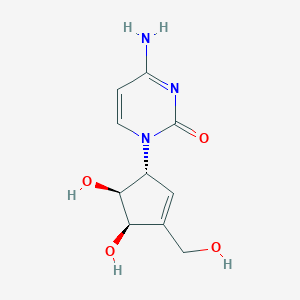
Cyclopentenylcytosine
概要
説明
Cyclopentenylcytosine is a synthetic nucleoside analog that has garnered significant attention due to its antiviral and anticancer properties. It is structurally similar to cytidine, a naturally occurring nucleoside, but features a cyclopentene ring in place of the ribose sugar. This modification imparts unique biological activities to this compound, making it a valuable compound in various scientific research fields.
準備方法
Synthetic Routes and Reaction Conditions: Cyclopentenylcytosine can be synthesized through several synthetic routes. One common method involves the cyclization of a suitable precursor, such as a cytidine derivative, under acidic or basic conditions. The reaction typically requires a catalyst to facilitate the cyclization process. The resulting this compound is then purified using chromatographic techniques to obtain a high-purity product.
Industrial Production Methods: In an industrial setting, the production of this compound involves large-scale synthesis using optimized reaction conditions to maximize yield and minimize impurities. The process may include steps such as solvent extraction, crystallization, and recrystallization to ensure the final product meets stringent quality standards. Advanced analytical techniques, such as high-performance liquid chromatography, are employed to monitor the purity and consistency of the compound.
化学反応の分析
Types of Reactions: Cyclopentenylcytosine undergoes various chemical reactions, including:
Oxidation: The compound can be oxidized to form different oxidation products, depending on the reagents and conditions used.
Reduction: Reduction reactions can convert this compound into its reduced forms, which may exhibit different biological activities.
Substitution: Substitution reactions involve the replacement of specific functional groups within the this compound molecule, leading to the formation of new derivatives with altered properties.
Common Reagents and Conditions:
Oxidation: Common oxidizing agents include potassium permanganate and hydrogen peroxide. The reactions are typically carried out under controlled temperature and pH conditions.
Reduction: Reducing agents such as sodium borohydride and lithium aluminum hydride are used in reduction reactions. These reactions often require anhydrous conditions to prevent unwanted side reactions.
Substitution: Substitution reactions may involve reagents like halogens, alkylating agents, or nucleophiles. The choice of reagent and reaction conditions depends on the desired substitution product.
Major Products Formed: The major products formed from these reactions vary based on the specific reaction conditions and reagents used. For example, oxidation of this compound can yield various oxidized derivatives, while reduction may produce reduced forms with different biological activities. Substitution reactions can lead to the formation of new nucleoside analogs with potential therapeutic applications.
科学的研究の応用
Cyclopentenylcytosine has a wide range of scientific research applications, including:
Chemistry: The compound is used as a building block in the synthesis of novel nucleoside analogs and other bioactive molecules.
Biology: this compound is employed in studies investigating the mechanisms of nucleoside metabolism and the role of nucleosides in cellular processes.
Medicine: The compound has shown promise as an antiviral and anticancer agent. It has demonstrated activity against a variety of viruses and cancer cell lines, making it a potential candidate for therapeutic development.
Industry: this compound is used in the development of new pharmaceuticals and as a research tool in drug discovery and development.
作用機序
The mechanism of action of cyclopentenylcytosine involves the inhibition of cytidine triphosphate synthetase, an enzyme crucial for the synthesis of cytidine triphosphate. By inhibiting this enzyme, this compound disrupts the synthesis of cytidine triphosphate, leading to a depletion of this essential nucleotide. This disruption affects various cellular processes, including DNA and RNA synthesis, ultimately resulting in the inhibition of viral replication and cancer cell proliferation.
類似化合物との比較
Cyclopentenylcytosine is unique among nucleoside analogs due to its cyclopentene ring structure. This structural feature distinguishes it from other nucleoside analogs, such as:
Cidofovir: A nucleoside analog with antiviral activity, but with a different mechanism of action and structural features.
Gemcitabine: An anticancer nucleoside analog that incorporates into DNA and inhibits DNA synthesis.
Cytarabine: A nucleoside analog used in the treatment of leukemia, which also incorporates into DNA and inhibits DNA synthesis.
The unique structure of this compound imparts distinct biological activities, making it a valuable compound for research and therapeutic applications.
特性
IUPAC Name |
4-amino-1-[(1R,4R,5S)-4,5-dihydroxy-3-(hydroxymethyl)cyclopent-2-en-1-yl]pyrimidin-2-one | |
---|---|---|
Source | PubChem | |
URL | https://pubchem.ncbi.nlm.nih.gov | |
Description | Data deposited in or computed by PubChem | |
InChI |
InChI=1S/C10H13N3O4/c11-7-1-2-13(10(17)12-7)6-3-5(4-14)8(15)9(6)16/h1-3,6,8-9,14-16H,4H2,(H2,11,12,17)/t6-,8-,9+/m1/s1 | |
Source | PubChem | |
URL | https://pubchem.ncbi.nlm.nih.gov | |
Description | Data deposited in or computed by PubChem | |
InChI Key |
DUJGMZAICVPCBJ-VDAHYXPESA-N | |
Source | PubChem | |
URL | https://pubchem.ncbi.nlm.nih.gov | |
Description | Data deposited in or computed by PubChem | |
Canonical SMILES |
C1=CN(C(=O)N=C1N)C2C=C(C(C2O)O)CO | |
Source | PubChem | |
URL | https://pubchem.ncbi.nlm.nih.gov | |
Description | Data deposited in or computed by PubChem | |
Isomeric SMILES |
C1=CN(C(=O)N=C1N)[C@@H]2C=C([C@H]([C@H]2O)O)CO | |
Source | PubChem | |
URL | https://pubchem.ncbi.nlm.nih.gov | |
Description | Data deposited in or computed by PubChem | |
Molecular Formula |
C10H13N3O4 | |
Source | PubChem | |
URL | https://pubchem.ncbi.nlm.nih.gov | |
Description | Data deposited in or computed by PubChem | |
DSSTOX Substance ID |
DTXSID70238212 | |
Record name | Cyclopentenyl cytosine | |
Source | EPA DSSTox | |
URL | https://comptox.epa.gov/dashboard/DTXSID70238212 | |
Description | DSSTox provides a high quality public chemistry resource for supporting improved predictive toxicology. | |
Molecular Weight |
239.23 g/mol | |
Source | PubChem | |
URL | https://pubchem.ncbi.nlm.nih.gov | |
Description | Data deposited in or computed by PubChem | |
Solubility |
Water 7.5 (mg/mL), Acetate buffer, pH 4 10.0 (mg/mL), Carbonate buffer, pH 9 7.5 (mg/mL), 0.1 N HCl 15.0 (mg/mL), 0.1 N NaOH 7.5 (mg/mL), Ethanol < 1 (mg/mL), Methanol < 1 (mg/mL), Butanol < 1 (mg/mL), Dimethylacetamide > 30.0 (mg/mL), DMSO > 30.0 (mg/mL), Acetonitrile < 1 (mg/mL), Ethyl acetate < 1 (mg/mL), Chloroform < 1 (mg/mL), Toluene < 1 (mg/mL) | |
Record name | CYCLOPENTENYLCYTOSINE | |
Source | NCI Investigational Drugs | |
URL | http://dtp.nci.nih.gov/NCI-InvestigationalDrugsCI92/375575%20(1992).txt | |
Description | An investigational drug is one that is under study but does not have permission from the U.S. Food and Drug Administration (FDA) to be legally marketed and sold in the United States. NCI provides the investigational drug to the physicians who are participating in clinical trials or TRC protocols. For more information please visit NCI investigational drug website: https://www.cancer.gov/about-cancer/treatment/drugs/investigational-drug-access-fact-sheet | |
CAS No. |
90597-22-1 | |
Record name | Cyclopentenylcytosine | |
Source | CAS Common Chemistry | |
URL | https://commonchemistry.cas.org/detail?cas_rn=90597-22-1 | |
Description | CAS Common Chemistry is an open community resource for accessing chemical information. Nearly 500,000 chemical substances from CAS REGISTRY cover areas of community interest, including common and frequently regulated chemicals, and those relevant to high school and undergraduate chemistry classes. This chemical information, curated by our expert scientists, is provided in alignment with our mission as a division of the American Chemical Society. | |
Explanation | The data from CAS Common Chemistry is provided under a CC-BY-NC 4.0 license, unless otherwise stated. | |
Record name | Cyclopentenyl cytosine | |
Source | ChemIDplus | |
URL | https://pubchem.ncbi.nlm.nih.gov/substance/?source=chemidplus&sourceid=0090597221 | |
Description | ChemIDplus is a free, web search system that provides access to the structure and nomenclature authority files used for the identification of chemical substances cited in National Library of Medicine (NLM) databases, including the TOXNET system. | |
Record name | Cyclopentenylcytosine | |
Source | DTP/NCI | |
URL | https://dtp.cancer.gov/dtpstandard/servlet/dwindex?searchtype=NSC&outputformat=html&searchlist=375575 | |
Description | The NCI Development Therapeutics Program (DTP) provides services and resources to the academic and private-sector research communities worldwide to facilitate the discovery and development of new cancer therapeutic agents. | |
Explanation | Unless otherwise indicated, all text within NCI products is free of copyright and may be reused without our permission. Credit the National Cancer Institute as the source. | |
Record name | Cyclopentenyl cytosine | |
Source | EPA DSSTox | |
URL | https://comptox.epa.gov/dashboard/DTXSID70238212 | |
Description | DSSTox provides a high quality public chemistry resource for supporting improved predictive toxicology. | |
Record name | CYCLOPENTENYL CYTOSINE | |
Source | FDA Global Substance Registration System (GSRS) | |
URL | https://gsrs.ncats.nih.gov/ginas/app/beta/substances/69MO0NDN8K | |
Description | The FDA Global Substance Registration System (GSRS) enables the efficient and accurate exchange of information on what substances are in regulated products. Instead of relying on names, which vary across regulatory domains, countries, and regions, the GSRS knowledge base makes it possible for substances to be defined by standardized, scientific descriptions. | |
Explanation | Unless otherwise noted, the contents of the FDA website (www.fda.gov), both text and graphics, are not copyrighted. They are in the public domain and may be republished, reprinted and otherwise used freely by anyone without the need to obtain permission from FDA. Credit to the U.S. Food and Drug Administration as the source is appreciated but not required. | |
Retrosynthesis Analysis
AI-Powered Synthesis Planning: Our tool employs the Template_relevance Pistachio, Template_relevance Bkms_metabolic, Template_relevance Pistachio_ringbreaker, Template_relevance Reaxys, Template_relevance Reaxys_biocatalysis model, leveraging a vast database of chemical reactions to predict feasible synthetic routes.
One-Step Synthesis Focus: Specifically designed for one-step synthesis, it provides concise and direct routes for your target compounds, streamlining the synthesis process.
Accurate Predictions: Utilizing the extensive PISTACHIO, BKMS_METABOLIC, PISTACHIO_RINGBREAKER, REAXYS, REAXYS_BIOCATALYSIS database, our tool offers high-accuracy predictions, reflecting the latest in chemical research and data.
Strategy Settings
Precursor scoring | Relevance Heuristic |
---|---|
Min. plausibility | 0.01 |
Model | Template_relevance |
Template Set | Pistachio/Bkms_metabolic/Pistachio_ringbreaker/Reaxys/Reaxys_biocatalysis |
Top-N result to add to graph | 6 |
Feasible Synthetic Routes
Q1: How does Cyclopentenylcytosine exert its antitumor and antiviral effects?
A1: this compound's primary mechanism of action is through the inhibition of CTP synthetase. [] Inside the cell, CPEC is phosphorylated to its active metabolite, this compound triphosphate (CPEC-TP). [] CPEC-TP acts as a potent inhibitor of CTP synthetase, the enzyme responsible for converting UTP to CTP. [] This inhibition leads to a depletion of intracellular CTP and dCTP pools, which are essential for DNA and RNA synthesis. [] The depletion of these nucleotides ultimately disrupts cellular replication and transcription processes, leading to antitumor and antiviral effects. []
Q2: What is the significance of CTP depletion caused by this compound?
A2: CTP, alongside dCTP, plays a crucial role in DNA and RNA synthesis. [] Depletion of these nucleotide pools, primarily due to CTP synthetase inhibition by CPEC, hinders these essential cellular processes. [] This disruption is particularly detrimental to rapidly dividing cells, such as cancer cells and virus-infected cells, explaining CPEC's antitumor and antiviral activity. [, ]
Q3: What is the molecular formula and weight of this compound?
A3: Unfortunately, the provided research abstracts do not disclose the specific molecular formula and weight of CPEC. This information is crucial for a comprehensive understanding of the compound's properties. Further investigation into its chemical structure and properties would be beneficial.
Q4: Is there any spectroscopic data available for this compound?
A4: The abstracts provided do not include specific spectroscopic data for CPEC. Such data, including NMR, IR, and mass spectrometry, would be valuable for confirming its structure and studying its interactions with biological targets.
Q5: How is this compound absorbed, distributed, metabolized, and excreted (ADME) in the body?
A5: Studies in nonhuman primates revealed that CPEC is rapidly cleared from plasma, primarily through deamination into the inactive metabolite cyclopentenyluridine (CPE-U). [] This metabolic pathway leads to a considerably higher clearance rate in primates compared to rodents and dogs, which primarily excrete CPEC through the kidneys. [] Less than 20% of the administered dose is excreted unchanged in the urine of primates. [] Further research is necessary to fully understand the ADME profile of CPEC in humans.
Q6: What is the in vitro efficacy of this compound against various viruses?
A6: this compound demonstrates potent in vitro antiviral activity against a broad range of viruses. Studies show promising activity against HSV-1, HSV-2, vaccinia, cytomegalovirus, varicella-zoster virus, influenza virus, vesicular stomatitis virus, Japanese encephalitis virus, and Punta Toro virus. [] Notably, it exhibits activity against both TK+ and TK− strains of HSV-1. [] This broad-spectrum activity highlights its potential as a therapeutic agent against various viral infections.
Q7: Has this compound shown efficacy in any animal models of disease?
A7: Yes, CPEC has demonstrated efficacy in several animal models. In a rabbit model of adenovirus ocular infection, topical application of CPEC effectively reduced viral replication. [, ] It exhibited comparable antiviral activity to cidofovir, a known antiviral agent. [] Additionally, in a rat model of brain tumors, continuous intratumoral infusion of CPEC significantly prolonged survival compared to controls. [] These findings support the potential of CPEC as a treatment for both viral infections and certain cancers.
Q8: Are there any known mechanisms of resistance to this compound?
A8: Research has identified alterations in CTP synthase as a potential mechanism of resistance to CPEC. In a study using murine leukemia L1210 cells, a resistant cell line (L1210/CPEC) exhibited a modified CTP synthase enzyme with a decreased sensitivity to CPEC-TP inhibition. [] These resistant cells also displayed increased CTP synthetase gene expression, leading to elevated CTP and dCTP pools, which could contribute to their decreased sensitivity to CPEC. []
Q9: What strategies are being explored to improve the delivery of this compound to specific targets?
A10: Due to its poor penetration across the blood-brain barrier, direct intratumoral infusion of CPEC has been investigated for treating brain tumors. [] This method allows for localized drug delivery, achieving higher drug concentrations within the tumor while minimizing systemic exposure. [] Further research is needed to explore alternative drug delivery systems, such as nanoparticles or prodrugs, which could improve CPEC's bioavailability to other target tissues.
試験管内研究製品の免責事項と情報
BenchChemで提示されるすべての記事および製品情報は、情報提供を目的としています。BenchChemで購入可能な製品は、生体外研究のために特別に設計されています。生体外研究は、ラテン語の "in glass" に由来し、生物体の外で行われる実験を指します。これらの製品は医薬品または薬として分類されておらず、FDAから任何の医療状態、病気、または疾患の予防、治療、または治癒のために承認されていません。これらの製品を人間または動物に体内に導入する形態は、法律により厳格に禁止されています。これらのガイドラインに従うことは、研究と実験において法的および倫理的な基準の遵守を確実にするために重要です。