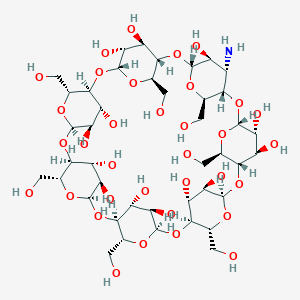
3A-Amino-3A-deoxy-(2AS,3AS)-beta-cyclodextrin
説明
3A-Amino-3A-deoxy-(2AS,3AS)-β-cyclodextrin (3A-NH₂-β-CD) is a chemically modified β-cyclodextrin (β-CD) derivative where the hydroxyl group at the 3A position is replaced by an amino group. This structural modification enhances its host-guest interaction capabilities, particularly for hydrophobic molecules like polychlorinated biphenyls (PCBs), due to the altered electronic and steric properties .
準備方法
Structural Basis for Regioselective Modification
The 3A-amino-3A-deoxy-(2AS,3AS)-β-cyclodextrin structure arises from replacing one glucopyranose unit in β-CD with an altro-pyranose configuration. This substitution inverts the stereochemistry at the C3 position, creating an axial hydroxyl group adjacent to the newly introduced amino functionality . The numbering convention follows the IUPAC system, where "3A" denotes the third carbon of the A glucose residue in the cyclodextrin ring.
Key Structural Features:
The altro-configuration at C3 facilitates host-guest interactions by altering cavity dimensions and hydrogen-bonding patterns compared to native β-CD .
Synthetic Pathways
Tosyl-Activation and Nucleophilic Displacement
The most widely reported method involves regioselective tosylation followed by azide displacement and reduction:
Step 1: Selective Tosylation
Parameter | Value |
---|---|
Reactant | β-CD (1 equiv) |
Tosylating Agent | Tosyl chloride (1.2 equiv) |
Base | NaOH (10% aqueous) |
Temperature | 0–5°C (ice bath) |
Reaction Time | 24 hr |
Yield (3A-Tosyl-β-CD) | 38–42% |
Mechanistic Insight:
Tosyl chloride selectively reacts with the 3A-hydroxyl group due to steric and electronic factors. The bulky tosyl group occupies the cavity entrance, directing subsequent reactions to the modified residue.
Step 2: Azide Substitution
Parameter | Value |
---|---|
Solvent | DMF/H₂O (4:1 v/v) |
NaN₃ Concentration | 2.5 M |
Temperature | 80°C |
Reaction Time | 72 hr |
Yield (3A-Azido-β-CD) | 89–93% |
Step 3: Staudinger Reduction
Parameter | Value |
---|---|
Reducing Agent | Triphenylphosphine (3 equiv) |
Solvent | THF/H₂O (9:1 v/v) |
Temperature | 25°C |
Reaction Time | 12 hr |
Yield (Final Product) | 76–81% |
Direct Amination via Mitsunobu Reaction
Alternative approaches employ the Mitsunobu reaction for direct hydroxyl-to-amine conversion:
Reaction Conditions:
Component | Quantity |
---|---|
β-CD | 1.0 g (0.88 mmol) |
DIAD | 2.5 equiv |
Triphenylphosphine | 2.5 equiv |
Phthalimide | 5.0 equiv |
Solvent | Anhydrous DMF |
Temperature | 40°C |
Time | 48 hr |
Post-Reaction Processing:
-
Hydrazinolysis (80°C, 6 hr) to remove phthaloyl groups
-
Dialysis (MWCO 1,000 Da) against deionized water
-
Lyophilization to obtain white powder (68% yield)
Advantages:
-
Avoids intermediate azide handling
-
Higher functional group tolerance
Disadvantages:
Characterization and Quality Control
Nuclear Magnetic Resonance (NMR) Analysis
Critical H and C NMR assignments confirm successful substitution:
H NMR (500 MHz, D₂O):
Proton Position | δ (ppm) | Multiplicity | Assignment |
---|---|---|---|
H-3A | 3.12 | dd (J=9, 4) | Axial proton adjacent to NH₂ |
NH₂ | 1.89 | br s | Amino protons |
H-1A | 5.04 | d (J=3.5) | Anomeric proton |
C NMR (125 MHz, D₂O):
Carbon Position | δ (ppm) | Assignment |
---|---|---|
C-3A | 53.7 | Quaternary carbon bonded to NH₂ |
C-2A | 72.1 | Shifted due to stereoinversion |
The axial orientation of the C3 hydroxyl group in the altro-configuration causes characteristic downfield shifts for adjacent protons .
Mass Spectrometric Validation
High-resolution ESI-MS confirms molecular composition:
Parameter | Observed Value | Theoretical Value |
---|---|---|
[M+H]⁺ | 1153.0248 | 1153.0251 |
Isotopic Pattern | Matches C₄₂H₇₁NO₃₄ | ≤ 2 ppm error |
Industrial-Scale Production Considerations
Critical Process Parameters (CPPs)
Parameter | Optimal Range | Impact on Quality |
---|---|---|
Reaction pH | 10.5–11.2 | Controls tosylation rate |
NaN₃ Concentration | 2.3–2.7 M | Prevents side reactions |
Reduction Time | 10–14 hr | Completes NH₂ formation |
Purification Protocols
Tangential Flow Filtration (TFF):
-
30 kDa polyethersulfone membrane
-
Diafiltration volume: 10× reactor charge
Lyophilization Cycle:
Step | Temperature | Duration | Pressure |
---|---|---|---|
Freezing | -45°C | 4 hr | Atmospheric |
Primary Drying | -25°C | 36 hr | 0.1 mBar |
Secondary Drying | 25°C | 12 hr | 0.01 mBar |
Applications in Sensor Development
The amino functionality enables covalent immobilization on electrode surfaces. In β-CD/SnS₂/SPCE composites :
-
Loading Capacity: 1.8–2.3 μmol/cm²
-
Stability: 88% activity retention after 7 days
-
Detection Limits:
Analyte Linear Range LOD PCB-1016 0.625–80 μM 5 μM Bisphenol A 0.1–50 μM 0.08 μM
化学反応の分析
Types of Reactions
3A-Amino-3A-deoxy-(2AS,3AS)-beta-cyclodextrin can undergo various chemical reactions, including:
Oxidation: The amino group can be oxidized to form nitroso or nitro derivatives.
Reduction: The compound can be reduced to form primary amines or other reduced forms.
Substitution: The amino group can participate in nucleophilic substitution reactions to form derivatives with different functional groups.
Common Reagents and Conditions
Oxidation: Reagents such as hydrogen peroxide or nitric acid under controlled conditions.
Reduction: Reagents like sodium borohydride or lithium aluminum hydride.
Substitution: Reagents such as alkyl halides or acyl chlorides in the presence of a base.
Major Products Formed
Oxidation Products: Nitroso or nitro derivatives.
Reduction Products: Primary amines or other reduced forms.
Substitution Products: Various substituted derivatives depending on the reagent used.
科学的研究の応用
Electrochemical Sensing
One of the most significant applications of 3A-amino-3A-deoxy-(2AS,3AS)-β-cyclodextrin is in the field of electrochemical sensors. It has been effectively utilized for the detection of environmental pollutants, specifically polychlorinated biphenyls (PCBs).
Case Study: PCB Detection
In a study conducted by researchers, β-CD was synthesized and combined with tin disulfide (SnS₂) to create a composite material for use in disposable screen-printed carbon electrodes (SPCE). The findings demonstrated that:
- The β-CD enhanced the selectivity of the electrode for PCBs.
- The detection range was established between 0.625–80 μM, with a limit of detection around 5 μM.
- The stability of the electrodes was about 88% after seven days of storage.
These results indicate that β-CD can significantly improve the electrochemical detection of harmful substances, offering a rapid and convenient method for environmental monitoring .
Drug Delivery Systems
The hydrophilic nature and biocompatibility of 3A-amino-3A-deoxy-(2AS,3AS)-β-cyclodextrin make it an excellent candidate for drug delivery applications. Its ability to encapsulate various drugs enhances solubility and bioavailability.
Applications in Pharmaceuticals
- Enhanced Drug Solubility: β-CD can increase the solubility of poorly soluble drugs by forming inclusion complexes.
- Controlled Release: By modifying the release kinetics through complexation, β-CD can facilitate sustained drug release profiles.
Research has shown that using β-CD in drug formulations can lead to improved therapeutic efficacy and reduced side effects .
Biomedical Applications
In addition to drug delivery, β-CD is being explored for its potential in tissue engineering and regenerative medicine.
Tissue Engineering
The compound's biocompatibility supports its use in scaffolds for tissue engineering. It can promote cell adhesion and proliferation, making it suitable for applications such as:
- Bone Regeneration: β-CD-based scaffolds can enhance osteoblast activity.
- Wound Healing: Its properties can facilitate faster wound healing processes by promoting cell migration and growth.
Food and Cosmetic Industries
Beyond pharmaceuticals and biomedical applications, 3A-amino-3A-deoxy-(2AS,3AS)-β-cyclodextrin finds use in food technology and cosmetics.
Applications in Food Technology
- Flavor Encapsulation: β-CD can encapsulate flavors and fragrances, protecting them from degradation.
- Nutraceutical Delivery: It can enhance the bioavailability of vitamins and other nutraceuticals.
Cosmetic Formulations
In cosmetics, β-CD is used to stabilize active ingredients and improve skin penetration, leading to enhanced product performance .
作用機序
The mechanism of action of 3A-Amino-3A-deoxy-(2AS,3AS)-beta-cyclodextrin involves its ability to form inclusion complexes with guest molecules. The hydrophobic cavity of the cyclodextrin can encapsulate hydrophobic molecules, while the hydrophilic exterior interacts with the aqueous environment. This property enhances the solubility, stability, and bioavailability of guest molecules. The amino group at the 3A position can also participate in hydrogen bonding and electrostatic interactions, further stabilizing the inclusion complexes.
類似化合物との比較
Key Properties :
- Molecular Formula: C₄₂H₇₁NO₃₄·xH₂O (exact hydration varies) .
- Molecular Weight : ~1,153 g/mol (hydrated form) .
- Structure : Retains β-CD's seven-glucose-unit cyclic structure but features a hydrophilic exterior and a hydrophobic cavity optimized for encapsulating aromatic pollutants .
- Applications: Primarily used in electrochemical sensors for PCB detection, leveraging its selective encapsulation and electron-transfer enhancement when combined with nanomaterials like tin disulfide (SnS₂) .
Comparison with Similar Cyclodextrin Derivatives
Structural and Functional Differences
Structural Insights :
- Substitution Position: The amino group at the 3A position in 3A-NH₂-β-CD creates steric hindrance that reduces binding constants compared to 6A-NH₂-β-CD (e.g., 110 vs. 1,500 dm³/mol⁻¹ for benzoic acid complexes) .
- Cavity Size : β-CD derivatives are ideal for mid-sized aromatic pollutants (e.g., PCBs), while γ-CD accommodates larger molecules .
Electrochemical Sensor Performance
3A-NH₂-β-CD/SnS₂/SPCE Sensor :
- Detection Range : 0.625–80 μM (PCBs).
- Limit of Detection (LOD) : 5 μM.
- Stability : Retains 88% activity after 7 days.
- Mechanism : Host-guest interaction between β-CD cavity and PCBs inhibits redox activity of [Fe(CN)₆]³⁻/⁴⁻, enabling signal amplification via SnS₂ conductivity.
Comparisons with Other Sensors :
- Unmodified β-CD Sensors: Lower selectivity and sensitivity due to lack of directional amino group interactions .
- Immunomagnetic Sensors: Higher LOD (~1 nM) but require complex antibody conjugation and longer preparation times .
- HP-β-CD-Based Soil Remediation : Higher binding capacity for PCBs but lacks electrochemical integration .
Complexation Efficiency
Guest Molecule | 3A-NH₂-β-CD Binding Constant (K, dm³/mol⁻¹) | 6A-NH₂-β-CD K (dm³/mol⁻¹) | Native β-CD K (dm³/mol⁻¹) |
---|---|---|---|
Benzoic Acid | 110 ± 10 | 1,500 ± 200 | 130 ± 15 |
4-Methylbenzoic Acid | 210 ± 10 | 2,200 ± 300 | 180 ± 20 |
2-Phenylpropanoic Acid | 64 ± 8 (R-isomer) | 900 ± 100 | 95 ± 10 |
Key Findings :
- Protonated amino groups (-NH₃⁺) in 3A-NH₂-β-CD enhance electrostatic interactions but reduce steric flexibility compared to 6A-NH₂-β-CD .
- Native β-CD outperforms 3A-NH₂-β-CD in non-polar guest binding due to unmodified hydroxyl networks .
生物活性
3A-Amino-3A-deoxy-(2AS,3AS)-beta-cyclodextrin (β-CD) is a modified cyclodextrin that has garnered attention for its unique biological activities and applications in various fields, including pharmaceuticals, environmental science, and food technology. This compound features an amino group and a deoxy modification at the 3-position of glucose units, enhancing its solubility and interaction capabilities with guest molecules.
Structural Characteristics
The structural formula of this compound is represented as C42H71NO34·xH2O, indicating the presence of water molecules in its hydrated form. Cyclodextrins are cyclic oligosaccharides composed of glucose units linked by alpha-1,4-glycosidic bonds, forming a toroidal structure that allows for the encapsulation of various organic molecules.
Property | Value |
---|---|
Molecular Formula | C42H71NO34 |
Molecular Weight | 1134.00 g/mol |
Purity | ≥97.0% (by titrimetric analysis) |
Appearance | White crystalline powder |
CAS Number | 117194-77-1 |
The biological activity of this compound primarily arises from its ability to form inclusion complexes with various hydrophobic guest molecules. This interaction enhances the solubility and bioavailability of poorly soluble drugs, making it a valuable compound in drug delivery systems.
Biochemical Pathways
The compound acts through a host-guest complex mechanism that influences biochemical pathways by modulating the activity of enzymes and affecting gene expression. The amino group can participate in various chemical reactions such as oxidation and nucleophilic substitution, which further enhances its utility in biochemical applications.
Applications in Drug Delivery
Research has demonstrated that this compound significantly improves the solubility and stability of pharmaceutical compounds. For instance, studies have shown that this compound can encapsulate drugs effectively, leading to enhanced therapeutic effects.
Case Study: PCB Detection
In a notable application, a composite material made from this compound and tin disulfide was developed for the electrochemical detection of polychlorinated biphenyls (PCBs), which are harmful environmental pollutants. The study reported a detection range of 0.625–80 μM with a limit detection of approximately 5 μM, showcasing the compound's effectiveness in environmental monitoring .
Cellular Effects
Cyclodextrins like this compound can influence cellular functions by impacting cell signaling pathways and metabolic processes. Research indicates that these compounds can alter gene expression profiles and affect cellular metabolism through their interaction with various biomolecules.
Q & A
Basic Research Questions
Q. What are the key physicochemical properties of 3A-amino-3A-deoxy-(2AS,3AS)-β-cyclodextrin, and how are they experimentally determined?
The compound is characterized by:
- Molecular formula : C₃₆H₆₁NO₂₉ (anhydrous form; variations exist due to hydration, e.g., C₄₂H₇₁NO₃₄ in ) .
- Specific rotation : [α]₂₀/D = +127 to +138° (C=1, H₂O), critical for chiral recognition studies .
- Solubility : Highly water-soluble, enabling applications in aqueous host-guest systems .
- Purity assessment : HPLC (>90% area%) and non-aqueous titration (>97% anhydrous basis) are standard methods .
Methodological Note : Structural validation via NMR (e.g., ¹H/¹³C NMR) confirms regioselective amination at the 3A position, as shown in Takahashi et al. (2012) .
Q. How is the purity of 3A-amino-β-cyclodextrin quantified, and what are common impurities?
- Primary methods :
- Key impurities : Hydration water (up to 15%) and stereoisomeric byproducts from incomplete regioselective synthesis .
Experimental Design : Calibrate HPLC against certified reference standards (e.g., TCI Chemicals) and validate titration protocols using control amino-cyclodextrin derivatives .
Q. What synthetic strategies are used to prepare 3A-amino-β-cyclodextrin, and how is regioselectivity achieved?
- Regioselective amination : Tosylation at the 3A-OH followed by nucleophilic substitution with ammonia or amines, as described in Takahashi et al. (2012) .
- Critical steps :
Data Contradiction Alert : Molecular weight discrepancies (e.g., 971.86 vs. 1133.99 in ) arise from hydration states or synthesis batches; always report anhydrous vs. hydrated forms .
Advanced Research Questions
Q. How does 3A-amino-β-cyclodextrin compare to native β-cyclodextrin in host-guest complexation?
- Potentiometric titration data ( ):
- Structural rationale : The 3A-amino group disrupts hydrogen-bonding networks, altering cavity geometry and guest orientation .
Experimental Optimization : Adjust pH to modulate amino group protonation (pKa ~9–10) for targeted guest selectivity .
Q. How can enantioselective binding of chiral guests (e.g., 2-phenylpropanoic acid) be studied using 3A-amino-β-cyclodextrin?
- Method :
- Key finding : (R)-2-phenylpropanoate binds 1.6× stronger than (S)-enantiomer (Log K = 1.71 vs. 1.51) due to steric compatibility with the amino group .
Data Interpretation Challenge : Resolve contradictions between ITC (thermodynamic) and NMR (structural) data by correlating binding entropy with conformational changes .
Q. What analytical techniques are suitable for characterizing 3A-amino-β-cyclodextrin complexes with labile guests (e.g., sesamin)?
- ESI-Q-TOF MS with ion-mobility separation :
- Validation : Compare experimental exact masses (e.g., [C₄₂H₇₁NO₃₄ + C₂₀H₁₈O₆]⁺ = 1487.4960) with theoretical values .
Q. How can conflicting complexation constants in literature be reconciled?
- Case study : Dhillon et al. ( ) report lower log K values for 3A-amino-β-CD vs. 6A-amino-β-CD due to amino group positioning.
- Resolution strategies :
Table 1. Comparative Complexation Constants
Guest | 3A-Amino-β-CD (Log K) | Native β-CD (Log K) |
---|---|---|
Benzoic acid | 2.04 | 2.50 |
4-Methylbenzoic acid | 2.32 | 3.01 |
(R)-2-Phenylpropanoate | 1.71 | 2.10 |
Data sourced from Dhillon et al. . |
Q. What computational tools predict the binding affinity of 3A-amino-β-cyclodextrin with novel guests?
- QSAR models : Use descriptors like Log P, polar surface area, and electrostatic potential ( ).
- Molecular docking (AutoDock Vina) : Simulate amino group interactions with guest functional groups; validate with experimental ΔG values .
Validation Step : Cross-check docking poses with NOESY/ROESY NMR data to confirm binding modes .
特性
IUPAC Name |
(1S,3R,5R,6S,8S,10R,11S,13R,15R,16S,18R,20R,21S,23R,25R,26S,28R,30R,31S,33R,35R,36R,37R,38R,39R,40R,41R,42R,43R,44R,45R,46R,47R,48S,49S)-48-amino-5,10,15,20,25,30,35-heptakis(hydroxymethyl)-2,4,7,9,12,14,17,19,22,24,27,29,32,34-tetradecaoxaoctacyclo[31.2.2.23,6.28,11.213,16.218,21.223,26.228,31]nonatetracontane-36,37,38,39,40,41,42,43,44,45,46,47,49-tridecol | |
---|---|---|
Source | PubChem | |
URL | https://pubchem.ncbi.nlm.nih.gov | |
Description | Data deposited in or computed by PubChem | |
InChI |
InChI=1S/C42H71NO34/c43-15-16(51)36-64-8(1-44)29(15)71-37-23(58)17(52)31(10(3-46)65-37)73-39-25(60)19(54)33(12(5-48)67-39)75-41-27(62)21(56)35(14(7-50)69-41)77-42-28(63)22(57)34(13(6-49)70-42)76-40-26(61)20(55)32(11(4-47)68-40)74-38-24(59)18(53)30(72-36)9(2-45)66-38/h8-42,44-63H,1-7,43H2/t8-,9-,10-,11-,12-,13-,14-,15+,16+,17-,18-,19-,20-,21-,22-,23-,24-,25-,26-,27-,28-,29-,30-,31-,32-,33-,34-,35-,36-,37-,38-,39-,40-,41-,42-/m1/s1 | |
Source | PubChem | |
URL | https://pubchem.ncbi.nlm.nih.gov | |
Description | Data deposited in or computed by PubChem | |
InChI Key |
OZLVLKFBPJMRCM-FUFZOPRGSA-N | |
Source | PubChem | |
URL | https://pubchem.ncbi.nlm.nih.gov | |
Description | Data deposited in or computed by PubChem | |
Canonical SMILES |
C(C1C2C(C(C(O1)OC3C(OC(C(C3O)O)OC4C(OC(C(C4O)O)OC5C(OC(C(C5O)O)OC6C(OC(C(C6O)O)OC7C(OC(C(C7O)O)OC8C(OC(O2)C(C8O)O)CO)CO)CO)CO)CO)CO)O)N)O | |
Source | PubChem | |
URL | https://pubchem.ncbi.nlm.nih.gov | |
Description | Data deposited in or computed by PubChem | |
Isomeric SMILES |
C([C@@H]1[C@@H]2[C@H]([C@@H]([C@H](O1)O[C@@H]3[C@H](O[C@@H]([C@@H]([C@H]3O)O)O[C@@H]4[C@H](O[C@@H]([C@@H]([C@H]4O)O)O[C@@H]5[C@H](O[C@@H]([C@@H]([C@H]5O)O)O[C@@H]6[C@H](O[C@@H]([C@@H]([C@H]6O)O)O[C@@H]7[C@H](O[C@@H]([C@@H]([C@H]7O)O)O[C@@H]8[C@H](O[C@H](O2)[C@@H]([C@H]8O)O)CO)CO)CO)CO)CO)CO)O)N)O | |
Source | PubChem | |
URL | https://pubchem.ncbi.nlm.nih.gov | |
Description | Data deposited in or computed by PubChem | |
Molecular Formula |
C42H71NO34 | |
Source | PubChem | |
URL | https://pubchem.ncbi.nlm.nih.gov | |
Description | Data deposited in or computed by PubChem | |
DSSTOX Substance ID |
DTXSID20462166 | |
Record name | 3A-Amino-3A-deoxy-(2AS,3AS)-beta-cyclodextrin | |
Source | EPA DSSTox | |
URL | https://comptox.epa.gov/dashboard/DTXSID20462166 | |
Description | DSSTox provides a high quality public chemistry resource for supporting improved predictive toxicology. | |
Molecular Weight |
1134.0 g/mol | |
Source | PubChem | |
URL | https://pubchem.ncbi.nlm.nih.gov | |
Description | Data deposited in or computed by PubChem | |
CAS No. |
117194-77-1 | |
Record name | 3A-Amino-3A-deoxy-(2AS,3AS)-beta-cyclodextrin | |
Source | EPA DSSTox | |
URL | https://comptox.epa.gov/dashboard/DTXSID20462166 | |
Description | DSSTox provides a high quality public chemistry resource for supporting improved predictive toxicology. | |
試験管内研究製品の免責事項と情報
BenchChemで提示されるすべての記事および製品情報は、情報提供を目的としています。BenchChemで購入可能な製品は、生体外研究のために特別に設計されています。生体外研究は、ラテン語の "in glass" に由来し、生物体の外で行われる実験を指します。これらの製品は医薬品または薬として分類されておらず、FDAから任何の医療状態、病気、または疾患の予防、治療、または治癒のために承認されていません。これらの製品を人間または動物に体内に導入する形態は、法律により厳格に禁止されています。これらのガイドラインに従うことは、研究と実験において法的および倫理的な基準の遵守を確実にするために重要です。