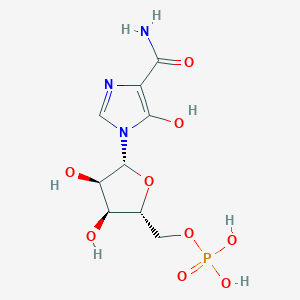
Bredinin 5'-monophosphate
概要
説明
Bredinin 5'-monophosphate (breMP) is the active metabolite of Bredinin (mizoribine), an imidazole nucleoside antibiotic originally isolated from Eupenicillium brefeldianum . Bredinin itself is a prodrug that undergoes intracellular phosphorylation to form breMP, which acts as a potent inhibitor of inosine monophosphate dehydrogenase (IMPDH), a key enzyme in the de novo purine biosynthesis pathway . IMPDH catalyzes the oxidation of inosine 5'-monophosphate (IMP) to xanthosine 5'-monophosphate (XMP), a critical step in guanosine 5'-monophosphate (GMP) synthesis. By mimicking the transition state of IMPDH catalysis, breMP binds with high affinity (Ki = 0.4 nM for E. coli IMPDH; 4.0 nM for human type II IMPDH), leading to competitive inhibition and depletion of intracellular GTP pools . This mechanism underpins breMP’s immunosuppressive and antiviral activities, which are reversed by exogenous guanosine or GMP supplementation .
BreMP also selectively inhibits mammalian DNA polymerases α and β in vitro, with distinct inhibition modes:
準備方法
Photochemical Ring-Opening and Phosphorylation
Synthesis via AICA-Riboside Precursor
The most widely documented route to bredinin 5'-monophosphate derivatives begins with 2',3'-O-isopropylidene-AICA-riboside (4), which undergoes ultraviolet (UV) light-induced ring-opening in 0.1 M aqueous formic acid to yield the intermediate (5) at 34% efficiency . This step exploits the photolability of the imidazole ring, generating a malonamide structure amenable to phosphorylation. Subsequent protection of the amine group with di-tert-butyl pyrocarbonate (Boc) produces compound (6), which is phosphorylated using p-chlorophenylphosphoroditriazolide in dioxane .
Phosphorylation and Deprotection Challenges
Phosphorylation of the 5'-hydroxyl group requires careful selection of protecting groups due to the zwitterionic nature of the bredinin base, which complicates traditional phosphodiester methods . Oleyl or cetyl alcohol is introduced via phosphotriester formation (7a/b), followed by simultaneous deprotection of the isopropylidene and Boc groups using 90% trifluoroacetic acid (TFA). Final ring closure with triethyl orthoformate in dimethylformamide (DMF) at 90°C furnishes the protected monophosphate (9a/b), which is deblocked using N,N,N',N'-tetramethylguanidinium pyridine-2-aldoximate to yield this compound analogs (2a/b) .
Table 1: Key Reaction Parameters and Yields for Photochemical Synthesis
Step | Reagents/Conditions | Yield (%) |
---|---|---|
Ring-opening of (4) | UV (254 nm), 0.1 M HCOOH | 34 |
Boc protection | Di-tert-butyl pyrocarbonate, DMF | 82 |
Phosphorylation | p-ClPhP(O)(NTz)₂, dioxane | 68 |
Deprotection | 90% TFA, rt, 2 h | 91 |
Final deblocking | TMGPox, aqueous dioxane | 76 |
Intramolecular Cyclization of Carbocyclic Analogs
Alternative Pathways for Ring Formation
A carbocyclic analog of this compound was synthesized to circumvent challenges associated with ribose phosphorylation . Starting from a protected carbocyclic diol (5), sequential malonyl ester formation and oximation produced intermediate (10), which was catalytically reduced to amine (11). Cyclization using triethyl orthoformate in ethanol at 90°C with acetic acid catalysis afforded the imidazolium-5-olate ring (12) in 55% yield . Deprotection with methanolic HCl yielded the target compound (3), albeit with moderate antiviral activity.
Limitations in Photolytic Steps
Initial attempts to replicate the photochemical ring-opening used in ribose-based syntheses failed for carbocyclic precursors, leading to intractable mixtures . This underscores the dependency of photolytic efficiency on the sugar moiety’s stereoelectronic properties.
Table 2: Performance of Carbocyclic Synthesis
Intermediate | Key Transformation | Yield (%) |
---|---|---|
5 → 6 | MOM deprotection, acetylation | 65.1 |
11 → 12 | Cyclization with orthoformate | 55 |
12 → 3 | Acidic deprotection | 54 |
Enzymatic Salvage Synthesis
Fermentation and Purification
An enzymatic salvage pathway utilizing Eapenisillium brefeldianum has been reported for bredinin production . The extracellular bredinin is adsorbed onto Amberlite IRA-411 (OH⁻ form) and eluted with 2.0% acetic acid. While yields are unspecified, this method avoids harsh chemical conditions, making it suitable for lab-scale isolation.
Comparative Analysis of Methods
Efficiency and Scalability
The photochemical method achieves moderate yields (34–76%) but requires specialized UV equipment and hazardous reagents like TFA. The carbocyclic route offers a safer alternative with comparable yields (54–65%) but lower bioactivity. Enzymatic synthesis is environmentally benign but lacks yield data and scalability metrics.
Structural Insights from NMR
Nuclear magnetic resonance (NMR) studies of related 5'-monophosphates, such as inosine 5'-monophosphate, reveal critical chemical shifts (e.g., C1 at 66.154 ppm, H31 at 6.115 ppm) . These data assist in verifying this compound purity and regiochemistry.
化学反応の分析
ミゾリビンリン酸は、次のような様々な化学反応を起こします。
酸化: ミゾリビンリン酸は、特定の条件下で酸化されて酸化誘導体となる可能性があります。
還元: 還元反応は、ミゾリビンリン酸をその還元形に変換することができます。
置換: ミゾリビンリン酸は、特定の官能基が他の基に置き換えられる置換反応を起こす可能性があります。これらの反応で使用される一般的な試薬と条件には、酸化剤、還元剤、特定の触媒が含まれます. これらの反応から生成される主な生成物は、使用される特定の試薬と条件によって異なります。
科学的研究の応用
Scientific Research Applications
Bredinin 5'-monophosphate has a diverse range of applications across multiple scientific disciplines:
-
Chemistry :
- Utilized as a reagent in chemical reactions to study the inhibition of specific enzymes.
- Serves as a model compound for exploring nucleoside interactions and modifications.
-
Biology :
- Employed in studies involving DNA polymerases, particularly mammalian DNA polymerases alpha and beta.
- Investigated for its effects on lymphocyte differentiation and proliferation due to its immunosuppressive properties.
-
Medicine :
- Potential therapeutic applications include treatment for autoimmune diseases and organ transplantation.
- Its broad-spectrum antiviral activity positions it as a candidate for further investigation in viral infections.
-
Industry :
- Used in the pharmaceutical industry for the development of new immunosuppressive drugs.
- May play a role in the synthesis of other biologically active compounds.
Case Studies and Research Findings
Numerous studies have documented the effects and potential applications of this compound:
- A study demonstrated that breMP inhibited mammalian DNA polymerases alpha and beta effectively at low concentrations (less than 7 µg/ml), showcasing its potency as an inhibitor .
- Research highlighted its role in suppressing lymphocyte proliferation, which is crucial for understanding its immunosuppressive effects .
- Additional investigations into its antiviral properties revealed that breMP could be effective against various viral infections, warranting further clinical studies.
作用機序
類似化合物との比較
Comparative Analysis with Structurally or Functionally Related Compounds
Guanosine 5'-Monophosphate (GMP)
- Structural Similarity: Both breMP and GMP are ribonucleotides with a 5'-monophosphate group. However, breMP contains a carbamoylated imidazole ring, whereas GMP features a guanine base .
- Functional Contrast: GMP is a downstream product of IMPDH activity and reverses breMP-induced inhibition of viral replication and cell proliferation .
Inosine 5'-Monophosphate (IMP)
- Role in Metabolism : IMP is the substrate of IMPDH and a precursor for AMP and GMP synthesis. BreMP’s structural similarity to IMP allows it to occupy the IMPDH active site, whereas IMP itself is metabolized into downstream purines .
Adenosine 5'-Monophosphate (AMP)
- Energy Metabolism : AMP is a component of the adenine nucleotide pool, critical for ATP synthesis and cellular energy homeostasis .
- Inhibitory Profile: AMP weakly binds IMPDH (millimolar affinity) but primarily interacts with the adenosine portion of the dinucleotide site, unlike breMP’s high-affinity, transition-state mimicry .
Other Nucleotide Analogs
- Ribavirin Monophosphate: Shares breMP’s antiviral activity but targets viral RNA polymerases via lethal mutagenesis rather than IMPDH inhibition .
- Oxanosine Monophosphate: A natural IMPDH inhibitor (Ki = 1 μM) with antineoplastic activity, but less potent than breMP (Ki = 0.4–4.0 nM) .
- SM-108 Nucleotide : A synthetic IMP dehydrogenase inhibitor activated by adenine phosphoribosyltransferase. Like breMP, it requires phosphorylation for activity but exhibits a distinct structure-activity relationship .
Data Tables: Key Biochemical and Pharmacological Comparisons
Table 1. Inhibition Constants (Ki) of IMPDH Inhibitors
Table 2. Selectivity Profiles of Nucleotide Analogs
Compound | Target Enzymes/Pathways | Reversal Agents | Therapeutic Use |
---|---|---|---|
This compound | IMPDH, DNA polymerases α/β | Guanosine, GMP | Immunosuppression, Antiviral |
Ribavirin Monophosphate | Viral RNA-dependent RNA polymerases | None | Hepatitis C, RSV |
SM-108 Nucleotide | IMP dehydrogenase (after activation) | Adenine | Antitumor |
Mechanistic and Clinical Implications
BreMP’s unique ability to inhibit both IMPDH and DNA polymerases positions it as a multitarget agent. In contrast, breMP’s antiviral activity against RNA viruses (e.g., BVDV, HCV) remains significant, particularly when combined with IFN-α (EC50 = 0.66 μM vs. 5.3 μM alone) .
生物活性
Bredinin 5'-monophosphate (breMP) is an active metabolite of the immunosuppressive drug bredinin, which has garnered attention for its selective inhibitory effects on mammalian DNA polymerases and its potential antiviral properties. This article explores the biological activity of breMP, focusing on its mechanisms of action, effects on DNA polymerases, and antiviral activities.
Bredinin itself does not exhibit significant inhibitory effects on DNA polymerases; however, upon conversion to its 5'-monophosphate form (breMP), it demonstrates potent inhibition of mammalian DNA polymerases alpha (pol. α) and beta (pol. β). The following table summarizes the inhibitory effects observed:
DNA Polymerase | Inhibition Concentration | Mechanism of Action |
---|---|---|
Pol. α | < 7 µg/ml | Competes with substrate and non-competitively with template-primer |
Pol. β | 7 µg/ml | Competes with both substrate and template-primer |
In vitro studies indicate that breMP selectively inhibits these polymerases without affecting plant or prokaryotic DNA polymerases, highlighting its specificity for mammalian systems . The inhibition mechanism varies between the two polymerases; for pol. β, breMP competes with both the substrate and the template-primer, while for pol. α, it competes only with the substrate .
Antiviral Activity
Bredinin and its analogs have shown antiviral properties against various RNA viruses. The active metabolite breMP is believed to mimic the transition state in the catalysis of inosine monophosphate dehydrogenase (IMPDH), a key enzyme in nucleotide synthesis, which is critical for viral replication . The following table outlines the antiviral activity of bredinin against different viruses:
Virus Type | EC50 Value (µM) | Comments |
---|---|---|
Bovine Viral Diarrhea Virus | 0.66 (in combination with IFN-α) | Synergistic effect observed |
Respiratory Syncytial Virus | Submicromolar range | Effective against multiple strains |
Hepatitis C Virus | Not specified | Further studies ongoing |
Bredinin's antiviral efficacy is particularly notable in combination therapies, where it enhances the effects of interferon-alpha against certain viral infections .
Case Studies and Research Findings
Several studies have documented the biological activities of bredinin and breMP:
- Inhibition of DNA Polymerases : A study demonstrated that breMP effectively inhibits mammalian DNA polymerase activities at concentrations significantly lower than those required for bredinin itself. This suggests that breMP's conversion in vivo is crucial for its immunosuppressive effects .
- Antiviral Studies : Research has shown that bredinin exhibits low antiviral activity against several RNA viruses, but its potential can be enhanced through structural modifications to increase efficacy . Ongoing investigations aim to explore new analogs that may improve this activity.
- Clinical Implications : Bredinin has been used clinically in Japan as an immunosuppressive agent, particularly in organ transplantation settings. Understanding its mechanism through breMP provides insights into optimizing its use in clinical practice .
Q & A
Q. Basic: What is the molecular mechanism by which Bredinin 5'-monophosphate inhibits mammalian DNA polymerases?
Answer:
this compound (breMP) selectively inhibits DNA polymerase α (pol α) and β (pol β) through distinct competitive mechanisms. For pol β, breMP competes with both the substrate (dNTPs) and template-primer, while for pol α, it acts as a non-competitive inhibitor against the template-primer but competes with the substrate . This specificity is critical for its immunosuppressive effects, as breMP does not inhibit prokaryotic (e.g., E. coli pol I) or plant DNA polymerases.
Methodology:
- Use in vitro enzyme activity assays with purified pol α/β and varying substrate (dNTP) concentrations.
- Measure inhibition kinetics via Lineweaver-Burk plots to distinguish competitive vs. non-competitive inhibition.
- Validate selectivity using parallel assays with non-mammalian polymerases (e.g., Taq polymerase) .
Q. Advanced: How do experimental conditions (e.g., temperature, pH) influence breMP's inhibitory efficacy in enzymatic assays?
Answer:
Enzyme kinetics are highly sensitive to assay conditions. For example, thermophilic archaeal IMP cyclohydrolases (e.g., AF1811 from Archaeoglobus fulgidus) show underestimated kcat values at suboptimal temperatures (e.g., 50°C vs. their native 76–85°C) . Similarly, breMP’s inhibition constants (Ki) may vary with pH or ionic strength, altering its binding affinity to pol α/β.
Methodology:
- Perform activity assays at multiple temperatures (e.g., 25–70°C) and pH ranges (5.0–9.0).
- Calculate IC50 shifts under varied conditions using Michaelis-Menten kinetics.
- Normalize data against control reactions (e.g., enzyme stability assays) to isolate breMP-specific effects .
Q. Basic: What experimental models are appropriate for studying breMP's immunosuppressive activity?
Answer:
- Bacterial complementation assays : Use E. coli ΔpurH strains transformed with plasmids encoding purine salvage pathway enzymes (e.g., AICAR transformylase) to test breMP’s functional rescue .
- Mammalian cell cultures : Measure lymphocyte proliferation inhibition via thymidine incorporation assays.
Methodology: - For bacterial models: Plate transformed E. coli on purine-deficient media and monitor growth rescue with breMP supplementation .
- For mammalian systems: Treat activated T-cells with breMP and quantify DNA synthesis using radiolabeled thymidine .
Q. Advanced: How can researchers address discrepancies in reported kinetic parameters (e.g., Km, kcat) for breMP across studies?
Answer:
Discrepancies often arise from assay standardization issues. For instance, IMP cyclohydrolase studies showed TK0430’s Km (1.56 µM) is 50x lower than Methanocaldococcus jannaschii PurO due to temperature differences (50°C vs. native 85°C) .
Methodology:
- Replicate studies under identical conditions (temperature, buffer composition, enzyme purity).
- Include internal controls (e.g., known inhibitors like aphidicolin for pol α) to calibrate assays.
- Use high-purity breMP (HPLC-validated) to minimize batch variability .
Q. Basic: What structural features of breMP confer specificity for mammalian DNA polymerases?
Answer:
breMP’s 5'-monophosphate group is critical for binding to pol α/β active sites, as unphosphorylated Bredinin shows no inhibition. Comparative docking studies suggest its ribose moiety interacts with conserved residues in mammalian polymerases, absent in prokaryotic homologs .
Methodology:
- Conduct crystallography or cryo-EM to resolve breMP-polymerase complexes.
- Synthesize breMP analogs (e.g., 3'-monophosphate) and test inhibitory activity via in vitro assays.
Q. Advanced: What strategies can resolve contradictory data on breMP’s off-target effects in non-immune cells?
Answer:
Contradictions may stem from cell-type-specific metabolic differences (e.g., nucleotide salvage pathway activity).
Methodology:
- Perform RNA-seq or metabolomics to identify breMP-sensitive pathways in divergent cell lines.
- Use CRISPR knockouts (e.g., POLA1/POLB) to isolate polymerase-specific effects.
- Validate findings with isoform-specific inhibitors (e.g., α-amanitin for pol II) .
Q. Basic: How is breMP’s enzymatic inhibition quantified in vitro?
Answer:
- Kinetic assays : Measure initial reaction rates (V0) at varying breMP concentrations.
- Data fitting : Use nonlinear regression (e.g., GraphPad Prism) to calculate Ki values from Michaelis-Menten curves .
Methodology: - Optimize substrate concentrations to avoid substrate depletion.
- Include negative controls (e.g., heat-inactivated enzymes) to account for non-enzymatic background .
Q. Advanced: Can breMP’s selectivity be exploited to study polymerase-specific DNA repair mechanisms?
Answer:
Yes. breMP’s pol β inhibition makes it a tool to dissect base excision repair (BER), where pol β is dominant.
Methodology:
特性
IUPAC Name |
[(2R,3S,4R,5R)-5-(4-carbamoyl-5-hydroxyimidazol-1-yl)-3,4-dihydroxyoxolan-2-yl]methyl dihydrogen phosphate | |
---|---|---|
Source | PubChem | |
URL | https://pubchem.ncbi.nlm.nih.gov | |
Description | Data deposited in or computed by PubChem | |
InChI |
InChI=1S/C9H14N3O9P/c10-7(15)4-8(16)12(2-11-4)9-6(14)5(13)3(21-9)1-20-22(17,18)19/h2-3,5-6,9,13-14,16H,1H2,(H2,10,15)(H2,17,18,19)/t3-,5-,6-,9-/m1/s1 | |
Source | PubChem | |
URL | https://pubchem.ncbi.nlm.nih.gov | |
Description | Data deposited in or computed by PubChem | |
InChI Key |
KTKAFSMJDTUUAN-UUOKFMHZSA-N | |
Source | PubChem | |
URL | https://pubchem.ncbi.nlm.nih.gov | |
Description | Data deposited in or computed by PubChem | |
Canonical SMILES |
C1=NC(=C(N1C2C(C(C(O2)COP(=O)(O)O)O)O)O)C(=O)N | |
Source | PubChem | |
URL | https://pubchem.ncbi.nlm.nih.gov | |
Description | Data deposited in or computed by PubChem | |
Isomeric SMILES |
C1=NC(=C(N1[C@H]2[C@@H]([C@@H]([C@H](O2)COP(=O)(O)O)O)O)O)C(=O)N | |
Source | PubChem | |
URL | https://pubchem.ncbi.nlm.nih.gov | |
Description | Data deposited in or computed by PubChem | |
Molecular Formula |
C9H14N3O9P | |
Source | PubChem | |
URL | https://pubchem.ncbi.nlm.nih.gov | |
Description | Data deposited in or computed by PubChem | |
DSSTOX Substance ID |
DTXSID40977565 | |
Record name | 5-Hydroxy-1-(5-O-phosphonopentofuranosyl)-1H-imidazole-4-carboxamide | |
Source | EPA DSSTox | |
URL | https://comptox.epa.gov/dashboard/DTXSID40977565 | |
Description | DSSTox provides a high quality public chemistry resource for supporting improved predictive toxicology. | |
Molecular Weight |
339.20 g/mol | |
Source | PubChem | |
URL | https://pubchem.ncbi.nlm.nih.gov | |
Description | Data deposited in or computed by PubChem | |
CAS No. |
62025-48-3 | |
Record name | Bredinin 5'-monophosphate | |
Source | ChemIDplus | |
URL | https://pubchem.ncbi.nlm.nih.gov/substance/?source=chemidplus&sourceid=0062025483 | |
Description | ChemIDplus is a free, web search system that provides access to the structure and nomenclature authority files used for the identification of chemical substances cited in National Library of Medicine (NLM) databases, including the TOXNET system. | |
Record name | 5-Hydroxy-1-(5-O-phosphonopentofuranosyl)-1H-imidazole-4-carboxamide | |
Source | EPA DSSTox | |
URL | https://comptox.epa.gov/dashboard/DTXSID40977565 | |
Description | DSSTox provides a high quality public chemistry resource for supporting improved predictive toxicology. | |
Retrosynthesis Analysis
AI-Powered Synthesis Planning: Our tool employs the Template_relevance Pistachio, Template_relevance Bkms_metabolic, Template_relevance Pistachio_ringbreaker, Template_relevance Reaxys, Template_relevance Reaxys_biocatalysis model, leveraging a vast database of chemical reactions to predict feasible synthetic routes.
One-Step Synthesis Focus: Specifically designed for one-step synthesis, it provides concise and direct routes for your target compounds, streamlining the synthesis process.
Accurate Predictions: Utilizing the extensive PISTACHIO, BKMS_METABOLIC, PISTACHIO_RINGBREAKER, REAXYS, REAXYS_BIOCATALYSIS database, our tool offers high-accuracy predictions, reflecting the latest in chemical research and data.
Strategy Settings
Precursor scoring | Relevance Heuristic |
---|---|
Min. plausibility | 0.01 |
Model | Template_relevance |
Template Set | Pistachio/Bkms_metabolic/Pistachio_ringbreaker/Reaxys/Reaxys_biocatalysis |
Top-N result to add to graph | 6 |
Feasible Synthetic Routes
試験管内研究製品の免責事項と情報
BenchChemで提示されるすべての記事および製品情報は、情報提供を目的としています。BenchChemで購入可能な製品は、生体外研究のために特別に設計されています。生体外研究は、ラテン語の "in glass" に由来し、生物体の外で行われる実験を指します。これらの製品は医薬品または薬として分類されておらず、FDAから任何の医療状態、病気、または疾患の予防、治療、または治癒のために承認されていません。これらの製品を人間または動物に体内に導入する形態は、法律により厳格に禁止されています。これらのガイドラインに従うことは、研究と実験において法的および倫理的な基準の遵守を確実にするために重要です。