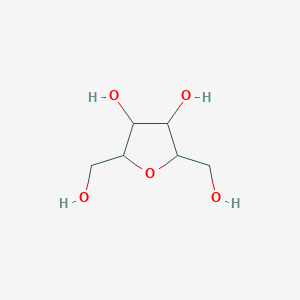
2,5-Anhydro-D-mannitol
概要
説明
2,5-Anhydro-D-mannitol (2,5-AM) is a fructose analog characterized by a unique anhydro bridge between C2 and C5, rendering it a metabolically trapped compound. It is synthesized from D-glucosamine via a two-step process with a 72% yield . Structurally, it adopts distinct conformations in solution (symmetric 4T3) and solid states (asymmetric), as observed in its derivatives like 3,4-Di-O-acetyl-2,5-anhydro-1,6-dideoxy-1,6-diiodo-D-mannitol . Functionally, 2,5-AM inhibits hepatic gluconeogenesis and glycogenolysis by forming phosphorylated intermediates (1-phosphate and 1,6-bisphosphate) that disrupt key enzymes such as glycogen phosphorylase (Ki = 0.66 mM) and phosphoglucomutase (Ki = 2.8 mM) . It also modulates feeding behavior in rats by reducing hepatic ATP levels via phosphate trapping and extends yeast chronological lifespan (CLS) by accumulating nonmetabolized 1,6-bisphosphate .
準備方法
2,5-Anhydro-D-mannitol can be synthesized through the dehydration of D-mannitol. One common method involves heating a solution of D-mannitol in the presence of a strong acid catalyst . Another practical process involves the reductive cleavage of glycosidic linkages in perallylated carbohydrates using triethylsilane, followed by deallylation with a palladium chloride-copper chloride-activated charcoal system .
化学反応の分析
2,5-Anhydro-D-mannitol undergoes various chemical reactions, including:
Oxidation: It can be oxidized to form corresponding aldehydes or ketones.
Reduction: It can be reduced to form different sugar alcohols.
Substitution: It can participate in substitution reactions to form various carbohydrate derivatives and analogs.
Common reagents and conditions used in these reactions include strong acids for dehydration, triethylsilane for reductive cleavage, and palladium chloride-copper chloride for deallylation . Major products formed from these reactions include various anhydroalditols and carbohydrate derivatives .
科学的研究の応用
Metabolic Research
2,5-AM plays a crucial role in studying carbohydrate metabolism, particularly in hepatic function. It has been shown to inhibit gluconeogenesis and glycogenolysis in liver cells, which are vital processes for maintaining blood glucose levels. This inhibition can lead to altered glucose homeostasis, making it a valuable tool for researchers investigating metabolic disorders such as diabetes.
Key Findings:
- Inhibition of Hepatic Processes : Research indicates that 2,5-AM decreases intracellular ATP levels while increasing sodium levels within hepatocytes compared to fructose, suggesting it alters cellular ion balance and metabolic pathways .
- Food Intake Modulation : Studies have demonstrated that administration of 2,5-AM can induce a dose-dependent increase in food intake in rats. Doses ranging from 50 to 800 mg/kg have been effective in modulating plasma glucose levels and increasing ketone bodies and free fatty acids without significantly affecting triglycerides .
Appetite Regulation
2,5-AM has been identified as a potential appetite modifier. Its ability to stimulate food intake makes it relevant for addressing conditions such as obesity and anorexia.
Mechanism of Action:
- The compound appears to create a metabolic state resembling fasting, thereby stimulating appetite. This effect is particularly pronounced when administered during fasting periods .
- It has been suggested that 2,5-AM may be used therapeutically to treat overeating or obesity by regulating feeding behavior through its metabolic effects on the liver .
Therapeutic Potential
The unique properties of 2,5-AM suggest several therapeutic applications beyond appetite modulation:
Potential Uses:
- Weight Management : By influencing food intake and energy metabolism, 2,5-AM could be explored as a treatment option for weight management in obese patients.
- Diabetes Management : Its role in inhibiting gluconeogenesis may offer insights into developing new strategies for managing diabetes by controlling blood sugar levels .
Synthesis and Structural Analysis
The synthesis of 2,5-AM can be achieved through various methods:
- Reduction of D-mannose or D-fructose using reducing agents like sodium borohydride.
- Enzymatic conversion of D-mannose using specific enzymes.
Comparison with Similar Compounds:
Compound | Structure Similarity | Primary Application |
---|---|---|
This compound | Fructose analogue | Appetite stimulation and metabolic research |
D-fructose | High | Energy source |
Mannitol | Moderate | Diuretic and sweetener |
Case Studies
Several studies highlight the efficacy of 2,5-AM in various applications:
- Food Intake Study : A study involving rats showed that administering 200 mg/kg of 2,5-AM significantly increased food intake during fasting periods while decreasing plasma glucose levels and increasing ketone bodies .
- Hepatic Function Analysis : Research indicated that 2,5-AM inhibits gluconeogenesis and glycogenolysis effectively in isolated rat hepatocytes, providing insights into its potential use in metabolic disorder treatments .
作用機序
2,5-Anhydro-D-mannitol exerts its effects by inhibiting gluconeogenesis and glycogenolysis in the liver. It is phosphorylated in the liver, which decreases available ATP and signals eating behavior through a vagal nerve mechanism . Additionally, it acts as a competitive inhibitor of fructose uptake, suppressing cell growth in certain cancer cells by targeting the GLUT5 transporter .
類似化合物との比較
A. 1,5-Anhydro-D-Fructose
- Synthesis : Derived from starch via enzymatic degradation, contrasting with 2,5-AM’s glucosamine-based synthesis .
B. 2,5-Anhydro-D-Glucitol
- Structure: Differs in stereochemistry at C3 (gluco vs. manno configuration) .
- Enzymatic Recognition : Less effective in inhibiting phosphoglucomutase compared to 2,5-AM (Ki = 3.2 mM vs. 2.8 mM) .
C. 2-Deoxy-D-Glucose
- Mechanism : Blocks glycolysis via competitive inhibition of hexokinase, whereas 2,5-AM primarily targets hepatic enzymes and ATP depletion .
Metabolic and Functional Comparisons
A. Fructose Analogs Targeting GLUT5
- 2,5-AM Derivatives: C-3 modifications (e.g., sulfonamides, thioureas) enhance GLUT5 binding for drug delivery. For example, 3-deoxy-3-[N-(4-fluorobenzenesulfonamide)amino]-2,5-AM shows >50% uptake inhibition in GLUT5-expressing cells .
- 1-Amino-2,5-AM: Synthesized via nitrous acid deamination, it serves as a precursor for glycoconjugates but exhibits lower transporter affinity than fluorinated derivatives .
B. Anti-Aging Compounds
- Rapamycin : Extends CLS via mTOR inhibition, while 2,5-AM acts through glycolytic intermediate accumulation .
- Curcumin : Activates stress-response pathways, unlike 2,5-AM’s direct metabolic interference .
Table 2: Enzyme Modulation by Phosphorylated 2,5-AM Intermediates
A. GLUT5-Targeted Drug Delivery
- Coumarin Glycoconjugates : 2,5-AM derivatives (e.g., ManCou) exhibit GLUT5-specific uptake, with fluorescence intensity correlating to coumarin functionalization .
- Fluorinated Derivatives: 3-deoxy-3-[N-(5-fluoro-2,4-dinitrophenyl)amino]-2,5-AM demonstrates enhanced binding in molecular dynamics simulations .
B. Anti-Aging Therapeutics
生物活性
2,5-Anhydro-D-mannitol (2,5-AM) is a carbohydrate derivative that has garnered attention for its biological activities, particularly in relation to glucose metabolism and appetite regulation. This article synthesizes findings from various studies to provide a comprehensive overview of the biological activity of 2,5-AM, including its mechanisms of action, effects on food intake, and potential therapeutic applications.
Chemical Structure and Properties
2,5-AM is a C2-symmetric fructose analogue that exists primarily in a furanose ring form. This unique structure allows it to interact effectively with glucose transporters, particularly GLUT5. The compound's symmetrical nature means that positions 1 and 6 are equivalent, which is advantageous for labeling and studying its transport characteristics in biological systems .
Interaction with GLUT5 Transporter
2,5-AM acts as a substrate for the GLUT5 transporter, which is responsible for the transport of fructose across cell membranes. Studies have shown that 2,5-AM can inhibit the uptake of D-fructose by GLUT5, with an IC50 value significantly lower than that for D-fructose itself. For instance, one study reported an IC50 of approximately 20 mM for a derivative of 2,5-AM compared to about 300 mM for D-fructose . This suggests that 2,5-AM may have a higher affinity for GLUT5 than fructose.
Effects on Metabolism
Research indicates that 2,5-AM influences metabolic pathways by inhibiting gluconeogenesis and glycogenolysis in hepatocytes. In experiments involving fasted rats, administration of 2,5-AM resulted in decreased plasma glucose levels and increased concentrations of ketone bodies and free fatty acids . These effects suggest that 2,5-AM mimics a fasting state, potentially leading to increased food intake as the body seeks to restore energy balance.
Appetite Regulation
One of the notable effects of 2,5-AM is its ability to increase food intake. In studies where rats were administered varying doses (50-800 mg/kg), a dose-dependent increase in food consumption was observed following administration . This effect was attributed to the compound's central action on appetite regulation and its ability to alter metabolic states.
Case Studies
- Food Intake Study : A study demonstrated that administering 200 mg/kg of 2,5-AM to rats led to significant increases in food intake during fasting periods. The compound's ability to mimic fasting conditions was highlighted as a key factor in this response .
- Gluconeogenesis Inhibition : Another investigation focused on the inhibitory effects of 2,5-AM on gluconeogenesis from lactate and pyruvate in isolated hepatocytes from fasted rats. The results indicated that 2,5-AM effectively reduced glucose production under these conditions .
Research Findings Summary
Therapeutic Potential
Given its unique properties and effects on metabolism and appetite regulation, 2,5-AM holds potential as a therapeutic agent for conditions such as obesity and diabetes. Its ability to modulate glucose levels and influence feeding behavior could be beneficial in developing treatments aimed at managing these metabolic disorders.
Q & A
Basic Research Questions
Q. What experimental methods are recommended for synthesizing and characterizing 2,5-anhydro-D-mannitol in metabolic studies?
- Methodological Answer : Synthesis often involves catalytic dehydration of D-mannitol derivatives under controlled acidic conditions. Characterization requires nuclear magnetic resonance (NMR) spectroscopy (¹H and ¹³C) to confirm stereochemistry and purity, complemented by high-performance liquid chromatography (HPLC) for quantitative analysis . For metabolic studies, radiolabeled ¹⁴C- or ³H-tracers are used to track hepatic phosphorylation and downstream pathways .
Q. How should researchers design in vivo experiments to study this compound’s effects on gluconeogenesis inhibition?
- Methodological Answer : Utilize fasted rodent models to isolate gluconeogenic activity. Administer this compound intravenously (IV) or intraperitoneally (IP), and measure plasma glucose, lactate, and pyruvate levels. Liver tissue should be assayed for gluconeogenic enzyme activity (e.g., PEPCK, G6Pase) and ATP content via ³¹P-NMR spectroscopy to correlate metabolic flux with energy depletion .
Advanced Research Questions
Q. What mechanisms underlie the contradiction between this compound’s ATP-depleting effects and its differential outcomes in fed vs. fasted states?
- Methodological Answer : In fed states, hepatic glycogen reserves buffer ATP decline, masking gluconeogenesis suppression. In fasting, glycogen depletion amplifies ATP loss, triggering compensatory feeding behavior. Experimental validation involves:
- Comparing ATP kinetics (via ³¹P-NMR) and gluconeogenic output in fed vs. fasted rats.
- Blocking fatty acid oxidation (e.g., with etomoxir) to isolate carbohydrate metabolism effects .
Q. How can researchers reconcile discrepancies in this compound’s reported effects on food intake across species (e.g., rats vs. Syrian hamsters)?
- Methodological Answer : Species-specific vagal nerve sensitivity may explain differences. Use hepatic vagotomy models in rats to test if feeding responses are abolished. In hamsters, pair this compound with indirect calorimetry to assess energy expenditure and substrate oxidation (e.g., respiratory quotient analysis). Contrast Fos-like immunoreactivity in hypothalamic nuclei to identify neural pathway variations .
Q. What experimental strategies address the compound’s rapid renal clearance, which complicates chronic metabolic studies?
- Methodological Answer : Optimize dosing regimens (e.g., continuous IV infusion) to maintain steady-state plasma levels. Alternatively, use osmotic pumps for sustained release. Monitor urinary excretion of this compound and its metabolites (e.g., D-mannitol-1-phosphate) via LC-MS/MS to adjust dosing .
Q. Data Analysis and Interpretation
Q. How should researchers analyze conflicting data on this compound’s role in carbohydrate-protein interactions?
- Methodological Answer : Use surface plasmon resonance (SPR) or isothermal titration calorimetry (ITC) to quantify binding affinities with glycoproteins. Pair with molecular docking simulations to identify structural motifs (e.g., hydroxyl group positioning) critical for interactions. Validate in cell-based assays (e.g., hepatocyte membrane polarization studies) .
Q. What statistical approaches are recommended for time-series data on hepatic ATP decline and feeding behavior?
- Methodological Answer : Apply mixed-effects models to account for inter-individual variability in ATP kinetics. Use cross-correlation analysis to determine temporal lag between ATP nadir and feeding onset. For small-sample studies, Bayesian hierarchical models improve robustness .
Q. Technical Notes
- Chemical Stability : Store this compound at -20°C in anhydrous DMSO or lyophilized form to prevent hygroscopic degradation .
- In Vivo Solubility : For IV administration, dissolve in saline (≤50 mg/mL) with gentle heating (37°C). For oral gavage, use 5% carboxymethyl cellulose (CMC-Na) to enhance bioavailability .
特性
IUPAC Name |
2,5-bis(hydroxymethyl)oxolane-3,4-diol | |
---|---|---|
Details | Computed by Lexichem TK 2.7.0 (PubChem release 2021.05.07) | |
Source | PubChem | |
URL | https://pubchem.ncbi.nlm.nih.gov | |
Description | Data deposited in or computed by PubChem | |
InChI |
InChI=1S/C6H12O5/c7-1-3-5(9)6(10)4(2-8)11-3/h3-10H,1-2H2 | |
Details | Computed by InChI 1.0.6 (PubChem release 2021.05.07) | |
Source | PubChem | |
URL | https://pubchem.ncbi.nlm.nih.gov | |
Description | Data deposited in or computed by PubChem | |
InChI Key |
MCHWWJLLPNDHGL-UHFFFAOYSA-N | |
Details | Computed by InChI 1.0.6 (PubChem release 2021.05.07) | |
Source | PubChem | |
URL | https://pubchem.ncbi.nlm.nih.gov | |
Description | Data deposited in or computed by PubChem | |
Canonical SMILES |
C(C1C(C(C(O1)CO)O)O)O | |
Details | Computed by OEChem 2.3.0 (PubChem release 2021.05.07) | |
Source | PubChem | |
URL | https://pubchem.ncbi.nlm.nih.gov | |
Description | Data deposited in or computed by PubChem | |
Molecular Formula |
C6H12O5 | |
Details | Computed by PubChem 2.1 (PubChem release 2021.05.07) | |
Source | PubChem | |
URL | https://pubchem.ncbi.nlm.nih.gov | |
Description | Data deposited in or computed by PubChem | |
DSSTOX Substance ID |
DTXSID60961441 | |
Record name | 2,5-Anhydrohexitol | |
Source | EPA DSSTox | |
URL | https://comptox.epa.gov/dashboard/DTXSID60961441 | |
Description | DSSTox provides a high quality public chemistry resource for supporting improved predictive toxicology. | |
Molecular Weight |
164.16 g/mol | |
Details | Computed by PubChem 2.1 (PubChem release 2021.05.07) | |
Source | PubChem | |
URL | https://pubchem.ncbi.nlm.nih.gov | |
Description | Data deposited in or computed by PubChem | |
CAS No. |
41107-82-8 | |
Record name | 2,5-Anhydro-D-mannitol | |
Source | DTP/NCI | |
URL | https://dtp.cancer.gov/dtpstandard/servlet/dwindex?searchtype=NSC&outputformat=html&searchlist=129241 | |
Description | The NCI Development Therapeutics Program (DTP) provides services and resources to the academic and private-sector research communities worldwide to facilitate the discovery and development of new cancer therapeutic agents. | |
Explanation | Unless otherwise indicated, all text within NCI products is free of copyright and may be reused without our permission. Credit the National Cancer Institute as the source. | |
Record name | 2,5-Anhydrohexitol | |
Source | EPA DSSTox | |
URL | https://comptox.epa.gov/dashboard/DTXSID60961441 | |
Description | DSSTox provides a high quality public chemistry resource for supporting improved predictive toxicology. | |
Retrosynthesis Analysis
AI-Powered Synthesis Planning: Our tool employs the Template_relevance Pistachio, Template_relevance Bkms_metabolic, Template_relevance Pistachio_ringbreaker, Template_relevance Reaxys, Template_relevance Reaxys_biocatalysis model, leveraging a vast database of chemical reactions to predict feasible synthetic routes.
One-Step Synthesis Focus: Specifically designed for one-step synthesis, it provides concise and direct routes for your target compounds, streamlining the synthesis process.
Accurate Predictions: Utilizing the extensive PISTACHIO, BKMS_METABOLIC, PISTACHIO_RINGBREAKER, REAXYS, REAXYS_BIOCATALYSIS database, our tool offers high-accuracy predictions, reflecting the latest in chemical research and data.
Strategy Settings
Precursor scoring | Relevance Heuristic |
---|---|
Min. plausibility | 0.01 |
Model | Template_relevance |
Template Set | Pistachio/Bkms_metabolic/Pistachio_ringbreaker/Reaxys/Reaxys_biocatalysis |
Top-N result to add to graph | 6 |
Feasible Synthetic Routes
試験管内研究製品の免責事項と情報
BenchChemで提示されるすべての記事および製品情報は、情報提供を目的としています。BenchChemで購入可能な製品は、生体外研究のために特別に設計されています。生体外研究は、ラテン語の "in glass" に由来し、生物体の外で行われる実験を指します。これらの製品は医薬品または薬として分類されておらず、FDAから任何の医療状態、病気、または疾患の予防、治療、または治癒のために承認されていません。これらの製品を人間または動物に体内に導入する形態は、法律により厳格に禁止されています。これらのガイドラインに従うことは、研究と実験において法的および倫理的な基準の遵守を確実にするために重要です。