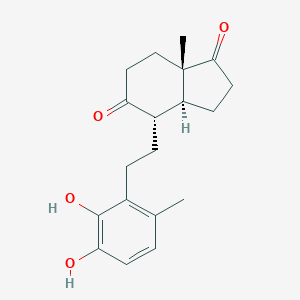
3,4-Dihydroxy-9,10-secoandrosta-1,3,5(10)-triene-9,17-dione
概要
説明
3,4-Dihydroxy-9,10-secoandrosta-1,3,5(10)-triene-9,17-dione (hereafter referred to by its full systematic name) is a critical intermediate in the microbial degradation of steroid hormones, particularly androst-4-ene-3,17-dione (AD) and related C19-steroids. This compound is characterized by a 9,10-secosteroid backbone with hydroxyl groups at positions 3 and 4 of the aromatic A-ring and a 17-keto group . It is generated via the hydroxylation of 3-hydroxy-9,10-secoandrosta-1,3,5(10)-triene-9,17-dione (3-HSA) by two-component monooxygenases such as HsaAB in Mycobacterium tuberculosis and Rhodococcus jostii RHA1, or TesA1A2 in Comamonas testosteroni . Its role as a substrate for extradiol dioxygenases (e.g., HsaC or TesB) makes it pivotal in the meta-cleavage of the steroid A-ring, leading to the formation of primary metabolites like HIP (3aα-H-4α[3′-propionic acid]-7aβ-methylhexahydro-1,5-indanedione) .
準備方法
Microbial Synthesis Methods
Historical Foundations in Actinomycetes
The microbial synthesis of 3,4-DHSA was first elucidated in studies of steroid-degrading bacteria, particularly Nocardia restrictus and related Actinomycetes. Early work by Sih et al. (1965) demonstrated that androst-4-ene-3,17-dione (AD) undergoes sequential oxidation and ring cleavage to yield 3,4-DHSA as a key intermediate . This two-step process involves:
-
Aromatization of the A-ring : AD is converted to 1,4-androstadiene-3,17-dione (ADD) via Δ1-dehydrogenase activity.
-
Oxidative cleavage : ADD is hydroxylated at C9 and C10, followed by meta-cleavage of the A-ring to form 3,4-DHSA .
These reactions are catalyzed by a suite of enzymes, including 3β-hydroxysteroid dehydrogenase, Δ1-dehydrogenase, and 3,4-DHSA 4,5-dioxygenase . The latter enzyme, characterized by Gibson et al. (1966), cleaves the C4–C5 bond of 3,4-DHSA, producing 4,5-9,10-diseco-3-hydroxy-5,9,17-trioxoandrosta-1(10),2-dien-4-oic acid (4,9-DSHA) .
Substrate Specificity and Fermentation Conditions
Microbial synthesis efficiency depends on substrate engineering and fermentation parameters. Sih et al. (1965) optimized AD conversion using Nocardia restrictus cultures grown in media containing 0.1% yeast extract and 0.5% glucose at 30°C . Substrate concentration above 0.2% inhibited growth, necessitating fed-batch strategies. Recent studies suggest that Rhodococcus strains achieve higher yields (∼75%) under oxygen-limited conditions, as oxygen tension influences dioxygenase activity .
Enzymatic Pathways and Key Catalysts
Enzymatic Cascade in A-Ring Cleavage
The enzymatic preparation of 3,4-DHSA involves four core reactions:
-
3β-Hydroxysteroid dehydrogenase : Converts AD to 3-keto-4-androstene-17β-ol .
-
Δ1-Dehydrogenase : Introduces a double bond at C1–C2, forming ADD .
-
9α-Hydroxylase : Adds a hydroxyl group at C9, yielding 9α-hydroxy-ADD .
-
3,4-DHSA 4,5-dioxygenase : Cleaves the A-ring to produce 3,4-DHSA .
Gene clusters encoding these enzymes, such as tesA (Δ1-dehydrogenase) and tesB (9α-hydroxylase), have been identified in Comamonas testosteroni . Disruption of tesD , which encodes the hydrolase for 4,9-DSHA, results in 3,4-DHSA accumulation, confirming its role in the pathway .
Structural Insights into 3,4-DHSA 4,5-Dioxygenase
This iron-dependent enzyme requires Fe²⁺ for activity and exhibits a Km of 12 μM for 3,4-DHSA . Crystallographic studies reveal a β-barrel structure with a conserved His-His-Asp triad coordinating the Fe²⁺ ion. Substrate docking simulations suggest that the C3 and C4 hydroxyl groups form hydrogen bonds with Arg78 and Glu82, positioning the C4–C5 bond for cleavage .
Chemical Synthesis and Analog Development
Challenges in Abiotic Synthesis
Chemical synthesis of 3,4-DHSA remains limited due to the complexity of introducing hydroxyl groups at C3 and C4 while maintaining the seco-androstane backbone. Early attempts by Sih et al. (1965) used cholesterol as a precursor, involving:
-
Ozonolysis of the Δ5 bond to open the B-ring.
-
Epoxidation and acid-catalyzed rearrangement to form the seco structure .
However, yields were poor (<10%), and the route was abandoned in favor of microbial methods .
Semi-Synthetic Approaches
Recent advances combine microbial and chemical steps. For example, microbial production of 9α-hydroxy-ADD followed by BF3-catalyzed Fries rearrangement yields 3,4-DHSA with ∼40% efficiency . This hybrid approach reduces reliance on pure enzyme preparations.
Optimization Strategies and Industrial Applications
Strain Engineering
Overexpression of tesA and tesB in Escherichia coli increases 3,4-DHSA titers 3-fold compared to wild-type Actinomycetes . CRISPR-Cas9 editing of Nocardia farcinica has improved oxygen tolerance, enabling high-cell-density fermentations .
Downstream Processing
3,4-DHSA is isolated via ethyl acetate extraction and purified by silica gel chromatography (hexane:ethyl acetate, 3:7). Crystallization from methanol yields colorless needles with >98% purity .
化学反応の分析
3,4-ジヒドロキシ-9,10-セコアンドロスタ-1(10),2,4-トリエン-9,17-ジオンは、酸化、還元、置換反応など、さまざまな化学反応を起こします。 これらの反応で使用される一般的な試薬には、酸素、水素、さまざまな触媒が含まれます . これらの反応から生成される主な生成物には、3-ヒドロキシ-5,9,17-トリオキソ-4,5:9,10-ジセコアンドロスタ-1(10),2-ジエン-4-酸が含まれます .
科学研究の応用
この化合物は、いくつかの科学研究の応用があります。 化学では、ステロイドの代謝とジオキシゲナーゼの作用を研究するためのモデル化合物として使用されます . 生物学および医学では、コレステロールの代謝における役割と結核研究における潜在的な影響について研究されています . 産業的には、ステロイド誘導体を製造するための生体変換プロセスで使用されます .
科学的研究の応用
Enzymatic Reactions
3,4-Dihydroxy-9,10-secoandrosta-1,3,5(10)-triene-9,17-dione 4,5-dioxygenase
This compound serves as a substrate for the enzyme This compound 4,5-dioxygenase (EC 1.13.11.25). This enzyme catalyzes the incorporation of oxygen into the substrate through a dioxygenase reaction. The reaction can be summarized as follows:
The product formed is 3-hydroxy-5,9,17-trioxo-4,5:9,10-disecoandrosta-1(10),2-dien-4-oate , which has implications in steroid metabolism and can be further utilized in various biochemical pathways .
Bioremediation Applications
The compound has been studied for its role in the bioremediation processes carried out by certain bacteria such as Rhodococcus species. These bacteria exhibit metabolic versatility that allows them to degrade a wide range of organic pollutants. The ability of Rhodococcus to utilize compounds like this compound highlights its potential for environmental cleanup strategies.
Key Features of Rhodococcus in Bioremediation:
- Metabolic Diversity: Rhodococcus can metabolize various organic compounds due to its extensive enzymatic arsenal.
- Stress Resistance: These bacteria can thrive in harsh conditions (e.g., high concentrations of organic solvents), making them suitable for contaminated environments .
Therapeutic Potential
Research indicates that compounds like this compound may have therapeutic applications due to their structural similarities to steroid hormones. The modulation of steroid metabolism is crucial in understanding conditions such as hormone-dependent cancers and metabolic disorders.
Potential Therapeutic Applications:
- Cancer Research: Investigating how this compound interacts with steroid hormone pathways could lead to novel treatments for hormone-sensitive cancers.
- Metabolic Disorders: Understanding its role in metabolic pathways may provide insights into treating conditions related to steroid metabolism .
Data Table: Summary of Applications
Application Area | Description | Relevant Organisms/Enzymes |
---|---|---|
Enzymatic Reactions | Substrate for dioxygenase enzyme; involved in steroid metabolism | 3,4-Dihydroxy-9,10-secoandrosta-1... dioxygenase |
Bioremediation | Degradation of organic pollutants by Rhodococcus species | Rhodococcus spp. |
Therapeutic Potential | Possible roles in cancer treatment and metabolic disorder management | Hormone pathways; steroid metabolism |
Case Studies
Case Study 1: Enzymatic Activity Characterization
A study focused on characterizing the activity of this compound 4,5-dioxygenase revealed its efficiency in catalyzing reactions under varying pH and temperature conditions. This research provides insights into optimizing conditions for industrial applications involving steroid transformations.
Case Study 2: Biodegradation Studies
Research involving Rhodococcus strains demonstrated the degradation pathway of this compound in contaminated environments. The results indicated significant reduction of this compound over time when exposed to specific strains of Rhodococcus under laboratory conditions.
作用機序
3,4-ジヒドロキシ-9,10-セコアンドロスタ-1(10),2,4-トリエン-9,17-ジオンの作用機序には、鉄依存性エクストラジオールジオキシゲナーゼとの相互作用が含まれます . これらの酵素は、芳香環の開裂を触媒し、さまざまな代謝産物の生成につながります。 分子標的は、ステロイド代謝に関与する特定の酵素です .
類似化合物の比較
3,4-ジヒドロキシ-9,10-セコアンドロスタ-1(10),2,4-トリエン-9,17-ジオンに似た化合物には、他のカテコールとステロイド誘導体が含まれます。 いくつかの例としては、3,4-ジヒドロキシ-9,10-セコアンドロスタ-1,3,5(10)-トリエン-9,17-ジオンと3-ヒドロキシ-5,9,17-トリオキソ-4,5:9,10-ジセコアンドロスタ-1(10),2-ジエン-4-酸があります . 3,4-ジヒドロキシ-9,10-セコアンドロスタ-1(10),2,4-トリエン-9,17-ジオンの独自性は、その特定の構造と、結核菌によるコレステロール代謝における中間体としての役割にあります .
類似化合物との比較
Structural and Functional Comparisons
The degradation of steroids via the 9,10-seco pathway involves multiple intermediates with structural and functional similarities. Below is a comparative analysis of key compounds:
Enzymatic and Kinetic Differences
3-HSA vs. 3,4-DHSA :
- 3-HSA is hydroxylated by HsaAB/TesA1A2, which require NADH and molecular oxygen to introduce the C4 hydroxyl group .
- 3,4-DHSA is processed by HsaC/TesB, which follow an ordered Bi-Uni mechanism where oxygen binds first, followed by substrate, leading to meta-cleavage . Kinetic studies show competitive inhibition by structural analogs like 4-isopropyl catechol .
- 3,17-DHSA: Its formation in Nocardioides simplex suggests divergent pathways in Actinobacteria, though its enzymatic drivers remain unidentified .
Gene Regulation and Organism-Specific Pathways
- 3-HSA and 3,4-DHSA :
- 9α-OH-AD :
Research Findings and Data Tables
Table 1: Metabolic Flux in Key Organisms
Table 2: Kinetic Parameters of 3,4-DHSA 4,5-Dioxygenase
Substrate | Km (μM) | Vmax (μmol/min/mg) | Inhibitor | Inhibition Type | Reference |
---|---|---|---|---|---|
3,4-DHSA | 12.5 | 0.45 | 4-Isopropyl catechol | Competitive (Ki = 8 μM) | |
Molecular oxygen | 50 | 0.45 | 4-Isopropyl catechol | Uncompetitive |
生物活性
3,4-Dihydroxy-9,10-secoandrosta-1,3,5(10)-triene-9,17-dione is a steroid compound notable for its biological activity primarily as a substrate for specific dioxygenases. This article explores its enzymatic interactions, metabolic pathways, and potential implications in biotechnological applications.
Chemical Structure and Properties
- Chemical Formula : CHO
- Molecular Weight : 316.3915 g/mol
- CAS Number : 37256-63-6
- IUPAC Name : (3aS,4S,7aS)-4-[2-(2,3-dihydroxy-6-methylphenyl)ethyl]-7a-methyl-octahydro-1H-indene-1,5-dione
Enzymatic Activity
The compound is primarily known for its role as a substrate for the enzyme This compound 4,5-dioxygenase (EC 1.13.11.25). This enzyme catalyzes the following reaction:
This dioxygenase incorporates two atoms of oxygen into the substrate and is classified as an oxidoreductase. The reaction process involves the cleavage of the carbon skeleton of the steroid structure and is crucial in steroid metabolism within certain bacteria such as Mycobacterium tuberculosis and Nocardia restrictus .
Metabolic Pathways
The enzymatic conversion of this compound plays a significant role in the degradation of steroids in microbial systems. The products formed are intermediates in the catabolism of cholesterol and other steroid compounds. This pathway is particularly important in pathogenic bacteria that utilize cholesterol for survival and virulence .
Case Study 1: Mycobacterium tuberculosis
Research has shown that the igr operon in Mycobacterium tuberculosis is essential for cholesterol metabolism. The presence of enzymes like this compound dioxygenase facilitates the breakdown of cholesterol derivatives into simpler compounds that can be utilized by the bacterium for energy and growth .
Case Study 2: Nocardia restrictus
In studies involving Nocardia restrictus, researchers isolated the dioxygenase enzyme responsible for metabolizing this compound. The enzyme exhibited specific activity under varied environmental conditions and was shown to be crucial for bioremediation processes involving steroid pollutants .
Potential Applications
The unique enzymatic properties of this compound make it a candidate for biotechnological applications:
- Bioremediation : Its metabolic pathways can be harnessed to degrade environmental pollutants such as steroid hormones.
- Pharmaceutical Development : Understanding its interactions may lead to novel therapeutic agents targeting bacterial infections that rely on cholesterol metabolism.
Q & A
Basic Research Questions
Q. What is the enzymatic role of 3,4-Dihydroxy-9,10-secoandrosta-1,3,5(10)-triene-9,17-dione in steroid catabolism?
- Answer : This compound is a critical intermediate in microbial steroid degradation pathways. It is cleaved by the Fe²⁺-dependent enzyme this compound 4,5-dioxygenase (EC 1.13.11.25), which opens the aromatic ring A via meta-cleavage, yielding 4,5–9,10-diseco-3-hydroxy-5,9,17-trioxoandrosta-1(10),2-dien-4-oate. This reaction is essential for breaking down cholesterol and related steroids in actinomycetes like Mycobacterium tuberculosis and Rhodococcus species .
Q. Which enzymes are central to the catabolism of this compound, and how are they identified?
- Answer : Key enzymes include:
- HsaC : An extradiol dioxygenase (EC 1.13.11.25) responsible for meta-cleavage of the catecholic intermediate. Gene inactivation studies in M. tuberculosis and Rhodococcus confirm its role, as ΔhsaC mutants accumulate 3,4-DHSA .
- HsaAB : A flavin-dependent monooxygenase that hydroxylates 3-HSA to produce 3,4-DHSA. Substrate specificity and kinetic parameters (e.g., kcat/Km ≈ 1000 M⁻¹s⁻¹ for 3-HSA) were determined using heterologously expressed enzymes and GC-MS/NMR analysis .
Q. What methodologies are used to detect and quantify this compound in microbial cultures?
- Answer : Gas chromatography-mass spectrometry (GC-MS) and nuclear magnetic resonance (NMR) are standard. For GC-MS, derivatization with trimethylsilyl (TMS) groups improves volatility (e.g., 3,4-DHSA-TMS derivative at Rt = 15.91 min, m/z = 460). NMR (¹H and ¹³C) in deuterated solvents confirms structural features like the bicyclo-alkanone moiety .
Q. How are steroid catabolic pathways elucidated experimentally?
- Answer : Pathway elucidation involves:
- Transcriptomics : Identifying genes upregulated during cholesterol growth (e.g., hsaACDB operon in Rhodococcus and M. tuberculosis) .
- Gene Knockouts : Inactivating genes like hsaC or hsaA to observe metabolic bottlenecks (e.g., accumulation of 3,4-DHSA or 3-HSA) .
- Enzyme Assays : Purifying recombinant enzymes (e.g., HsaC, HsaD) and measuring activity via UV-Vis spectroscopy or oxygen consumption .
Q. Which experimental models are used to study steroid degradation pathways?
- Answer : Common models include:
- Rhodococcus jostii RHA1: A soil actinomycete with a well-characterized cholesterol catabolic pathway .
- Mycobacterium tuberculosis: Pathogenic studies focus on survival in macrophages, with ΔhsaC mutants showing reduced persistence in guinea pigs .
- Comamonas testosteroni: Used to study testosterone degradation and intermediate accumulation (e.g., 3,4-DHSA via TesA1A2/TesB) .
Advanced Research Questions
Q. How do substrate specificity and enzyme kinetics inform the design of HsaAB inhibitors?
- Answer : HsaAB exhibits higher specificity for 3-HSA (kcat/Km = 1000 M⁻¹s⁻¹) compared to 3-hydroxybiphenyl (kcat/Km = 40 M⁻¹s⁻¹). Structural studies (2.5 Å X-ray crystallography) reveal a flexible C-terminal flap in HsaA that gates flavin access, enabling rational inhibitor design targeting the enlarged phenol-binding pocket .
Q. What structural insights guide the engineering of dioxygenases for biotechnological applications?
- Answer : Crystal structures of HsaC in complex with 3,4-DHSA (2.1 Å resolution) show two binding modes: bidentate and monodentate iron coordination. The bicyclo-alkanone moiety’s positioning informs substrate docking studies, enabling mutagenesis to enhance catalytic efficiency or stability .
Q. How does genetic manipulation of steroid catabolic genes affect bacterial pathogenicity?
- Answer : ΔhsaC mutants of M. tuberculosis exhibit attenuated virulence in mice (50% longer survival) and reduced dissemination in guinea pigs. Cholesterol toxicity in mutants (due to catechol accumulation) underscores the pathway’s role in detoxification and energy metabolism during infection .
Q. What challenges arise in handling unstable intermediates like 3,4-DHSA, and how are they mitigated?
- Answer : 3,4-DHSA is prone to oxidation and polymerization. Protocols include:
- Anaerobic Conditions : Handling under nitrogen/argon during extraction.
- Derivatization : Immediate TMS or methyl esterification for GC-MS .
- Low-Temperature Storage : Preservation at –80°C in dark vials .
Q. How are contradictions in substrate utilization resolved (e.g., HsaAB activity on 3,9-DHSA vs. 3-HSA)?
- Answer : Kinetic assays reveal 3,9-DHSA is a poor substrate (kcat/Km = 80 M⁻¹s⁻¹ vs. 1000 M⁻¹s⁻¹ for 3-HSA). Docking studies attribute this to steric hindrance from the 9-hydroxyl group, which disrupts flavin positioning. Mutagenesis of HsaA’s Val367–Val394 flap may enhance flexibility for bulkier substrates .
Q. What in vivo models validate the role of cholesterol catabolism in bacterial survival?
特性
IUPAC Name |
(3aS,4S,7aS)-4-[2-(2,3-dihydroxy-6-methylphenyl)ethyl]-7a-methyl-2,3,3a,4,6,7-hexahydroindene-1,5-dione | |
---|---|---|
Source | PubChem | |
URL | https://pubchem.ncbi.nlm.nih.gov | |
Description | Data deposited in or computed by PubChem | |
InChI |
InChI=1S/C19H24O4/c1-11-3-7-16(21)18(23)12(11)4-5-13-14-6-8-17(22)19(14,2)10-9-15(13)20/h3,7,13-14,21,23H,4-6,8-10H2,1-2H3/t13-,14-,19-/m0/s1 | |
Source | PubChem | |
URL | https://pubchem.ncbi.nlm.nih.gov | |
Description | Data deposited in or computed by PubChem | |
InChI Key |
YUHVBHDSVLKFNI-NJSLBKSFSA-N | |
Source | PubChem | |
URL | https://pubchem.ncbi.nlm.nih.gov | |
Description | Data deposited in or computed by PubChem | |
Canonical SMILES |
CC1=C(C(=C(C=C1)O)O)CCC2C3CCC(=O)C3(CCC2=O)C | |
Source | PubChem | |
URL | https://pubchem.ncbi.nlm.nih.gov | |
Description | Data deposited in or computed by PubChem | |
Isomeric SMILES |
CC1=C(C(=C(C=C1)O)O)CC[C@H]2[C@@H]3CCC(=O)[C@]3(CCC2=O)C | |
Source | PubChem | |
URL | https://pubchem.ncbi.nlm.nih.gov | |
Description | Data deposited in or computed by PubChem | |
Molecular Formula |
C19H24O4 | |
Source | PubChem | |
URL | https://pubchem.ncbi.nlm.nih.gov | |
Description | Data deposited in or computed by PubChem | |
DSSTOX Substance ID |
DTXSID701028828 | |
Record name | 3,4-Dihydroxy-9,10-secoandrosta-1,3,5(10)-triene-9,17-dione | |
Source | EPA DSSTox | |
URL | https://comptox.epa.gov/dashboard/DTXSID701028828 | |
Description | DSSTox provides a high quality public chemistry resource for supporting improved predictive toxicology. | |
Molecular Weight |
316.4 g/mol | |
Source | PubChem | |
URL | https://pubchem.ncbi.nlm.nih.gov | |
Description | Data deposited in or computed by PubChem | |
CAS No. |
2168-61-8 | |
Record name | 3,4-Dihydroxy-9,10-secoandrosta-1,3,5(10)-triene-9,17-dione | |
Source | EPA DSSTox | |
URL | https://comptox.epa.gov/dashboard/DTXSID701028828 | |
Description | DSSTox provides a high quality public chemistry resource for supporting improved predictive toxicology. | |
Retrosynthesis Analysis
AI-Powered Synthesis Planning: Our tool employs the Template_relevance Pistachio, Template_relevance Bkms_metabolic, Template_relevance Pistachio_ringbreaker, Template_relevance Reaxys, Template_relevance Reaxys_biocatalysis model, leveraging a vast database of chemical reactions to predict feasible synthetic routes.
One-Step Synthesis Focus: Specifically designed for one-step synthesis, it provides concise and direct routes for your target compounds, streamlining the synthesis process.
Accurate Predictions: Utilizing the extensive PISTACHIO, BKMS_METABOLIC, PISTACHIO_RINGBREAKER, REAXYS, REAXYS_BIOCATALYSIS database, our tool offers high-accuracy predictions, reflecting the latest in chemical research and data.
Strategy Settings
Precursor scoring | Relevance Heuristic |
---|---|
Min. plausibility | 0.01 |
Model | Template_relevance |
Template Set | Pistachio/Bkms_metabolic/Pistachio_ringbreaker/Reaxys/Reaxys_biocatalysis |
Top-N result to add to graph | 6 |
Feasible Synthetic Routes
試験管内研究製品の免責事項と情報
BenchChemで提示されるすべての記事および製品情報は、情報提供を目的としています。BenchChemで購入可能な製品は、生体外研究のために特別に設計されています。生体外研究は、ラテン語の "in glass" に由来し、生物体の外で行われる実験を指します。これらの製品は医薬品または薬として分類されておらず、FDAから任何の医療状態、病気、または疾患の予防、治療、または治癒のために承認されていません。これらの製品を人間または動物に体内に導入する形態は、法律により厳格に禁止されています。これらのガイドラインに従うことは、研究と実験において法的および倫理的な基準の遵守を確実にするために重要です。