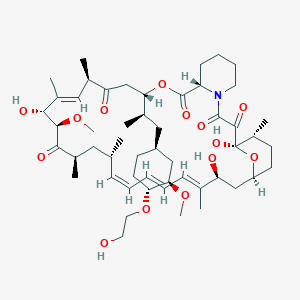
O-デスメチル エベロリムス (>80パーセント)
- 専門家チームからの見積もりを受け取るには、QUICK INQUIRYをクリックしてください。
- 品質商品を競争力のある価格で提供し、研究に集中できます。
説明
O-Desmethyl Everolimus is a derivative of Everolimus, a compound known for its immunosuppressive and anti-proliferative properties. Everolimus is widely used in the treatment of various cancers and in preventing organ transplant rejection. O-Desmethyl Everolimus is an important metabolite of Everolimus, formed through the demethylation process. This compound retains many of the pharmacological properties of its parent compound, making it a subject of interest in scientific research and pharmaceutical development.
科学的研究の応用
O-Desmethyl Everolimus has a wide range of scientific research applications, including:
Chemistry: Used as a reference compound in analytical chemistry for the study of Everolimus metabolism and degradation pathways.
Biology: Investigated for its effects on cellular processes, including cell growth, proliferation, and apoptosis.
Medicine: Explored for its potential therapeutic applications in cancer treatment and immunosuppression, similar to Everolimus.
Industry: Utilized in the development of new pharmaceutical formulations and drug delivery systems.
作用機序
Target of Action
O-Desmethyl Everolimus, like Everolimus, primarily targets the mammalian target of rapamycin (mTOR) . mTOR is a serine/threonine kinase that plays a crucial role in regulating cell growth, proliferation, and survival .
Mode of Action
O-Desmethyl Everolimus acts as an mTOR inhibitor . It binds with high affinity to the FK506 binding protein-12 (FKBP-12), forming a drug complex that inhibits the activation of mTOR . This inhibition disrupts several downstream processes, including protein synthesis and cell cycle progression, which are essential for cell growth and proliferation .
Biochemical Pathways
The main metabolic pathway of O-Desmethyl Everolimus is mediated by CYP3A4 and CYP3A5 , producing predominantly hydroxylated Everolimus metabolites . The mTOR pathway, which is frequently deregulated in cancer, is also affected by this compound .
Pharmacokinetics
The pharmacokinetics of O-Desmethyl Everolimus involve several factors. Everolimus clearance is significantly affected by the estimated glomerular filtration rate, concomitant use of fluconazole, sex, as well as the total daily dose of Everolimus . The individual apparent clearance of Everolimus can be moderately correlated with the dose per trough concentration ratio of tacrolimus in each patient .
Result of Action
The inhibition of mTOR by O-Desmethyl Everolimus leads to a decrease in cell growth, proliferation, and survival . This makes it a valuable tool in the treatment of various types of malignancies, including advanced renal cell carcinoma, hormone receptor-positive, HER2-negative breast cancer, and neuroendocrine tumors of pancreatic origin .
Action Environment
The action of O-Desmethyl Everolimus can be influenced by various environmental factors. For instance, higher Everolimus dose requirement is associated with co-administration of sodium-mycophenolic acid and the CYP3A5 expressor genotype, while a lower dose is required for patients receiving vitamin K antagonists . Metabolic profiles, which reflect the combined effect of genetic, environmental, and physiological factors on the individual drug response, can also affect the action of this compound .
生化学分析
Biochemical Properties
O-Desmethyl Everolimus, like Everolimus, is likely to interact with various enzymes, proteins, and other biomolecules in the mTOR pathway The specific interactions of O-Desmethyl Everolimus have not been fully elucidated
Cellular Effects
Everolimus has been shown to have significant effects on various types of cells and cellular processes . It influences cell function by impacting cell signaling pathways, gene expression, and cellular metabolism
Molecular Mechanism
Everolimus works by inhibiting the mTOR pathway, exerting its effects at the molecular level through binding interactions with biomolecules, enzyme inhibition or activation, and changes in gene expression
Temporal Effects in Laboratory Settings
Studies on Everolimus have shown changes in its effects over time, including information on the drug’s stability, degradation, and long-term effects on cellular function . Similar studies need to be conducted on O-Desmethyl Everolimus to understand its temporal effects.
Dosage Effects in Animal Models
The effects of O-Desmethyl Everolimus at different dosages in animal models have not been extensively studied. A study on Everolimus showed that it had neuroprotective effects in a rat model of intracerebral hemorrhage
Metabolic Pathways
Everolimus is known to impact glucose metabolism, affecting glycolysis and the pentose phosphate pathway
準備方法
Synthetic Routes and Reaction Conditions
The synthesis of O-Desmethyl Everolimus typically involves the demethylation of Everolimus. This process can be achieved using various chemical reagents and conditions. One common method involves the use of strong acids or bases to remove the methyl group from Everolimus. The reaction is usually carried out under controlled temperature and pressure conditions to ensure the desired product is obtained with high purity.
Industrial Production Methods
In an industrial setting, the production of O-Desmethyl Everolimus involves large-scale chemical reactors where Everolimus is subjected to demethylation. The process is optimized to maximize yield and purity while minimizing the production of unwanted by-products. Advanced purification techniques, such as chromatography, are employed to isolate O-Desmethyl Everolimus from the reaction mixture.
化学反応の分析
Types of Reactions
O-Desmethyl Everolimus undergoes various chemical reactions, including:
Oxidation: This reaction involves the addition of oxygen or the removal of hydrogen, often using oxidizing agents like hydrogen peroxide or potassium permanganate.
Reduction: This reaction involves the addition of hydrogen or the removal of oxygen, typically using reducing agents like sodium borohydride or lithium aluminum hydride.
Substitution: This reaction involves the replacement of one functional group with another, often using reagents like halogens or alkylating agents.
Common Reagents and Conditions
Oxidation: Hydrogen peroxide, potassium permanganate, and other oxidizing agents under acidic or basic conditions.
Reduction: Sodium borohydride, lithium aluminum hydride, and other reducing agents under controlled temperature and pressure.
Substitution: Halogens, alkylating agents, and other reagents under various conditions depending on the desired substitution.
Major Products Formed
The major products formed from these reactions depend on the specific reagents and conditions used. For example, oxidation of O-Desmethyl Everolimus can lead to the formation of hydroxylated derivatives, while reduction can produce more reduced forms of the compound. Substitution reactions can introduce new functional groups, leading to a variety of derivatives with different pharmacological properties.
類似化合物との比較
Similar Compounds
Everolimus: The parent compound, known for its immunosuppressive and anti-proliferative properties.
Sirolimus: Another mTOR inhibitor with similar pharmacological effects.
Zotarolimus: A derivative of Sirolimus, used in drug-eluting stents to prevent restenosis.
Uniqueness
O-Desmethyl Everolimus is unique in that it retains many of the pharmacological properties of Everolimus while potentially offering different pharmacokinetic and metabolic profiles. This makes it a valuable compound for further research and development, particularly in the context of personalized medicine and targeted therapies.
特性
IUPAC Name |
(1R,9S,12S,15R,16E,18R,19R,21R,23S,24Z,26E,28E,30S,32S,35R)-1,18,30-trihydroxy-12-[(2R)-1-[(1S,3R,4R)-4-(2-hydroxyethoxy)-3-methoxycyclohexyl]propan-2-yl]-19-methoxy-15,17,21,23,29,35-hexamethyl-11,36-dioxa-4-azatricyclo[30.3.1.04,9]hexatriaconta-16,24,26,28-tetraene-2,3,10,14,20-pentone |
Source
|
---|---|---|
Source | PubChem | |
URL | https://pubchem.ncbi.nlm.nih.gov | |
Description | Data deposited in or computed by PubChem | |
InChI |
InChI=1S/C52H81NO14/c1-31-15-11-10-12-16-32(2)41(55)29-39-20-18-37(7)52(62,67-39)49(59)50(60)53-22-14-13-17-40(53)51(61)66-44(34(4)27-38-19-21-43(65-24-23-54)45(28-38)63-8)30-42(56)33(3)26-36(6)47(58)48(64-9)46(57)35(5)25-31/h10-12,15-16,26,31,33-35,37-41,43-45,47-48,54-55,58,62H,13-14,17-25,27-30H2,1-9H3/b12-10+,15-11-,32-16+,36-26+/t31-,33-,34-,35-,37-,38+,39+,40+,41+,43-,44+,45-,47-,48+,52-/m1/s1 |
Source
|
Source | PubChem | |
URL | https://pubchem.ncbi.nlm.nih.gov | |
Description | Data deposited in or computed by PubChem | |
InChI Key |
IENBGEQGDJTPQU-YUPAPZQESA-N |
Source
|
Source | PubChem | |
URL | https://pubchem.ncbi.nlm.nih.gov | |
Description | Data deposited in or computed by PubChem | |
Canonical SMILES |
CC1CCC2CC(C(=CC=CC=CC(CC(C(=O)C(C(C(=CC(C(=O)CC(OC(=O)C3CCCCN3C(=O)C(=O)C1(O2)O)C(C)CC4CCC(C(C4)OC)OCCO)C)C)O)OC)C)C)C)O |
Source
|
Source | PubChem | |
URL | https://pubchem.ncbi.nlm.nih.gov | |
Description | Data deposited in or computed by PubChem | |
Isomeric SMILES |
C[C@@H]1CC[C@H]2C[C@@H](/C(=C/C=C/C=C\[C@H](C[C@H](C(=O)[C@@H]([C@@H](/C(=C/[C@H](C(=O)C[C@H](OC(=O)[C@@H]3CCCCN3C(=O)C(=O)[C@@]1(O2)O)[C@H](C)C[C@@H]4CC[C@H]([C@@H](C4)OC)OCCO)C)/C)O)OC)C)C)/C)O |
Source
|
Source | PubChem | |
URL | https://pubchem.ncbi.nlm.nih.gov | |
Description | Data deposited in or computed by PubChem | |
Molecular Formula |
C52H81NO14 |
Source
|
Source | PubChem | |
URL | https://pubchem.ncbi.nlm.nih.gov | |
Description | Data deposited in or computed by PubChem | |
Molecular Weight |
944.2 g/mol |
Source
|
Source | PubChem | |
URL | https://pubchem.ncbi.nlm.nih.gov | |
Description | Data deposited in or computed by PubChem | |
試験管内研究製品の免責事項と情報
BenchChemで提示されるすべての記事および製品情報は、情報提供を目的としています。BenchChemで購入可能な製品は、生体外研究のために特別に設計されています。生体外研究は、ラテン語の "in glass" に由来し、生物体の外で行われる実験を指します。これらの製品は医薬品または薬として分類されておらず、FDAから任何の医療状態、病気、または疾患の予防、治療、または治癒のために承認されていません。これらの製品を人間または動物に体内に導入する形態は、法律により厳格に禁止されています。これらのガイドラインに従うことは、研究と実験において法的および倫理的な基準の遵守を確実にするために重要です。