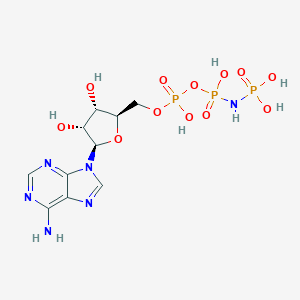
Phosphoaminophosphonic acid-adenylate ester
概要
説明
ホスホアミノホスホン酸アデニレートエステルは、アデノシン三リン酸(ATP)のアナログとして機能する化学化合物です。この化合物では、ベータからガンマリン酸へ架橋する酸素原子が窒素原子に置換されています。 この修飾により、可溶性および膜結合性ミトコンドリアATPアーゼの強力な競合阻害剤となるだけでなく、酸化リン酸化のATP依存性反応も阻害されます .
準備方法
ホスホアミノホスホン酸アデニレートエステルの合成には、5'-アデニル酸とイミドジリン酸の反応が含まれます。反応条件は通常、エステル結合の正しい形成を確実にするために制御された環境が必要です。 工業的な生産方法は、一貫性と純度を維持するために自動化反応器を用いた大規模合成を含む場合があります .
化学反応の分析
ホスホアミノホスホン酸アデニレートエステルは、次のようなさまざまな種類の化学反応を起こします。
酸化: この化合物は特定の条件下で酸化されて、さまざまな酸化された生成物が生成される可能性があります。
還元: 還元反応は、エステルをその還元型に変換することができ、これは異なる生物学的活性を持つ可能性があります。
置換: エステルは、官能基の1つが別の基に置換される置換反応を受けることができ、その化学的性質が変わります。
これらの反応に使用される一般的な試薬には、過酸化水素などの酸化剤、水素化ホウ素ナトリウムなどの還元剤、および置換反応を促進するさまざまな触媒が含まれます。 これらの反応によって生成される主要な生成物は、使用される特定の条件と試薬によって異なります .
科学研究への応用
ホスホアミノホスホン酸アデニレートエステルは、幅広い科学研究への応用があります。
化学: ATPアナログとその酵素との相互作用を研究するためのモデル化合物として使用されます。
生物学: この化合物は、ミトコンドリアATPアーゼの阻害とその細胞代謝への影響を理解するための研究で使用されます。
医学: ATP依存性プロセスを阻害する能力により、エネルギー代謝に関連する疾患の治療に役立つ可能性のある治療的用途があります。
科学的研究の応用
Phosphoaminophosphonic Acid-Adenylate Ester has a wide range of scientific research applications:
Chemistry: It is used as a model compound to study ATP analogs and their interactions with enzymes.
Biology: The compound is employed in research to understand mitochondrial ATPase inhibition and its effects on cellular metabolism.
Medicine: It has potential therapeutic applications due to its ability to inhibit ATP-dependent processes, which could be useful in treating diseases related to energy metabolism.
作用機序
ホスホアミノホスホン酸アデニレートエステルの作用機序は、ミトコンドリアATPアーゼとの相互作用を含みます。酸素原子を窒素原子に置換することにより、この化合物は酵素の活性を阻害し、ATPの加水分解を防ぎます。この阻害は、酸化リン酸化のATP依存性反応に影響を与え、細胞のエネルギー代謝が変化します。 分子標的は、ATP合成および利用に関与するさまざまなキナーゼおよび酵素です .
類似の化合物との比較
ホスホアミノホスホン酸アデニレートエステルは、窒素置換のためにユニークであり、他のATPアナログとは異なります。類似の化合物には次のものがあります。
ホスホアミノホスホン酸グアニレートエステル: 同様の阻害特性を持つグアノシン三リン酸(GTP)のアナログ.
アデノシン-5'-ホスホスルファート: 生化学研究で使用される別のATPアナログ.
アデノシン-5'-二リン酸: エネルギー代謝に関与する天然のATP誘導体.
これらの化合物は構造的に類似していますが、酵素に対する特定の相互作用と阻害効果が異なります。
類似化合物との比較
Phosphoaminophosphonic Acid-Adenylate Ester is unique due to its nitrogen substitution, which distinguishes it from other ATP analogs. Similar compounds include:
Phosphoaminophosphonic Acid-Guanylate Ester: An analog of guanosine triphosphate (GTP) with similar inhibitory properties.
Adenosine-5’-Phosphosulfate: Another ATP analog used in biochemical research.
Adenosine-5’-Diphosphate: A naturally occurring ATP derivative involved in energy metabolism.
These compounds share structural similarities but differ in their specific interactions and inhibitory effects on enzymes.
特性
IUPAC Name |
[[[[(2R,3S,4R,5R)-5-(6-aminopurin-9-yl)-3,4-dihydroxyoxolan-2-yl]methoxy-hydroxyphosphoryl]oxy-hydroxyphosphoryl]amino]phosphonic acid | |
---|---|---|
Source | PubChem | |
URL | https://pubchem.ncbi.nlm.nih.gov | |
Description | Data deposited in or computed by PubChem | |
InChI |
InChI=1S/C10H17N6O12P3/c11-8-5-9(13-2-12-8)16(3-14-5)10-7(18)6(17)4(27-10)1-26-31(24,25)28-30(22,23)15-29(19,20)21/h2-4,6-7,10,17-18H,1H2,(H,24,25)(H2,11,12,13)(H4,15,19,20,21,22,23)/t4-,6-,7-,10-/m1/s1 | |
Source | PubChem | |
URL | https://pubchem.ncbi.nlm.nih.gov | |
Description | Data deposited in or computed by PubChem | |
InChI Key |
PVKSNHVPLWYQGJ-KQYNXXCUSA-N | |
Source | PubChem | |
URL | https://pubchem.ncbi.nlm.nih.gov | |
Description | Data deposited in or computed by PubChem | |
Canonical SMILES |
C1=NC(=C2C(=N1)N(C=N2)C3C(C(C(O3)COP(=O)(O)OP(=O)(NP(=O)(O)O)O)O)O)N | |
Source | PubChem | |
URL | https://pubchem.ncbi.nlm.nih.gov | |
Description | Data deposited in or computed by PubChem | |
Isomeric SMILES |
C1=NC(=C2C(=N1)N(C=N2)[C@H]3[C@@H]([C@@H]([C@H](O3)COP(=O)(O)OP(=O)(NP(=O)(O)O)O)O)O)N | |
Source | PubChem | |
URL | https://pubchem.ncbi.nlm.nih.gov | |
Description | Data deposited in or computed by PubChem | |
Molecular Formula |
C10H17N6O12P3 | |
Source | PubChem | |
URL | https://pubchem.ncbi.nlm.nih.gov | |
Description | Data deposited in or computed by PubChem | |
DSSTOX Substance ID |
DTXSID90180289 | |
Record name | Adenylyl imidodiphosphate | |
Source | EPA DSSTox | |
URL | https://comptox.epa.gov/dashboard/DTXSID90180289 | |
Description | DSSTox provides a high quality public chemistry resource for supporting improved predictive toxicology. | |
Molecular Weight |
506.20 g/mol | |
Source | PubChem | |
URL | https://pubchem.ncbi.nlm.nih.gov | |
Description | Data deposited in or computed by PubChem | |
CAS No. |
25612-73-1, 114661-04-0 | |
Record name | 5′-Adenylic acid, anhydride with imidodiphosphoric acid (1:1) | |
Source | CAS Common Chemistry | |
URL | https://commonchemistry.cas.org/detail?cas_rn=25612-73-1 | |
Description | CAS Common Chemistry is an open community resource for accessing chemical information. Nearly 500,000 chemical substances from CAS REGISTRY cover areas of community interest, including common and frequently regulated chemicals, and those relevant to high school and undergraduate chemistry classes. This chemical information, curated by our expert scientists, is provided in alignment with our mission as a division of the American Chemical Society. | |
Explanation | The data from CAS Common Chemistry is provided under a CC-BY-NC 4.0 license, unless otherwise stated. | |
Record name | Adenylyl imidodiphosphate | |
Source | ChemIDplus | |
URL | https://pubchem.ncbi.nlm.nih.gov/substance/?source=chemidplus&sourceid=0025612731 | |
Description | ChemIDplus is a free, web search system that provides access to the structure and nomenclature authority files used for the identification of chemical substances cited in National Library of Medicine (NLM) databases, including the TOXNET system. | |
Record name | Formycin A 5'-(betagamma-imido)triphosphate | |
Source | ChemIDplus | |
URL | https://pubchem.ncbi.nlm.nih.gov/substance/?source=chemidplus&sourceid=0114661040 | |
Description | ChemIDplus is a free, web search system that provides access to the structure and nomenclature authority files used for the identification of chemical substances cited in National Library of Medicine (NLM) databases, including the TOXNET system. | |
Record name | Adenylyl imidodiphosphate | |
Source | EPA DSSTox | |
URL | https://comptox.epa.gov/dashboard/DTXSID90180289 | |
Description | DSSTox provides a high quality public chemistry resource for supporting improved predictive toxicology. | |
Retrosynthesis Analysis
AI-Powered Synthesis Planning: Our tool employs the Template_relevance Pistachio, Template_relevance Bkms_metabolic, Template_relevance Pistachio_ringbreaker, Template_relevance Reaxys, Template_relevance Reaxys_biocatalysis model, leveraging a vast database of chemical reactions to predict feasible synthetic routes.
One-Step Synthesis Focus: Specifically designed for one-step synthesis, it provides concise and direct routes for your target compounds, streamlining the synthesis process.
Accurate Predictions: Utilizing the extensive PISTACHIO, BKMS_METABOLIC, PISTACHIO_RINGBREAKER, REAXYS, REAXYS_BIOCATALYSIS database, our tool offers high-accuracy predictions, reflecting the latest in chemical research and data.
Strategy Settings
Precursor scoring | Relevance Heuristic |
---|---|
Min. plausibility | 0.01 |
Model | Template_relevance |
Template Set | Pistachio/Bkms_metabolic/Pistachio_ringbreaker/Reaxys/Reaxys_biocatalysis |
Top-N result to add to graph | 6 |
Feasible Synthetic Routes
試験管内研究製品の免責事項と情報
BenchChemで提示されるすべての記事および製品情報は、情報提供を目的としています。BenchChemで購入可能な製品は、生体外研究のために特別に設計されています。生体外研究は、ラテン語の "in glass" に由来し、生物体の外で行われる実験を指します。これらの製品は医薬品または薬として分類されておらず、FDAから任何の医療状態、病気、または疾患の予防、治療、または治癒のために承認されていません。これらの製品を人間または動物に体内に導入する形態は、法律により厳格に禁止されています。これらのガイドラインに従うことは、研究と実験において法的および倫理的な基準の遵守を確実にするために重要です。