
Triarylphosphines participate in various chemical reactions, often as ligands in coordination complexes. For instance, Burford et al. (2003) synthesized a series of phosphine-diphenylphosphenium donor-acceptor cationic complexes, highlighting the versatility of triarylphosphines in forming homoatomic P-P coordinate bonds (Burford, Ragogna, McDonald, & Ferguson, 2003).
Physical Properties Analysis
The physical properties of triarylphosphines like Tris(p-tert-butoxyphenyl)phosphine are dictated by their molecular structure. The bulky substituents often lead to significant steric hindrance, influencing their solubility, melting points, and crystalline structure.
Chemical Properties Analysis
The chemical properties of triarylphosphines are characterized by their electron-donating abilities and reactivity towards various chemical entities. For example, Hayashi et al. (2007) explored the basicity of Tris(2,4,6-trimethoxyphenyl)phosphine in organic synthesis, demonstrating its utility in the transformation of α,β-unsaturated aldehydes to saturated carboxylic-acid derivatives (Hayashi, Kawabata, Yoshimoto, & Tanaka, 2007).
科学的研究の応用
Phosphine Compounds in Synthesis and Catalysis
Phosphine compounds are pivotal in synthesis and catalysis due to their ability to act as ligands, forming complexes with various metals. These complexes are widely used in catalytic reactions, including hydrogenation, carbon-carbon bond formation, and more. The versatility of phosphine ligands stems from their electronic and steric properties, which can be finely tuned to optimize the activity and selectivity of metal catalysts (Chi & Chou, 2010; Li et al., 2007).
Phosphine Ligands in Organic Synthesis
The structural and electronic flexibility of phosphine ligands makes them invaluable in organic synthesis. They facilitate a range of transformations, including coupling reactions and the synthesis of complex molecules. Their ability to stabilize different metal centers opens the door to numerous catalytic processes essential for constructing organic frameworks (Dutartre et al., 2016).
Environmental Applications
Phosphine compounds also find applications in environmental science, particularly in the study of phosphorus cycles and the detection of phosphine in various matrices. Their role in environmental chemistry highlights the broader impacts of phosphine beyond synthesis and catalysis, including implications for atmospheric chemistry and biogeochemical cycles (Glindemann et al., 2005).
Bioorthogonal Chemistry
Phosphine compounds play a critical role in bioorthogonal chemistry, where they are used for labeling and tracking molecules within biological systems without interfering with natural processes. This application is particularly important in the development of diagnostic and therapeutic tools, showcasing the versatility of phosphine compounds in bridging chemistry and biology (Heiss et al., 2021).
Material Science and Luminescence
In material science, phosphine ligands contribute to the development of luminescent materials. Their ability to form complexes with metals that exhibit photophysical properties is utilized in creating sensors, light-emitting diodes (LEDs), and other photonic devices. This underscores the importance of phosphine compounds in the advancement of materials with novel optical properties (Szukalski et al., 2021).
特性
IUPAC Name |
tris[4-[(2-methylpropan-2-yl)oxy]phenyl]phosphane |
Source
|
---|---|---|
Source | PubChem | |
URL | https://pubchem.ncbi.nlm.nih.gov | |
Description | Data deposited in or computed by PubChem | |
InChI |
InChI=1S/C30H39O3P/c1-28(2,3)31-22-10-16-25(17-11-22)34(26-18-12-23(13-19-26)32-29(4,5)6)27-20-14-24(15-21-27)33-30(7,8)9/h10-21H,1-9H3 |
Source
|
Source | PubChem | |
URL | https://pubchem.ncbi.nlm.nih.gov | |
Description | Data deposited in or computed by PubChem | |
InChI Key |
UGNAOCDIZFIEQK-UHFFFAOYSA-N |
Source
|
Source | PubChem | |
URL | https://pubchem.ncbi.nlm.nih.gov | |
Description | Data deposited in or computed by PubChem | |
Canonical SMILES |
CC(C)(C)OC1=CC=C(C=C1)P(C2=CC=C(C=C2)OC(C)(C)C)C3=CC=C(C=C3)OC(C)(C)C |
Source
|
Source | PubChem | |
URL | https://pubchem.ncbi.nlm.nih.gov | |
Description | Data deposited in or computed by PubChem | |
Molecular Formula |
C30H39O3P |
Source
|
Source | PubChem | |
URL | https://pubchem.ncbi.nlm.nih.gov | |
Description | Data deposited in or computed by PubChem | |
Molecular Weight |
478.6 g/mol |
Source
|
Source | PubChem | |
URL | https://pubchem.ncbi.nlm.nih.gov | |
Description | Data deposited in or computed by PubChem | |
Product Name |
Tris-(p-TerT-buToxyphenyl) phosphine |
試験管内研究製品の免責事項と情報
BenchChemで提示されるすべての記事および製品情報は、情報提供を目的としています。BenchChemで購入可能な製品は、生体外研究のために特別に設計されています。生体外研究は、ラテン語の "in glass" に由来し、生物体の外で行われる実験を指します。これらの製品は医薬品または薬として分類されておらず、FDAから任何の医療状態、病気、または疾患の予防、治療、または治癒のために承認されていません。これらの製品を人間または動物に体内に導入する形態は、法律により厳格に禁止されています。これらのガイドラインに従うことは、研究と実験において法的および倫理的な基準の遵守を確実にするために重要です。