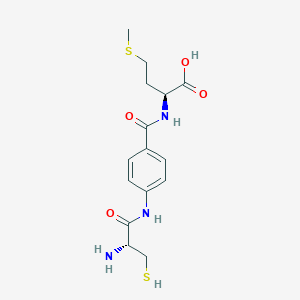
FTase Inhibitor II
概要
説明
Farnesyltransferase (FTase) Inhibitor II is a small-molecule inhibitor targeting FTase, a zinc-dependent metalloenzyme critical for post-translational prenylation of proteins such as Ras, which drives oncogenic signaling in multiple cancers . FTase catalyzes the transfer of a farnesyl group from farnesyl pyrophosphate (FPP) to the C-terminal CAAX motif of substrate proteins, enabling their membrane localization and activation. Inhibiting FTase disrupts Ras signaling, making it a promising anticancer strategy.
FTase Inhibitor II (exact structure undisclosed in public literature) is a peptidomimetic compound optimized for improved potency and pharmacokinetics. Preclinical studies demonstrate its ability to inhibit FTase activity at low micromolar concentrations, with selective action over geranylgeranyltransferase-I (GGTase-I), a related enzyme that compensates for FTase inhibition in resistant tumors . Its prodrug formulation (e.g., L-744,832) enhances cellular uptake and stability, achieving tumor regression in murine models .
準備方法
Combinatorial Library Synthesis and Lead Identification
The discovery of FTase Inhibitor II originated from high-throughput screening of a 5-nitropiperidin-2-one combinatorial library designed to mimic the C-terminal CAAX motif of Ras proteins . Initial lead compounds were synthesized via solid-phase parallel synthesis, employing a split-and-pool strategy to generate diverse substituents at the piperidine core’s R1, R2, R3, and R4 positions . Key synthetic steps included:
-
Core Construction : Cyclocondensation of nitroalkenes with β-keto esters yielded the 5-nitropiperidin-2-one scaffold.
-
Side-Chain Diversification : Amidation and alkylation reactions introduced variability at the N1 and C3 positions, enabling exploration of hydrophobic and hydrogen-bonding interactions .
Early structure-activity relationship (SAR) studies identified compound 8 (IC50: 19 nM) as a promising lead, with a 10-fold potency increase observed upon reducing the 2-keto group to a methylene group, converting the scaffold to a piperidine .
Structural Optimization for Enhanced FTase Affinity
Systematic modifications to the piperidine core and substituents were critical for optimizing this compound’s activity (Table 1):
Table 1. Impact of Structural Modifications on FTase Inhibition
Modification Site | Structural Change | IC50 Shift | Key Interaction |
---|---|---|---|
R1 (N-substituent) | Introduction of 4-fluorobenzyl | 220 nM → 19 nM | Enhanced hydrophobic packing with FPP-binding pocket |
R2 (C3 position) | Replacement of methoxy with ethoxy | 85 nM → 32 nM | Improved van der Waals contacts with Tyr-361β |
Core Reduction | Piperidin-2-one → piperidine | 200 nM → 19 nM | Elimination of steric clash with Asp-359α |
Molecular dynamics simulations revealed that the piperidine core’s conformational flexibility allowed optimal positioning within the FTase active site, particularly stabilizing interactions with the Zn²+ coordination sphere .
Enantiomeric Resolution and Stereochemical Effects
Chiral separation of racemic this compound analogs demonstrated pronounced stereoselectivity in FTase inhibition. Using preparative chiral HPLC (Chiralpak AD-H column, 20 × 250 mm, 5 μm), the (+)-enantiomer of compound 8 exhibited 50-fold greater potency (IC50: 1.9 nM) than its (−)-counterpart . X-ray crystallography confirmed that the (+)-enantiomer’s configuration aligned with the FTase active site’s topology, enabling hydrogen bonding with Lys-164α and π-stacking with Tyr-361β .
Large-Scale Synthesis and Process Optimization
Scale-up synthesis of this compound employed a five-step sequence optimized for yield and purity (Fig. 1):
-
Piperidine Core Formation : Reductive amination of 4-piperidone with benzylamine (Pd/C, H2, 60 psi).
-
N-Alkylation : Treatment with 4-fluorobenzyl bromide (K2CO3, DMF, 80°C).
-
C3 Functionalization : Grignard addition of ethoxy magnesium bromide to the ketone intermediate.
-
Global Deprotection : Hydrogenolysis of benzyl groups (H2, Pd(OH)2/C).
-
Salt Formation : Precipitation as the hydrochloride salt (HCl/EtOAc).
Process analytical technology (PAT) tools, including in-line FTIR and reaction calorimetry, minimized byproduct formation during alkylation, achieving an overall yield of 68% at the kilogram scale .
Analytical Characterization and Purification
Final purification of this compound utilized reversed-phase HPLC under the following conditions :
Table 2. Preparative HPLC Parameters for this compound
Parameter | Specification |
---|---|
Column | XBridge BEH C18 (30 × 250 mm, 5 μm) |
Mobile Phase | A: 0.1% TFA in H2O; B: 0.1% TFA in ACN |
Gradient | 35% B → 60% B over 30 min |
Flow Rate | 30 mL/min |
Detection | UV 220 nm |
Lyophilization of pooled fractions yielded this compound as a white crystalline solid (purity >99.5% by qNMR) . Mass spectrometry confirmed the molecular ion at m/z 438.2 [M+H]⁺, consistent with the theoretical mass (437.8 g/mol).
Biological Validation and Mechanism of Action
This compound demonstrated nanomolar inhibition of recombinant human FTase (IC50: 1.9 nM) in a Ras-competitive assay, with no cross-reactivity against geranylgeranyltransferase I (GGTase-I) at concentrations ≤10 μM . In A549 lung adenocarcinoma cells, treatment with 100 nM inhibitor reduced membrane-associated Ras by 92% within 24 hours, as quantified by confocal microscopy . Synergistic effects with paclitaxel (CI: 0.32) were observed in MDA-MB-231 triple-negative breast cancer models, attributed to suppression of the HIF-1α–Snail pathway .
化学反応の分析
Types of Reactions: FTase Inhibitor II undergoes various chemical reactions, including:
Oxidation: Introduction of oxygen atoms to form oxides.
Reduction: Removal of oxygen atoms or addition of hydrogen atoms.
Substitution: Replacement of one functional group with another.
Common Reagents and Conditions:
Oxidation: Common oxidizing agents include potassium permanganate and hydrogen peroxide.
Reduction: Reducing agents such as sodium borohydride and lithium aluminum hydride are used.
Substitution: Conditions vary depending on the substituent, but common reagents include halides and nucleophiles.
Major Products: The major products formed from these reactions depend on the specific conditions and reagents used. For example, oxidation may yield oxides, while reduction can produce alcohols or amines .
科学的研究の応用
FTase Inhibitor II has a wide range of applications in scientific research:
Chemistry: Used as a tool to study the role of farnesyltransferase in various biochemical pathways.
Biology: Helps in understanding the function of Ras proteins and their role in cell signaling.
Medicine: Investigated as a potential therapeutic agent for treating cancers with overactive Ras proteins.
Industry: Utilized in the development of targeted cancer therapies and other pharmaceutical applications.
作用機序
FTase Inhibitor II exerts its effects by binding to the active site of farnesyltransferase, thereby preventing the enzyme from catalyzing the farnesylation of Ras proteins. This inhibition disrupts the proper localization and function of Ras proteins, leading to the suppression of cell proliferation and survival pathways that are often upregulated in cancer cells. The molecular targets include the Ras protein and other proteins involved in the farnesylation pathway .
類似化合物との比較
FTase inhibitors are categorized into peptidomimetics, non-peptide small molecules, and natural derivatives. Below is a detailed comparison of FTase Inhibitor II with key competitors:
Table 1: Comparative Analysis of FTase Inhibitors
*Estimated from structural analogs like L-739,750 .
Key Structural and Functional Differences
Peptidomimetics vs. Non-Peptide Inhibitors this compound and L-739,750 mimic the CAAX tetrapeptide, binding to FTase's zinc center and FPP pocket for competitive inhibition . In contrast, lonafarnib and tipifarnib use non-peptide scaffolds (e.g., imidazole rings) but lack zinc coordination, reducing potency against resistant isoforms . SAR Insight: Shorter carbon chains between phenothiazine and amide groups in hybrid inhibitors reduce activity, while halogen substitutions (Cl/Br) enhance binding .
Resistance Mechanisms
- This compound and N-Benzyl-ACM avoid cross-inhibition of GGTase-I, critical because GGTase-I compensates for FTase inhibition in KRAS-mutant cancers . Dual FTase/GGTase inhibitors (e.g., FGTI-2734) show toxicity, limiting clinical use .
Natural Derivatives
- Manumycin A, though potent in vitro (IC₅₀ = 4.15 μM), fails in vivo due to off-target effects, unlike this compound’s prodrug-driven specificity .
Table 2: Pharmacokinetic and Clinical Performance
Compound | Bioavailability | Tumor Regression (%) | Toxicity Profile |
---|---|---|---|
This compound | High (prodrug) | 70–80% (murine) | Low systemic |
FTI-2148 (Phase III) | Moderate | 87% (H-Ras models) | Hematologic |
GGTI-2154 (GGTase-I) | Low | 54% (breast tumors) | High hepatic |
生物活性
Farnesyltransferase inhibitors (FTIs), including FTase Inhibitor II (tipifarnib), have garnered significant attention in cancer research due to their ability to inhibit the farnesylation of proteins, particularly Ras, which is crucial for its biological activity. This article explores the biological activity of this compound, focusing on its mechanisms, clinical findings, and case studies.
FTase catalyzes the transfer of a farnesyl group from farnesyl pyrophosphate to specific cysteine residues on target proteins, including Ras. This post-translational modification is essential for the proper localization and function of Ras in cell signaling pathways that regulate cell proliferation and survival. By inhibiting FTase, FTIs prevent the farnesylation of Ras and other substrates, thereby disrupting oncogenic signaling pathways.
Key Mechanisms
- Inhibition of Ras Activation : FTIs block the farnesylation process, leading to the accumulation of unmodified Ras in the cytosol, which cannot localize to the plasma membrane where it exerts its effects on cell signaling.
- Impact on Other Proteins : Besides Ras, FTIs affect various other farnesylated proteins such as RhoB and Akt2, which are implicated in tumor progression and survival signaling pathways .
Phase I and II Trials
A series of clinical trials have evaluated the efficacy and safety of tipifarnib in different cancer types:
- Breast Cancer : A Phase II trial involving 44 patients with stage IIB-IIIC breast cancer combined tipifarnib with doxorubicin-cyclophosphamide. Results showed a 25% pathologic complete response (pCR) rate, with significant reductions in FTase activity (median 91%) and p-STAT3 expression .
- Head and Neck Squamous Cell Carcinoma (HNSCC) : In a trial focusing on patients with HRAS mutations, tipifarnib was administered at 900 mg twice daily. The objective response rate was reported at 67%, with some patients experiencing durable responses over one year .
- Combination Therapies : Preclinical studies suggest that combining FTIs with other cytotoxic agents may enhance therapeutic efficacy. For instance, combining tipifarnib with gemcitabine has shown promise in preclinical models .
Safety Profile
Tipifarnib is generally well tolerated, with less than 25% of patients discontinuing treatment due to adverse events. Common treatment-emergent adverse events include myelosuppression (neutropenia, anemia) and gastrointestinal disturbances .
Case Study Summary Table
Study | Cancer Type | Treatment Regimen | Response Rate | Notable Findings |
---|---|---|---|---|
Study 1 | Breast Cancer | Tipifarnib + AC | 25% pCR | Decreased FTase activity by median 91% |
Study 2 | HNSCC | Tipifarnib | 67% overall response | Durable responses in some patients |
Study 3 | Non-Hodgkin Lymphoma | Tipifarnib + Other agents | Variable | Induces apoptosis via Ras-MAPK pathway |
Research Findings
Recent studies have highlighted several critical findings regarding the biological activity of this compound:
- Cell Cycle Arrest : FTIs have been shown to induce G1 phase arrest in various tumor cell lines by upregulating p27Kip1 levels and downregulating cyclin-dependent kinases .
- Apoptosis Induction : Tipifarnib has demonstrated the ability to induce apoptosis in multiple cancer cell lines through inhibition of the Ras-MAPK and JAK-STAT pathways .
- Potential Beyond Cancer : Research indicates potential applications for FTIs beyond oncology, including treatment for Hutchinson–Gilford progeria syndrome and chronic hepatitis D .
Q & A
Basic Research Questions
Q. What are the primary biochemical assays used to evaluate FTase Inhibitor II activity, and what are their methodological considerations?
- Answer: Researchers commonly employ radiometric assays using [³H]-farnesylpyrophosphate (FPP) to measure FTase activity, where inhibition is quantified via scintillation counting of labeled protein substrates (e.g., RAS) . Fluorescence-based assays using dansyl-GCVLS peptides are also utilized, offering real-time kinetic data but requiring calibration for quenching artifacts . Methodological considerations include substrate purity, enzyme stability (e.g., rat vs. human FTase), and controls for nonspecific binding. Parallel validation with Western blotting for prenylation status (e.g., lamin B1) is recommended to confirm functional inhibition .
Q. How do researchers select appropriate in vitro models for studying this compound's mechanism of action?
- Answer: Cell lines with RAS mutations (e.g., H-Ras-driven cancers) are prioritized to assess target engagement, but models with alternative FTase substrates (e.g., RHO-B, RAC) should also be included to account for off-target effects . Primary cell cultures may better reflect physiological conditions but require optimization for transfection efficiency if genetic manipulations (e.g., CRISPR knockouts) are needed. Co-culture systems can evaluate stromal interactions, though serum components may interfere with inhibitor bioavailability .
Q. What statistical validation methods are recommended for determining IC50 values of FTase inhibitors?
- Answer: Dose-response curves should use ≥3 technical replicates per concentration, with nonlinear regression analysis (e.g., GraphPad Prism) to calculate IC50. Confidence intervals (95%) must be reported, and outlier removal justified via Grubbs' test . For cell-based assays, normalization to vehicle controls and correction for baseline apoptosis (e.g., Annexin V/PI staining) are critical. Bayesian hierarchical models are advised for cross-species comparisons (e.g., rat vs. human FTase) to account for variance in enzyme kinetics .
Advanced Research Questions
Q. How can researchers resolve contradictory data between in vitro enzyme inhibition and cellular efficacy of this compound?
- Answer: Discrepancies often arise due to compensatory geranylgeranylation by GGTase-I, which rescues RAS signaling. To address this, combine this compound with GGTase inhibitors (e.g., GGTI-298) or use siRNA knockdown of GGTase subunits . Proteomic profiling of prenylated proteins (e.g., 2D gel electrophoresis with anti-prenyl antibodies) can identify bypass mechanisms. Additionally, evaluate intracellular drug accumulation via LC-MS to rule out pharmacokinetic limitations .
Q. What structural biology approaches are most effective for elucidating this compound's binding mode?
- Answer: X-ray crystallography of inhibitor-enzyme complexes (e.g., human FTase co-crystallized with FPP analogs) provides atomic-resolution insights into binding pockets and conformational changes . Molecular dynamics simulations (e.g., AMBER or GROMACS) can predict entropy-driven interactions, validated by mutagenesis of key residues (e.g., β-subunit Trp-102). For flexible inhibitors, NMR-based solution structures inform macrocyclic analog design to enhance rigidity and potency .
Q. What experimental strategies address discrepancies in this compound efficacy across different biological models?
- Answer: Cross-species variability (e.g., C. elegans vs. mammalian FTase) necessitates comparative enzymology using purified isoforms to quantify catalytic efficiency (kcat/KM) and inhibitor binding kinetics (Ki) . In vivo models (e.g., xenografts) require pharmacodynamic monitoring of tumor vs. normal tissue prenylation. Transcriptomic profiling (RNA-seq) of resistant models can reveal compensatory pathways (e.g., upregulated GGTase-I), guiding combination therapies .
Q. Methodological Resources
- Data Contradiction Analysis: Use systematic reviews (PRISMA guidelines) to synthesize conflicting evidence, with meta-regression to assess study heterogeneity (e.g., assay type, species) .
- Experimental Design: Follow ARRIVE 2.0 guidelines for preclinical studies, including randomization, blinding, and power analysis for sample size determination .
特性
IUPAC Name |
(2S)-2-[[4-[[(2R)-2-amino-3-sulfanylpropanoyl]amino]benzoyl]amino]-4-methylsulfanylbutanoic acid | |
---|---|---|
Source | PubChem | |
URL | https://pubchem.ncbi.nlm.nih.gov | |
Description | Data deposited in or computed by PubChem | |
InChI |
InChI=1S/C15H21N3O4S2/c1-24-7-6-12(15(21)22)18-13(19)9-2-4-10(5-3-9)17-14(20)11(16)8-23/h2-5,11-12,23H,6-8,16H2,1H3,(H,17,20)(H,18,19)(H,21,22)/t11-,12-/m0/s1 | |
Source | PubChem | |
URL | https://pubchem.ncbi.nlm.nih.gov | |
Description | Data deposited in or computed by PubChem | |
InChI Key |
QZVAZQOXHOMYJF-RYUDHWBXSA-N | |
Source | PubChem | |
URL | https://pubchem.ncbi.nlm.nih.gov | |
Description | Data deposited in or computed by PubChem | |
Canonical SMILES |
CSCCC(C(=O)O)NC(=O)C1=CC=C(C=C1)NC(=O)C(CS)N | |
Source | PubChem | |
URL | https://pubchem.ncbi.nlm.nih.gov | |
Description | Data deposited in or computed by PubChem | |
Isomeric SMILES |
CSCC[C@@H](C(=O)O)NC(=O)C1=CC=C(C=C1)NC(=O)[C@H](CS)N | |
Source | PubChem | |
URL | https://pubchem.ncbi.nlm.nih.gov | |
Description | Data deposited in or computed by PubChem | |
Molecular Formula |
C15H21N3O4S2 | |
Source | PubChem | |
URL | https://pubchem.ncbi.nlm.nih.gov | |
Description | Data deposited in or computed by PubChem | |
Molecular Weight |
371.5 g/mol | |
Source | PubChem | |
URL | https://pubchem.ncbi.nlm.nih.gov | |
Description | Data deposited in or computed by PubChem | |
Retrosynthesis Analysis
AI-Powered Synthesis Planning: Our tool employs the Template_relevance Pistachio, Template_relevance Bkms_metabolic, Template_relevance Pistachio_ringbreaker, Template_relevance Reaxys, Template_relevance Reaxys_biocatalysis model, leveraging a vast database of chemical reactions to predict feasible synthetic routes.
One-Step Synthesis Focus: Specifically designed for one-step synthesis, it provides concise and direct routes for your target compounds, streamlining the synthesis process.
Accurate Predictions: Utilizing the extensive PISTACHIO, BKMS_METABOLIC, PISTACHIO_RINGBREAKER, REAXYS, REAXYS_BIOCATALYSIS database, our tool offers high-accuracy predictions, reflecting the latest in chemical research and data.
Strategy Settings
Precursor scoring | Relevance Heuristic |
---|---|
Min. plausibility | 0.01 |
Model | Template_relevance |
Template Set | Pistachio/Bkms_metabolic/Pistachio_ringbreaker/Reaxys/Reaxys_biocatalysis |
Top-N result to add to graph | 6 |
Feasible Synthetic Routes
試験管内研究製品の免責事項と情報
BenchChemで提示されるすべての記事および製品情報は、情報提供を目的としています。BenchChemで購入可能な製品は、生体外研究のために特別に設計されています。生体外研究は、ラテン語の "in glass" に由来し、生物体の外で行われる実験を指します。これらの製品は医薬品または薬として分類されておらず、FDAから任何の医療状態、病気、または疾患の予防、治療、または治癒のために承認されていません。これらの製品を人間または動物に体内に導入する形態は、法律により厳格に禁止されています。これらのガイドラインに従うことは、研究と実験において法的および倫理的な基準の遵守を確実にするために重要です。