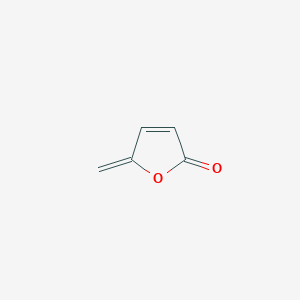
プロトアネモニン
概要
説明
Protoanemonin belongs to the class of organic compounds known as butenolides. These are dihydrofurans with a carbonyl group at the C2 carbon atom. Protoanemonin is soluble (in water) and an extremely weak basic (essentially neutral) compound (based on its pKa).
Protoanemonin is a butenolide.
科学的研究の応用
抗菌作用
プロトアネモニンは、抗菌作用を持つことが確認されています。グラム陽性菌とグラム陰性菌の両方の細菌株に対して有効です。 この化合物は、細菌の細胞壁を破壊し、細胞内の酵素活性を阻害することで、細菌の細胞死をもたらします .
抗真菌活性
研究によると、プロトアネモニンは抗真菌活性を示しており、真菌感染症の治療のための候補物質となっています。 そのメカニズムは、真菌の細胞膜の完全性と代謝過程の干渉に関与しています .
抗炎症作用
抗炎症作用において、プロトアネモニンは炎症性経路を調節する能力から有望視されています。 プロトアネモニンは、炎症性サイトカインの産生を阻害することで、炎症を軽減することができます .
抗がん作用の可能性
プロトアネモニンは、抗がん作用の可能性について研究されています。がん細胞のアポトーシスを誘導し、腫瘍の増殖を阻害することができます。 研究によると、がん細胞の細胞周期に影響を与え、細胞死につながる可能性が示唆されています .
抗原虫作用
この化合物は、特にマラリアなどの病気を引き起こす寄生虫に対する抗原虫作用を示しています。 プロトアネモニンは、これらの寄生虫のライフサイクルを阻害することで、新しい抗マラリア薬の可能性を提供する可能性があります .
創傷治癒
プロトアネモニンは、線維芽細胞の増殖とコラーゲンの合成を促進することにより、創傷治癒に貢献する可能性があります。これらの要素は、組織の修復と再生に不可欠です .
鎮痛作用
伝統的に、プロトアネモニンを含む化合物は、その鎮痛作用のために使用されてきました。 プロトアネモニンは、中枢神経系の痛み信号に対する反応を調節することで、痛みを軽減すると考えられています .
免疫調節作用
最後に、プロトアネモニンは、免疫調節作用を示しています。 プロトアネモニンは、免疫系の反応に影響を与える可能性があり、自己免疫疾患の治療を支援したり、ワクチンの有効性を高めたりする可能性があります .
Safety and Hazards
Contact with a wounded plant containing Protoanemonin can cause itch, rashes, or blistering on contact with the skin or mucosa . Ingesting the toxin can cause nausea, vomiting, dizziness, spasms, acute hepatitis, jaundice, or paralysis . The lethal dose or concentration (LD50) in mice is 190 mg·kg−1 .
将来の方向性
Protoanemonin significantly reduced the expression of genes and secretion of proteins known to be under control of quorum sensing in P.aeruginosa . Moreover, it activated genes and gene products involved in iron starvation response . This suggests that Protoanemonin could have potential applications in addressing conditions such as arthritis, cerebral ischemia, and ulcerative colitis .
作用機序
Target of Action
Protoanemonin, a toxin found in all plants of the buttercup family (Ranunculaceae), exhibits a wide spectrum of biological activity against various microorganisms . It has been studied as an antifungal agent on the dermatophyte Microsporum cookei . The primary targets of this molecule appear to be cytoplasmic microtubules, which contain a great number of sulfhydryl (ASH) groups .
Mode of Action
It is suggested that protoanemonin inhibits growth by reacting with sulfhydryl enzymes . It significantly reduces the expression of genes and secretion of proteins known to be under control of quorum sensing in Pseudomonas aeruginosa .
Biochemical Pathways
When the plant is wounded or macerated, the unstable glucoside found in the plant, ranunculin, is enzymatically broken down into glucose and the toxic protoanemonin . Protoanemonin significantly activates genes and gene products involved in iron starvation response .
Pharmacokinetics
The inherent instability of ranunculin and the rapid dimerization of protoanemonin render them unsuitable for use in biological assays . Anemonin, the product of protoanemonin’s cyclodimerization, stands out as the optimal molecule for bioassays . More detailed pharmacokinetic studies are needed to understand the ADME properties of protoanemonin.
Result of Action
Protoanemonin exhibits a wide range of biological activities. It inhibits the growth of Gram-positive and Gram-negative bacteria, fungi, and two protozoa . The degree of inhibition varies widely . It also causes mitochondria of root tip cells of Zea mays to disintegrate and affects mitotic division of meristematic tissue .
Action Environment
The action of protoanemonin can be influenced by environmental factors. For instance, it is produced by pseudomonads Pseudomonas sp. B13 and Pseudomonas reinekei MT1 as a blind end in the biodegradation of organochloride xenobiotics
生化学分析
Biochemical Properties
Protoanemonin exhibits a wide spectrum of biological activity. It has been shown to inhibit the growth of Gram-positive and Gram-negative bacteria, and of fungi . Protoanemonin inhibits growth by reacting with sulfhydryl enzymes . The degree of inhibition varies widely, and growth of two protozoa was inhibited in dilutions of protoanemonin ranging from 1/200,000 to 1/600,000 .
Cellular Effects
Protoanemonin has a significant impact on various types of cells. For instance, a dilution of 10^-6 of the antibiotic was toxic to chicken epithelial and fibroblastic cells in tissue culture . Mitochondria of root tip cells of Zea mays exposed to protoanemonin disintegrated and mitotic division of meristematic tissue was affected . Protoanemonin was reported to have a cytopathogenic effect on both normal and tumor tissue cultures .
Molecular Mechanism
The molecular mechanism of protoanemonin’s action involves its interaction with sulfhydryl enzymes. Cysteine caused a reduction in bacteriostatic properties of anemonin . Elongation of Avena coleoptiles was inhibited by protoanemonin but the inhibition was reversed by dimercaptopropanol .
Temporal Effects in Laboratory Settings
The inherent instability of ranunculin and the rapid dimerization of protoanemonin render them unsuitable for use in biological assays . Conversely, anemonin stands out as the optimal molecule for bioassays and demonstrates diverse biological properties, including anti-inflammatory, anti-infective, and anti-oxidant effects .
Dosage Effects in Animal Models
Toxicity experiments with guinea pigs and mice showed that protoanemonin was lethal if doses were administered to give titers required to inhibit bacterial growth . The LD50 of protoanemonin in male Swiss albino mice was 190 mg/kg .
Metabolic Pathways
When the plant is wounded or macerated, the unstable glucoside found in the plant, ranunculin, is enzymatically broken down into glucose and the toxic protoanemonin . When drying the plant, protoanemonin comes into contact with air and dimerizes to anemonin, which is further hydrolyzed to a non-toxic dicarboxylic acid .
特性
IUPAC Name |
5-methylidenefuran-2-one | |
---|---|---|
Source | PubChem | |
URL | https://pubchem.ncbi.nlm.nih.gov | |
Description | Data deposited in or computed by PubChem | |
InChI |
InChI=1S/C5H4O2/c1-4-2-3-5(6)7-4/h2-3H,1H2 | |
Source | PubChem | |
URL | https://pubchem.ncbi.nlm.nih.gov | |
Description | Data deposited in or computed by PubChem | |
InChI Key |
RNYZJZKPGHQTJR-UHFFFAOYSA-N | |
Source | PubChem | |
URL | https://pubchem.ncbi.nlm.nih.gov | |
Description | Data deposited in or computed by PubChem | |
Canonical SMILES |
C=C1C=CC(=O)O1 | |
Source | PubChem | |
URL | https://pubchem.ncbi.nlm.nih.gov | |
Description | Data deposited in or computed by PubChem | |
Molecular Formula |
C5H4O2 | |
Source | PubChem | |
URL | https://pubchem.ncbi.nlm.nih.gov | |
Description | Data deposited in or computed by PubChem | |
DSSTOX Substance ID |
DTXSID10148346 | |
Record name | Protoanemonin | |
Source | EPA DSSTox | |
URL | https://comptox.epa.gov/dashboard/DTXSID10148346 | |
Description | DSSTox provides a high quality public chemistry resource for supporting improved predictive toxicology. | |
Molecular Weight |
96.08 g/mol | |
Source | PubChem | |
URL | https://pubchem.ncbi.nlm.nih.gov | |
Description | Data deposited in or computed by PubChem | |
CAS No. |
108-28-1 | |
Record name | Protoanemonin | |
Source | CAS Common Chemistry | |
URL | https://commonchemistry.cas.org/detail?cas_rn=108-28-1 | |
Description | CAS Common Chemistry is an open community resource for accessing chemical information. Nearly 500,000 chemical substances from CAS REGISTRY cover areas of community interest, including common and frequently regulated chemicals, and those relevant to high school and undergraduate chemistry classes. This chemical information, curated by our expert scientists, is provided in alignment with our mission as a division of the American Chemical Society. | |
Explanation | The data from CAS Common Chemistry is provided under a CC-BY-NC 4.0 license, unless otherwise stated. | |
Record name | Protoanemonin | |
Source | ChemIDplus | |
URL | https://pubchem.ncbi.nlm.nih.gov/substance/?source=chemidplus&sourceid=0000108281 | |
Description | ChemIDplus is a free, web search system that provides access to the structure and nomenclature authority files used for the identification of chemical substances cited in National Library of Medicine (NLM) databases, including the TOXNET system. | |
Record name | Protoanemonin | |
Source | EPA DSSTox | |
URL | https://comptox.epa.gov/dashboard/DTXSID10148346 | |
Description | DSSTox provides a high quality public chemistry resource for supporting improved predictive toxicology. | |
Record name | 5-methylenefuran-2(5H)-one | |
Source | European Chemicals Agency (ECHA) | |
URL | https://echa.europa.eu/substance-information/-/substanceinfo/100.003.244 | |
Description | The European Chemicals Agency (ECHA) is an agency of the European Union which is the driving force among regulatory authorities in implementing the EU's groundbreaking chemicals legislation for the benefit of human health and the environment as well as for innovation and competitiveness. | |
Explanation | Use of the information, documents and data from the ECHA website is subject to the terms and conditions of this Legal Notice, and subject to other binding limitations provided for under applicable law, the information, documents and data made available on the ECHA website may be reproduced, distributed and/or used, totally or in part, for non-commercial purposes provided that ECHA is acknowledged as the source: "Source: European Chemicals Agency, http://echa.europa.eu/". Such acknowledgement must be included in each copy of the material. ECHA permits and encourages organisations and individuals to create links to the ECHA website under the following cumulative conditions: Links can only be made to webpages that provide a link to the Legal Notice page. | |
Record name | PROTOANEMONIN | |
Source | FDA Global Substance Registration System (GSRS) | |
URL | https://gsrs.ncats.nih.gov/ginas/app/beta/substances/66FQZ1A5SO | |
Description | The FDA Global Substance Registration System (GSRS) enables the efficient and accurate exchange of information on what substances are in regulated products. Instead of relying on names, which vary across regulatory domains, countries, and regions, the GSRS knowledge base makes it possible for substances to be defined by standardized, scientific descriptions. | |
Explanation | Unless otherwise noted, the contents of the FDA website (www.fda.gov), both text and graphics, are not copyrighted. They are in the public domain and may be republished, reprinted and otherwise used freely by anyone without the need to obtain permission from FDA. Credit to the U.S. Food and Drug Administration as the source is appreciated but not required. | |
Retrosynthesis Analysis
AI-Powered Synthesis Planning: Our tool employs the Template_relevance Pistachio, Template_relevance Bkms_metabolic, Template_relevance Pistachio_ringbreaker, Template_relevance Reaxys, Template_relevance Reaxys_biocatalysis model, leveraging a vast database of chemical reactions to predict feasible synthetic routes.
One-Step Synthesis Focus: Specifically designed for one-step synthesis, it provides concise and direct routes for your target compounds, streamlining the synthesis process.
Accurate Predictions: Utilizing the extensive PISTACHIO, BKMS_METABOLIC, PISTACHIO_RINGBREAKER, REAXYS, REAXYS_BIOCATALYSIS database, our tool offers high-accuracy predictions, reflecting the latest in chemical research and data.
Strategy Settings
Precursor scoring | Relevance Heuristic |
---|---|
Min. plausibility | 0.01 |
Model | Template_relevance |
Template Set | Pistachio/Bkms_metabolic/Pistachio_ringbreaker/Reaxys/Reaxys_biocatalysis |
Top-N result to add to graph | 6 |
Feasible Synthetic Routes
試験管内研究製品の免責事項と情報
BenchChemで提示されるすべての記事および製品情報は、情報提供を目的としています。BenchChemで購入可能な製品は、生体外研究のために特別に設計されています。生体外研究は、ラテン語の "in glass" に由来し、生物体の外で行われる実験を指します。これらの製品は医薬品または薬として分類されておらず、FDAから任何の医療状態、病気、または疾患の予防、治療、または治癒のために承認されていません。これらの製品を人間または動物に体内に導入する形態は、法律により厳格に禁止されています。これらのガイドラインに従うことは、研究と実験において法的および倫理的な基準の遵守を確実にするために重要です。