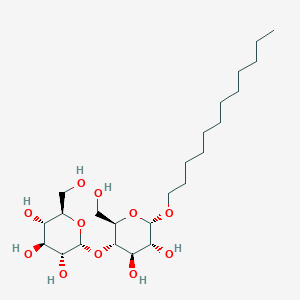
Dodecyl-alpha-D-maltoside
説明
ドデシル-α-D-マルトシドは、生化学および生物物理学の研究で広く用いられている非イオン性界面活性剤です。膜タンパク質やタンパク質複合体を可溶化することに特に優れており、細胞膜やタンパク質相互作用の研究に不可欠なツールとなっています。この化合物は、分子式C24H46O11、分子量510.62 g/molです .
2. 製法
合成経路と反応条件: ドデシル-α-D-マルトシドは、マルトースとドデカンオールの糖化によって合成されます。反応は通常、マルトースとドデカンオール間の糖苷結合の形成を促進するために酸触媒を用います。このプロセスは以下のように要約できます。
ステップ1: 適切な活性化基(例えば、トリクロロアセチミデート)を用いたマルトースの活性化。
ステップ2: 酸触媒(例えば、トリフルオロメタンスルホン酸)の存在下での活性化されたマルトースとドデカンオールの反応。
ステップ3: 結晶化またはクロマトグラフィーによる生成物の精製。
工業的生産方法: 工業的な環境では、ドデシル-α-D-マルトシドの生産は、高収率と高純度を確保するために制御された条件下での大規模な糖化反応を伴います。このプロセスは、費用対効果とスケーラビリティを最適化するために、連続フロー反応器や自動化された精製システムをしばしば利用します。
作用機序
ドデシル-α-D-マルトシドがその効果を発揮する主なメカニズムは、脂質二重層を破壊する能力にあります。この化合物は脂質二重層に挿入され、表面張力を低下させ、膜タンパク質の可溶化につながります。この作用は、疎水性ドデシル鎖と親水性マルトースヘッドにより促進され、それぞれ脂質相と水相と相互作用します .
類似化合物:
オクチル-β-D-グルコシド: アルキル鎖が短く、大きなタンパク質複合体を可溶化するための効果が低い非イオン性界面活性剤です。
Triton X-100: 広く用いられている非イオン性界面活性剤で、親水性ヘッドグループが異なります。類似の用途で使用されますが、可溶化特性は異なります。
ラウリルマルトシド: ドデシル-α-D-マルトシドに似ていますが、アルキル鎖の長さと分岐にわずかな違いがあります。
独自性: ドデシル-α-D-マルトシドは、疎水性と親水性の特性の最適なバランスにより、幅広い膜タンパク質を可溶化し、変性させずに非常に効果的であるという点でユニークです。臨界ミセル濃度(CMC)が低いため、少量で効率的に使用できます .
生化学分析
Biochemical Properties
Dodecyl-alpha-D-maltoside plays a significant role in biochemical reactions, particularly in the solubilization and crystallization of membrane proteins . It interacts with various enzymes, proteins, and other biomolecules, maintaining the solution-phase conformation of the protein .
Cellular Effects
This compound has been used to solubilize photosystem I (PSI) and photosystem II (PSII) complexes from plant thylakoid membranes, as well as intact grana . It influences cell function by facilitating the study of membrane proteins in their native state .
Molecular Mechanism
The mechanism of action of this compound involves its ability to form micelles that mimic the membrane environment, thereby preserving the alpha-helical structures and native conformations of membrane-associated proteins . It can also increase the solubility of certain compounds in aqueous solution .
Temporal Effects in Laboratory Settings
The effects of this compound can change over time in laboratory settings. For instance, it has been shown to stabilize intact oligomeric membrane protein complexes during the transition to vacuum .
Metabolic Pathways
It is known to interact with various enzymes and cofactors in the process of solubilizing membrane proteins .
Transport and Distribution
This compound is known to be involved in the transport and distribution of membrane proteins within cells and tissues . It interacts with various transporters and binding proteins, influencing its localization or accumulation.
Subcellular Localization
It is known to play a crucial role in the study of membrane proteins, which are localized in various compartments or organelles within the cell .
準備方法
Synthetic Routes and Reaction Conditions: Dodecyl-Alpha-D-Maltoside is synthesized through the glycosylation of maltose with dodecanol. The reaction typically involves the use of an acid catalyst to facilitate the formation of the glycosidic bond between the maltose and the dodecanol. The process can be summarized as follows:
Step 1: Activation of maltose with a suitable activating group (e.g., trichloroacetimidate).
Step 2: Reaction of the activated maltose with dodecanol in the presence of an acid catalyst (e.g., trifluoromethanesulfonic acid).
Step 3: Purification of the product through crystallization or chromatography.
Industrial Production Methods: In industrial settings, the production of this compound involves large-scale glycosylation reactions under controlled conditions to ensure high yield and purity. The process is optimized for cost-effectiveness and scalability, often utilizing continuous flow reactors and automated purification systems.
化学反応の分析
反応の種類: ドデシル-α-D-マルトシドは、その構造中のヒドロキシル基の存在により、主に置換反応を起こします。これらの反応は、化合物を特定の用途のために修飾するために使用できます。
一般的な試薬と条件:
酸化: 過酸化水素などの穏やかな酸化剤を用いて、ヒドロキシル基をカルボニル基に酸化することができます。
還元: 水素化ホウ素ナトリウムなどの還元剤を用いて、カルボニル基をヒドロキシル基に還元することができます。
置換: 様々な求核剤を塩基性条件下でヒドロキシル基に導入して、エーテルまたはエステルを形成することができます。
主な生成物: これらの反応の主な生成物には、特定の研究ニーズに合わせて調整できる、官能基が変化したドデシル-α-D-マルトシドの修飾バージョンが含まれます。
4. 科学研究での応用
ドデシル-α-D-マルトシドは、科学研究の様々な分野で広く用いられています。
化学: ナノ粒子の合成と安定化における界面活性剤として用いられます。
生物学: この化合物は、膜タンパク質を可溶化するために不可欠であり、X線結晶解析や核磁気共鳴分光法などの技術を用いた研究を促進します。
医学: 疎水性薬物の溶解性と安定性を向上させ、薬物送達システムの製剤に役立ちます。
産業: ドデシル-α-D-マルトシドは、その穏やかで刺激のない性質により、パーソナルケア製品や洗剤の製剤に使用されています.
科学的研究の応用
Dodecyl-Alpha-D-Maltoside is extensively used in various fields of scientific research:
Chemistry: It is used as a surfactant in the synthesis and stabilization of nanoparticles.
Biology: The compound is crucial for solubilizing membrane proteins, facilitating their study through techniques such as X-ray crystallography and nuclear magnetic resonance spectroscopy.
Medicine: It aids in the formulation of drug delivery systems, enhancing the solubility and stability of hydrophobic drugs.
類似化合物との比較
Octyl-β-D-Glucoside: Another non-ionic detergent with a shorter alkyl chain, making it less effective in solubilizing large protein complexes.
Triton X-100: A widely used non-ionic detergent with a different hydrophilic head group, often used in similar applications but with different solubilization properties.
Lauryl Maltoside: Similar to Dodecyl-Alpha-D-Maltoside but with slight variations in the alkyl chain length and branching.
Uniqueness: this compound is unique due to its optimal balance of hydrophobic and hydrophilic properties, making it highly effective in solubilizing a wide range of membrane proteins without denaturing them. Its critical micelle concentration (CMC) is low, allowing for efficient use in small quantities .
特性
IUPAC Name |
(2R,3R,4S,5S,6R)-2-[(2R,3S,4R,5R,6S)-6-dodecoxy-4,5-dihydroxy-2-(hydroxymethyl)oxan-3-yl]oxy-6-(hydroxymethyl)oxane-3,4,5-triol | |
---|---|---|
Source | PubChem | |
URL | https://pubchem.ncbi.nlm.nih.gov | |
Description | Data deposited in or computed by PubChem | |
InChI |
InChI=1S/C24H46O11/c1-2-3-4-5-6-7-8-9-10-11-12-32-23-21(31)19(29)22(16(14-26)34-23)35-24-20(30)18(28)17(27)15(13-25)33-24/h15-31H,2-14H2,1H3/t15-,16-,17-,18+,19-,20-,21-,22-,23+,24-/m1/s1 | |
Source | PubChem | |
URL | https://pubchem.ncbi.nlm.nih.gov | |
Description | Data deposited in or computed by PubChem | |
InChI Key |
NLEBIOOXCVAHBD-YHBSTRCHSA-N | |
Source | PubChem | |
URL | https://pubchem.ncbi.nlm.nih.gov | |
Description | Data deposited in or computed by PubChem | |
Canonical SMILES |
CCCCCCCCCCCCOC1C(C(C(C(O1)CO)OC2C(C(C(C(O2)CO)O)O)O)O)O | |
Source | PubChem | |
URL | https://pubchem.ncbi.nlm.nih.gov | |
Description | Data deposited in or computed by PubChem | |
Isomeric SMILES |
CCCCCCCCCCCCO[C@@H]1[C@@H]([C@H]([C@@H]([C@H](O1)CO)O[C@@H]2[C@@H]([C@H]([C@@H]([C@H](O2)CO)O)O)O)O)O | |
Source | PubChem | |
URL | https://pubchem.ncbi.nlm.nih.gov | |
Description | Data deposited in or computed by PubChem | |
Molecular Formula |
C24H46O11 | |
Source | PubChem | |
URL | https://pubchem.ncbi.nlm.nih.gov | |
Description | Data deposited in or computed by PubChem | |
Molecular Weight |
510.6 g/mol | |
Source | PubChem | |
URL | https://pubchem.ncbi.nlm.nih.gov | |
Description | Data deposited in or computed by PubChem | |
CAS No. |
116183-64-3 | |
Record name | (2R,3R,4S,5S,6R)-2-[(2R,3S,4R,5R,6S)-6-dodecoxy-4,5-dihydroxy-2-(hydroxymethyl)oxan-3-yl]oxy-6-(hydroxymethyl)oxane-3,4,5-triol | |
Source | European Chemicals Agency (ECHA) | |
URL | https://echa.europa.eu/information-on-chemicals | |
Description | The European Chemicals Agency (ECHA) is an agency of the European Union which is the driving force among regulatory authorities in implementing the EU's groundbreaking chemicals legislation for the benefit of human health and the environment as well as for innovation and competitiveness. | |
Explanation | Use of the information, documents and data from the ECHA website is subject to the terms and conditions of this Legal Notice, and subject to other binding limitations provided for under applicable law, the information, documents and data made available on the ECHA website may be reproduced, distributed and/or used, totally or in part, for non-commercial purposes provided that ECHA is acknowledged as the source: "Source: European Chemicals Agency, http://echa.europa.eu/". Such acknowledgement must be included in each copy of the material. ECHA permits and encourages organisations and individuals to create links to the ECHA website under the following cumulative conditions: Links can only be made to webpages that provide a link to the Legal Notice page. | |
Q1: Why is dodecyl-alpha-D-maltoside used in structural studies of membrane proteins like Photosystem II?
A: this compound is a mild detergent often used to extract and purify membrane proteins while preserving their native structure and function. This is crucial for techniques like electron microscopy, which require the protein to be in solution but retain its natural conformation. For example, researchers used this compound to isolate Photosystem II complexes from spinach, allowing them to visualize different supramolecular arrangements of the photosystem and its light-harvesting antenna [].
Q2: How does this compound assist in the crystallization of proteins like the human retinoic acid receptor (hRARgamma)?
A: Crystallization of proteins is often a delicate process. This compound can aid in this process by stabilizing the protein and mediating crystal contacts. In the case of hRARgamma, this compound was essential for both the stabilization and crystallization of the ligand-binding domain []. The detergent molecule was found to be completely ordered within the crystal structure, with its dodecyl moiety buried in a hydrophobic channel and the maltoside head group forming hydrogen bonds with water molecules and polar residues. This suggests that this compound can play a specific structural role in facilitating protein crystallization.
Q3: Can you elaborate on the specific structural insights gained from using this compound in studying green plant Photosystem I?
A: By using this compound to solubilize green plant Photosystem I, researchers were able to perform electron microscopy studies that revealed important structural details about this complex []. The study demonstrated that the light-harvesting complex I (LHCI) binds to the Photosystem I core complex on the side of the F/J subunits. This finding contributes to a better understanding of the spatial organization of light-harvesting complexes within Photosystem I, which is essential for efficient energy transfer during photosynthesis.
Q4: The studies mention the formation of different "supercomplexes" of Photosystem II after treatment with this compound. What does this tell us about the organization of the thylakoid membrane?
A: The observation of different Photosystem II supercomplexes with varying compositions of light-harvesting complexes suggests that the thylakoid membrane is not a static structure, but rather a dynamic environment [, ]. The ability of this compound to extract these different supercomplexes indicates that they exist as distinct entities within the membrane. This heterogeneity in Photosystem II organization may reflect the plant's ability to adapt to changing light conditions by altering the pathways of energy transfer. For example, different supercomplexes might be favored under high or low light conditions to optimize photosynthetic efficiency.
試験管内研究製品の免責事項と情報
BenchChemで提示されるすべての記事および製品情報は、情報提供を目的としています。BenchChemで購入可能な製品は、生体外研究のために特別に設計されています。生体外研究は、ラテン語の "in glass" に由来し、生物体の外で行われる実験を指します。これらの製品は医薬品または薬として分類されておらず、FDAから任何の医療状態、病気、または疾患の予防、治療、または治癒のために承認されていません。これらの製品を人間または動物に体内に導入する形態は、法律により厳格に禁止されています。これらのガイドラインに従うことは、研究と実験において法的および倫理的な基準の遵守を確実にするために重要です。