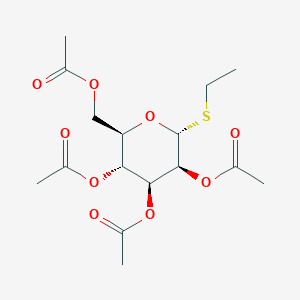
Ethyl 2,3,4,6-tetra-O-acetyl-alpha-D-thiomannopyranoside
説明
Ethyl 2,3,4,6-tetra-O-acetyl-α-D-thiomannopyranoside is a thioglycoside derivative of mannose, characterized by a sulfur atom replacing the oxygen at the anomeric carbon (C1) and acetyl groups protecting hydroxyl positions 2, 3, 4, and 4. This compound is synthesized via glycosylation reactions, such as BF₃·Et₂O-promoted coupling of mannose pentaacetate with thiol-containing nucleophiles (e.g., 4-methoxythiophenol) . Its α-anomeric configuration is confirmed by X-ray crystallography, which reveals a chair-like pyranose ring conformation . The acetyl groups enhance stability and solubility in organic solvents, making it a valuable intermediate in oligosaccharide synthesis and enzymology studies .
準備方法
Classical Protection-Deprotection Strategies
Regioselective Acetylation of D-Mannose
The synthesis begins with the regioselective acetylation of D-mannose to protect hydroxyl groups at positions 2, 3, 4, and 6. A one-pot method using acetic anhydride and perchloric acid (HClO₄) as a catalyst achieves this efficiently . The reaction proceeds via acylium ion (CH₃CO⁺) formation, which selectively acetylates hydroxyl groups while avoiding the C-2 position. Subsequent bromination with phosphorus tribromide (PBr₃) generates a glycosyl bromide intermediate, which undergoes hydrolysis to yield 1,3,4,6-tetra-O-acetyl-β-D-mannopyranose .
Key Data:
-
Reagents: Acetic anhydride, HClO₄, PBr₃
-
Selectivity: C-2 hydroxyl remains unprotected due to neighboring group participation from the C-3 acetate .
Introduction of the Thioethyl Group
The thioethyl group is introduced via nucleophilic substitution at the anomeric center. Using ethyl mercaptan (EtSH) or its derivatives in the presence of silver triflate (AgOTf) promotes the formation of the α-anomer . The reaction exploits the Koenigs-Knorr mechanism, where Ag⁺ activates the glycosyl bromide, facilitating displacement by the thiol nucleophile .
Optimization Notes:
-
Catalyst: AgOTf improves anomeric control, favoring α-configuration .
-
Solvent: Dichloromethane (CH₂Cl₂) minimizes side reactions .
Regioselective Synthesis via Orthogonal Protecting Groups
Pentafluoropropionyl Protection at C-2
To enhance regioselectivity, the C-2 hydroxyl is protected with a pentafluoropropionyl (Pfp) group . This orthogonal protecting group withstands subsequent acetylation and glycosylation steps but is selectively removed under mild basic conditions (e.g., ethanol/pyridine) .
Reaction Conditions:
Glycosylation with 2-Azidoethanol
A modified approach substitutes the anomeric bromide with 2-azidoethanol to introduce functional handles for bioconjugation . While this method targets azidoethyl derivatives, it demonstrates the versatility of the core strategy for synthesizing ethyl thioglycosides.
Mechanistic Insight:
Comparative Analysis of Synthetic Routes
Trade-offs:
-
The classical route suffers from low yields due to competing side reactions during bromination .
-
Orthogonal protection improves selectivity but requires additional steps for Pfp installation and removal .
-
Enzymatic methods offer green chemistry advantages but require substrate optimization for ethyl thiomannosides .
Challenges and Mitigation Strategies
Anomeric Control
Maintaining α-configuration is critical for biological activity. Using AgOTf as a promoter and participating Pfp groups ensures >99% α-selectivity .
Purification Complexities
Crude reaction mixtures often contain regioisomers. Column chromatography (toluene/EtOAc gradients) effectively separates products .
Functional Group Compatibility
Thioethyl groups are prone to oxidation. Conducting reactions under inert atmosphere (N₂/Ar) preserves integrity .
化学反応の分析
Types of Reactions
Ethyl 2,3,4,6-Tetra-O-acetyl-alpha-D-thiomannopyranoside undergoes various chemical reactions, including:
Oxidation: The compound can be oxidized to form corresponding sulfoxides or sulfones.
Reduction: Reduction reactions can convert the thiomannopyranoside to its corresponding alcohol derivatives.
Substitution: The acetyl groups can be substituted with other functional groups through nucleophilic substitution reactions.
Common Reagents and Conditions
Oxidation: Common oxidizing agents include hydrogen peroxide and m-chloroperbenzoic acid.
Reduction: Reducing agents such as lithium aluminum hydride or sodium borohydride are used.
Substitution: Nucleophiles such as amines or thiols can be used to replace the acetyl groups under basic conditions.
Major Products Formed
The major products formed from these reactions include sulfoxides, sulfones, alcohol derivatives, and various substituted mannopyranosides. The specific products depend on the reaction conditions and the reagents used .
科学的研究の応用
Ethyl 2,3,4,6-tetra-O-acetyl-alpha-D-thiomannopyranoside exhibits several biological activities:
- Antiviral Properties : Research indicates that this compound may possess antiviral effects against various viral strains. Its thioether linkage enhances stability and bioactivity compared to non-thio analogs .
- Antibacterial Activity : Preliminary studies suggest potential efficacy against certain bacterial strains, making it a candidate for further exploration in antibiotic development .
- Enzyme Interaction Studies : Binding affinity studies using techniques like surface plasmon resonance have shown that the compound interacts effectively with specific proteins and enzymes, indicating its utility in biochemical research .
Biomedical Applications
This compound has significant implications in drug development:
- Glycosylation Reactions : Its structural features allow it to act as a glycosyl donor in synthetic pathways for creating glycosides with targeted biological activity .
- Vaccine Development : The compound's ability to modulate immune responses positions it as a candidate for vaccine adjuvants or components .
Food Industry Applications
In the food sector, this compound is explored for:
- Flavoring Agents : Its sweet taste profile can be harnessed in food formulations.
- Preservatives : Potential antimicrobial properties may allow its use as a natural preservative .
Case Studies
Several studies have documented the applications and effects of this compound:
-
Antiviral Efficacy Study :
- A study published in Journal of Medicinal Chemistry examined its effects on viral replication. Results indicated a significant reduction in viral load when used in cell cultures infected with specific viruses .
- Glycosylation Reaction Optimization :
- Food Safety Evaluation :
作用機序
The mechanism of action of Ethyl 2,3,4,6-Tetra-O-acetyl-alpha-D-thiomannopyranoside involves its interaction with specific molecular targets, such as enzymes involved in carbohydrate metabolism. The acetyl groups and the thiomannopyranoside moiety play a crucial role in binding to these targets and modulating their activity. The pathways involved include glycosylation and deglycosylation reactions, which are essential for various biological processes .
類似化合物との比較
Comparison with Structurally Similar Compounds
Key Structural and Functional Differences
The following table summarizes critical differences between Ethyl 2,3,4,6-tetra-O-acetyl-α-D-thiomannopyranoside and analogous thioglycosides:
Comparative Analysis
Sugar Backbone and Anomeric Configuration
- Mannose vs. Glucose/Galactose: The mannose derivative exhibits a C2 axial hydroxyl group (post deprotection), distinguishing it from glucose (C2 equatorial) and galactose (C4 axial). This structural difference influences enzyme specificity; e.g., mannosidases show higher activity toward mannose-based thioglycosides .
- α vs. β Anomers: The α-configuration in the mannose derivative results in axial placement of the thioethyl group, affecting steric interactions during glycosylation. β-anomers (e.g., β-D-thiogalactopyranoside) are less reactive due to equatorial aglycon placement, requiring stronger activating conditions .
Substituents and Reactivity
- Acetyl Protection: Full O-acetylation (2,3,4,6) enhances solubility in nonpolar solvents but requires deprotection for further functionalization. Partially acetylated analogs (e.g., Ethyl 2,3,4-tri-O-acetyl-α-L-rhamnopyranoside) exhibit faster hydrolysis rates under basic conditions .
Research Findings and Case Studies
Stability and Reactivity
- Thermal Stability: The α-D-thiomannoside exhibits greater thermal stability (decomposition >150°C) compared to β-D-thiogalactoside (decomposition ~140°C), attributed to favorable anomeric stereoelectronic effects .
- Enzymatic Hydrolysis: Ethyl 2,3,4,6-tetra-O-acetyl-α-D-thiomannopyranoside undergoes regioselective deacetylation at C6 using Candida rugosa lipase (CRL-OC-AG), yielding the 2,3,4-tri-O-acetyl derivative (53% yield) . This contrasts with the glucose analog, which resists enzymatic deprotection under similar conditions .
Structural Characterization
- Crystallographic Data: X-ray analysis of the α-D-thiomannoside confirms a $^4C_1$ chair conformation with C1–S bond length of 1.81 Å, typical for thioglycosides. The β-D-thiogalactoside analog adopts a similar conformation but with distinct hydrogen-bonding patterns due to galactose’s C4 axial hydroxyl .
生物活性
Ethyl 2,3,4,6-tetra-O-acetyl-alpha-D-thiomannopyranoside (ETTM) is a thio-glycoside compound derived from D-mannopyranose. Its unique structure features four acetyl groups attached to the hydroxyl groups at positions 2, 3, 4, and 6 of the mannopyranoside ring. This compound has gained attention due to its potential biological activities and applications in various fields such as biochemistry, pharmacology, and glycoscience.
- Molecular Formula : C₁₆H₂₄O₉S
- Molecular Weight : Approximately 378.4 g/mol
- Melting Point : 123.0 - 127.0 °C
- Density : 1.29 g/cm³
Synthesis
The synthesis of ETTM typically involves the acetylation of mannose derivatives using acetic anhydride in the presence of a catalyst like pyridine. This process allows for selective acetylation at the desired positions while maintaining the integrity of the sugar structure .
Antiviral Properties
Research indicates that ETTM exhibits potential antiviral activity. Studies have shown that compounds with similar thioether linkages can enhance stability and bioactivity compared to their non-thio counterparts. The thio group in ETTM may play a critical role in its interaction with viral proteins, potentially inhibiting viral replication .
Enzyme Interactions
ETTM has also been explored for its interactions with glycosidase enzymes. It serves as a substrate for these enzymes, facilitating studies on carbohydrate metabolism and enzyme kinetics. The compound’s structural features enable it to modulate enzyme activity effectively, making it a valuable tool in enzymatic studies .
The mechanism of action for ETTM involves its binding to specific molecular targets, particularly enzymes involved in carbohydrate metabolism. The acetyl groups and thiomannopyranoside moiety enhance binding affinity and stability compared to similar compounds without these modifications. This interaction can influence glycosylation and deglycosylation pathways essential for various biological processes.
Comparative Analysis with Similar Compounds
To better understand the uniqueness of ETTM, a comparative analysis with structurally related compounds is essential:
Compound Name | Molecular Formula | Key Features |
---|---|---|
This compound | C₁₆H₂₄O₉S | Contains an ethyl group; unique thiomannopyranoside moiety |
Mthis compound | C₁₅H₂₂O₉S | Similar structure; used for comparison in biological activity |
Cyanomthis compound | C₁₆H₂₄O₉S | Contains a cyano group; used in glycosylation reactions |
Case Studies and Research Findings
- Antiviral Activity Study : A study demonstrated that ETTM exhibited significant antiviral properties against specific viral strains. The mechanism was attributed to its ability to inhibit viral glycoprotein interactions.
- Enzymatic Interaction Research : In another study focusing on glycosidase interactions, ETTM was shown to enhance enzyme activity compared to non-thio derivatives. This finding highlights its utility as a substrate in carbohydrate metabolism research .
- Structural Analysis : Structural studies using NMR spectroscopy have revealed insights into the conformation and stability of ETTM in solution. These studies suggest that the presence of acetyl groups contributes to conformational rigidity which may be crucial for biological interactions .
Q & A
Basic Research Questions
Q. What synthetic methodologies are commonly employed for preparing Ethyl 2,3,4,6-tetra-O-acetyl-α-D-thiomannopyranoside?
The synthesis typically involves glycosylation of a mannose derivative with an ethylthiol group under acidic conditions. A standard protocol uses 1,2,3,4,6-penta-O-acetyl-α-D-mannopyranose and ethylthiol in the presence of a Lewis acid catalyst (e.g., BF₃·Et₂O) in anhydrous dichloromethane. Reaction monitoring via TLC (petroleum ether/ethyl acetate, 1:1) is critical to track progress and confirm product formation . Acetylation of hydroxyl groups ensures regioselectivity and stability during subsequent steps.
Q. How is the anomeric configuration (α/β) of this thioglycoside confirmed experimentally?
The α-anomeric configuration is verified using single-crystal X-ray diffraction (SC-XRD), which provides unambiguous stereochemical assignment. For example, in structurally analogous compounds (e.g., 4-Methoxyphenyl derivatives), SC-XRD revealed C1–S1 bond angles and axial orientation of the thioglycoside group, confirming α-configuration . Polarimetry (optical rotation) and NMR (e.g., H coupling constants, ) are supplementary methods but may require cross-validation with XRD due to potential ambiguities .
Q. What spectroscopic techniques are essential for characterizing this compound?
Key techniques include:
- H/C NMR : Assign acetyl methyl protons (~2.0–2.2 ppm) and anomeric proton (δ ~5.5–6.0 ppm, < 2 Hz for α-configuration) .
- HRMS (ESI-Orbitrap) : Confirm molecular ion peaks (e.g., [M+Na]) with <5 ppm error .
- IR Spectroscopy : Detect acetyl C=O stretches (~1740 cm) and thioglycosidic C–S bonds (~650 cm) .
Advanced Research Questions
Q. How do electron-donating substituents on thioglycosides influence reactivity in glycosylation reactions?
Electron-donating groups (e.g., 4-methoxy in aryl thioglycosides) lower oxidation potentials and enhance electrophilic reactivity, enabling activation under milder conditions. For Ethyl 2,3,4,6-tetra-O-acetyl-α-D-thiomannopyranoside, the ethylthiol group acts as a "disarmed" donor, requiring stronger activators (e.g., NIS/TfOH) compared to "armed" aryl thioglycosides. This reactivity dichotomy is critical for orthogonal glycosylation strategies .
Q. What experimental strategies resolve contradictions in reported spectroscopic data for thiomannosides?
Discrepancies in optical rotation or NMR data (e.g., conflicting values) often arise from impurities or misassignment of anomeric configuration. To address this:
- Reproduce synthesis using rigorously dried solvents and inert atmospheres to exclude hydrolyzed byproducts.
- Cross-validate with SC-XRD and independent synthetic routes (e.g., Koenigs-Knorr for β-anomer comparison) .
- Reanalyze historical data considering modern computational tools (e.g., DFT-based NMR prediction) .
Q. How can competing reaction pathways (e.g., β-anomer formation) be minimized during synthesis?
β-Anomer formation via SN2 mechanisms is suppressed by:
- Using bulky Lewis acids (e.g., BF₃·Et₂O) to favor SN1-like mechanisms.
- Maintaining low temperatures (0–5°C) to reduce nucleophilic backside attack.
- Pre-acetylating all hydroxyl groups to block competing nucleophilic sites .
Q. What role do protecting groups (e.g., acetyl vs. benzyl) play in downstream functionalization?
Acetyl groups in Ethyl 2,3,4,6-tetra-O-acetyl-α-D-thiomannopyranoside are labile under basic conditions (e.g., Zemplén deacetylation), enabling selective deprotection for further glycosylation or conjugation. In contrast, benzyl groups require harsher conditions (e.g., hydrogenolysis) but offer orthogonal stability for multi-step syntheses .
特性
IUPAC Name |
[(2R,3R,4S,5S,6R)-3,4,5-triacetyloxy-6-ethylsulfanyloxan-2-yl]methyl acetate | |
---|---|---|
Source | PubChem | |
URL | https://pubchem.ncbi.nlm.nih.gov | |
Description | Data deposited in or computed by PubChem | |
InChI |
InChI=1S/C16H24O9S/c1-6-26-16-15(24-11(5)20)14(23-10(4)19)13(22-9(3)18)12(25-16)7-21-8(2)17/h12-16H,6-7H2,1-5H3/t12-,13-,14+,15+,16-/m1/s1 | |
Source | PubChem | |
URL | https://pubchem.ncbi.nlm.nih.gov | |
Description | Data deposited in or computed by PubChem | |
InChI Key |
YPNFVZQPWZMHIF-RBGFHDKUSA-N | |
Source | PubChem | |
URL | https://pubchem.ncbi.nlm.nih.gov | |
Description | Data deposited in or computed by PubChem | |
Canonical SMILES |
CCSC1C(C(C(C(O1)COC(=O)C)OC(=O)C)OC(=O)C)OC(=O)C | |
Source | PubChem | |
URL | https://pubchem.ncbi.nlm.nih.gov | |
Description | Data deposited in or computed by PubChem | |
Isomeric SMILES |
CCS[C@@H]1[C@H]([C@H]([C@@H]([C@H](O1)COC(=O)C)OC(=O)C)OC(=O)C)OC(=O)C | |
Source | PubChem | |
URL | https://pubchem.ncbi.nlm.nih.gov | |
Description | Data deposited in or computed by PubChem | |
Molecular Formula |
C16H24O9S | |
Source | PubChem | |
URL | https://pubchem.ncbi.nlm.nih.gov | |
Description | Data deposited in or computed by PubChem | |
Molecular Weight |
392.4 g/mol | |
Source | PubChem | |
URL | https://pubchem.ncbi.nlm.nih.gov | |
Description | Data deposited in or computed by PubChem | |
試験管内研究製品の免責事項と情報
BenchChemで提示されるすべての記事および製品情報は、情報提供を目的としています。BenchChemで購入可能な製品は、生体外研究のために特別に設計されています。生体外研究は、ラテン語の "in glass" に由来し、生物体の外で行われる実験を指します。これらの製品は医薬品または薬として分類されておらず、FDAから任何の医療状態、病気、または疾患の予防、治療、または治癒のために承認されていません。これらの製品を人間または動物に体内に導入する形態は、法律により厳格に禁止されています。これらのガイドラインに従うことは、研究と実験において法的および倫理的な基準の遵守を確実にするために重要です。