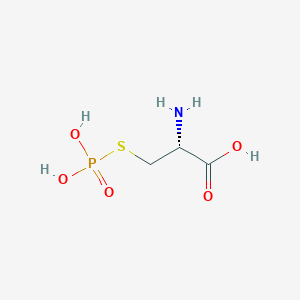
S-Phosphocysteine
- 専門家チームからの見積もりを受け取るには、QUICK INQUIRYをクリックしてください。
- 品質商品を競争力のある価格で提供し、研究に集中できます。
概要
説明
S-ホスホシステイン: は、アミノ酸システインのリン酸化誘導体です。L-システイン-S-コンジュゲートとして知られる有機化合物のクラスに属する小さな分子です。この化合物は、システインの硫黄原子に結合したチオリン酸基の存在によって特徴付けられます。S-ホスホシステインは、翻訳後修飾によるタンパク質機能の調節において、特にさまざまな生物学的プロセスにおいて重要な役割を果たします。
準備方法
合成経路と反応条件: : S-ホスホシステインの合成は、通常、システイン残基のリン酸化を伴います。一般的な方法の1つは、ジスルフィド架橋タンパク質の還元、続いてシステインの(3-アクリロイルアミドプロピル)トリメチルアンモニウムクロリド(APTA)によるアルキル化です。続いて、水酸化バリウム水溶液中でベータ脱離を行い、脱ヒドロアラニン残基を含む修飾されたポリペプチドを得ます。 これらの残基へのチオリン酸の共役付加により、S-ホスホシステインが生成されます .
工業的生産方法: : S-ホスホシステインの具体的な工業的生産方法は広く文書化されていませんが、一般的なアプローチは、前述の化学反応を使用して大規模合成を行うことです。このプロセスは、化学工学およびバイオプロセスの高度な技術を使用して、より高い収率と純度を実現するために最適化される可能性があります。
化学反応の分析
反応の種類: : S-ホスホシステインは、次のようなさまざまな化学反応を起こします。
酸化: チオリン酸基は、特定の条件下で酸化される可能性があります。
還元: 還元反応は、チオリン酸基の硫黄原子を標的にすることができます。
置換: チオリン酸基は、他の官能基に置き換えられる置換反応に関与することができます。
一般的な試薬と条件
酸化: 過酸化水素またはその他の酸化剤。
還元: ジチオスレイトール(DTT)などの還元剤。
置換: 置換反応には、さまざまな求核剤を使用できます。
主要な生成物: : これらの反応から生成される主要な生成物は、使用される特定の試薬と条件によって異なります。 たとえば、酸化はスルホン酸誘導体を生成する可能性がありますが、置換反応はさまざまなチオリン酸含有化合物を生成する可能性があります .
科学研究への応用
S-ホスホシステインには、次のようないくつかの科学研究への応用があります。
化学: リン酸化とそのタンパク質の構造と機能への影響を研究するためのモデル化合物として使用されます。
生物学: タンパク質の翻訳後修飾を通じて、細胞プロセスの調節に役割を果たします。
医学: タンパク質リン酸化が調節不全になっている病気における潜在的な治療的用途について調査されています。
科学的研究の応用
S-Phosphocysteine has several scientific research applications, including:
Chemistry: Used as a model compound to study phosphorylation and its effects on protein structure and function.
Biology: Plays a role in the regulation of cellular processes through post-translational modifications of proteins.
Medicine: Investigated for its potential therapeutic applications in diseases where protein phosphorylation is dysregulated.
作用機序
S-ホスホシステインの作用機序は、タンパク質における翻訳後修飾としての役割を含みます。これは、流入する糖基質のリン酸化を触媒する、ホスホエノールピルビン酸依存性糖リン酸転移酵素系の一部です。この修飾は、タンパク質の活性、安定性、相互作用を変化させる可能性があり、それによりさまざまな細胞プロセスが調節されます。 分子標的は、代謝経路に関与する酵素やトランスポーターです .
類似化合物の比較
類似化合物
- O-ホスホセリン
- O-ホスホスレオニン
- O-ホスホチロシン
- N1-ホスホトリプトファン
- O-ホスホヒドロキシリシン
- O-ホスホヒドロキシプロリン
比較: : S-ホスホシステインは、システインの硫黄原子に結合したチオリン酸基の存在により、ユニークです。一方、他のリン酸化アミノ酸は、通常、酸素原子または窒素原子に結合したリン酸基を持っています。 このユニークな構造により、S-ホスホシステインに独自の化学的特性と生物学的機能が与えられ、タンパク質リン酸化とその影響を研究するための貴重な化合物となっています .
類似化合物との比較
Similar Compounds
- O-Phosphoserine
- O-Phosphothreonine
- O-Phosphotyrosine
- N1-Phosphotryptophan
- O-Phosphohydroxylysine
- O-Phosphohydroxyproline
Comparison: : S-Phosphocysteine is unique due to the presence of a thiophosphate group attached to the sulfur atom of cysteine, whereas other phosphorylated amino acids typically have phosphate groups attached to oxygen or nitrogen atoms. This unique structure imparts distinct chemical properties and biological functions to this compound, making it a valuable compound for studying protein phosphorylation and its effects .
生物活性
S-Phosphocysteine (pCys) is an important post-translational modification that plays significant roles in various biological processes, particularly in signaling pathways and enzyme catalysis. This article reviews the biological activity of this compound, focusing on its mechanisms, physiological implications, and recent research findings.
Overview of this compound
This compound is formed when a phosphate group is covalently attached to the sulfur atom of cysteine residues in proteins. This modification can influence protein function, stability, and interactions, making it a critical component in cellular signaling and metabolic pathways. The formation of this compound often occurs through enzyme-catalyzed reactions, particularly in the context of phosphoenolpyruvate (PEP)-dependent systems, where it acts as a transient intermediate in carbohydrate transport processes .
- Enzyme-Catalyzed Reactions :
- Signal Transduction :
- Protein Stability and Function :
Biological Significance
This compound plays a critical role in several physiological processes:
- Metabolic Regulation : It is involved in regulating metabolic pathways by modulating enzyme activities that are essential for energy production and nutrient transport.
- Cellular Signaling : As a reversible modification, this compound can act as a molecular switch, toggling between active and inactive states in response to cellular signals.
- Disease Implications : Dysregulation of this compound levels has been linked to various diseases, including cancer, where it may influence tumor growth and metastasis through altered signaling pathways .
Research Findings
Recent studies have provided insights into the biological activity of this compound:
- A study demonstrated that phosphorylation on cysteine residues is more prevalent than previously recognized, suggesting its significant role in regulating gene expression and bacterial virulence factors .
- Investigations into cysteine phosphorylation methodologies have revealed advanced techniques for detecting S-phosphorylated peptides using mass spectrometry, enhancing our understanding of its biological implications .
Case Studies
- Cystic Fibrosis Transmembrane Conductance Regulator (CFTR) :
- PRL-CNNM Pathway :
Data Table: Summary of Key Findings on this compound
特性
CAS番号 |
115562-30-6 |
---|---|
分子式 |
C3H8NO5PS |
分子量 |
201.14 g/mol |
IUPAC名 |
(2R)-2-amino-3-phosphonosulfanylpropanoic acid |
InChI |
InChI=1S/C3H8NO5PS/c4-2(3(5)6)1-11-10(7,8)9/h2H,1,4H2,(H,5,6)(H2,7,8,9)/t2-/m0/s1 |
InChIキー |
MNEMQJJMDDZXRO-REOHCLBHSA-N |
SMILES |
C(C(C(=O)O)N)SP(=O)(O)O |
異性体SMILES |
C([C@@H](C(=O)O)N)SP(=O)(O)O |
正規SMILES |
C(C(C(=O)O)N)SP(=O)(O)O |
Key on ui other cas no. |
115562-30-6 |
同義語 |
S-phosphocysteine |
製品の起源 |
United States |
Retrosynthesis Analysis
AI-Powered Synthesis Planning: Our tool employs the Template_relevance Pistachio, Template_relevance Bkms_metabolic, Template_relevance Pistachio_ringbreaker, Template_relevance Reaxys, Template_relevance Reaxys_biocatalysis model, leveraging a vast database of chemical reactions to predict feasible synthetic routes.
One-Step Synthesis Focus: Specifically designed for one-step synthesis, it provides concise and direct routes for your target compounds, streamlining the synthesis process.
Accurate Predictions: Utilizing the extensive PISTACHIO, BKMS_METABOLIC, PISTACHIO_RINGBREAKER, REAXYS, REAXYS_BIOCATALYSIS database, our tool offers high-accuracy predictions, reflecting the latest in chemical research and data.
Strategy Settings
Precursor scoring | Relevance Heuristic |
---|---|
Min. plausibility | 0.01 |
Model | Template_relevance |
Template Set | Pistachio/Bkms_metabolic/Pistachio_ringbreaker/Reaxys/Reaxys_biocatalysis |
Top-N result to add to graph | 6 |
Feasible Synthetic Routes
試験管内研究製品の免責事項と情報
BenchChemで提示されるすべての記事および製品情報は、情報提供を目的としています。BenchChemで購入可能な製品は、生体外研究のために特別に設計されています。生体外研究は、ラテン語の "in glass" に由来し、生物体の外で行われる実験を指します。これらの製品は医薬品または薬として分類されておらず、FDAから任何の医療状態、病気、または疾患の予防、治療、または治癒のために承認されていません。これらの製品を人間または動物に体内に導入する形態は、法律により厳格に禁止されています。これらのガイドラインに従うことは、研究と実験において法的および倫理的な基準の遵守を確実にするために重要です。