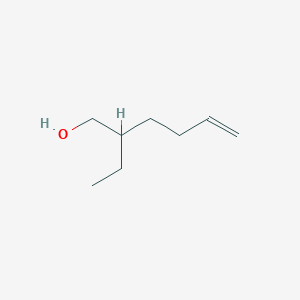
2-エチルヘキサ-5-エン-1-オール
概要
説明
2-Ethylhex-5-en-1-ol is an organic compound with the molecular formula C8H16O. It is a primary alcohol with a double bond located at the fifth carbon atom. This compound is known for its unique chemical properties and is used in various industrial and scientific applications.
科学的研究の応用
2-Ethylhex-5-en-1-ol has several applications in scientific research:
Chemistry: It is used as a chiral building block in the synthesis of complex organic molecules.
Biology: The compound’s enantiomers are studied for their biological activity and potential use in pharmaceuticals.
Medicine: Research is ongoing to explore its potential as a precursor in the synthesis of bioactive compounds.
Industry: It is used in the production of fragrances, flavors, and other specialty chemicals.
Safety and Hazards
作用機序
Target of Action
The primary target of 2-Ethylhex-5-en-1-ol is lipase, an enzyme that catalyzes the hydrolysis of fats . Lipase plays a crucial role in the digestion, transport, and processing of dietary lipids in most living organisms .
Mode of Action
2-Ethylhex-5-en-1-ol interacts with lipase through a process called transesterification . In this process, both the ® and (S) enantiomers of 2-Ethylhex-5-en-1-ol are prepared in good yields and high enantiomeric excess via lipase-catalyzed transacetylation of racemic alcohols with vinyl acetate . This interaction results in changes in the enantioselectivity and reaction rate, which are influenced by various experimental conditions .
Pharmacokinetics
The lipase-catalyzed transesterification process suggests that the compound may be metabolized in the body through enzymatic reactions . The impact of these properties on the bioavailability of 2-Ethylhex-5-en-1-ol would require further investigation.
Result of Action
The molecular and cellular effects of 2-Ethylhex-5-en-1-ol’s action primarily involve changes in the enantioselectivity and reaction rate of lipase-catalyzed reactions . The compound’s interaction with lipase results in the production of both enantiomers of 2-Ethylhex-5-en-1-ol in good yields .
Action Environment
The action, efficacy, and stability of 2-Ethylhex-5-en-1-ol are influenced by various environmental factors. For instance, the temperature and the type of solvent used can affect the enantioselectivity and reaction rate of the lipase-catalyzed transesterification process .
生化学分析
Biochemical Properties
2-Ethylhex-5-en-1-ol has been prepared in good yields and high enantiomeric excess via lipase-catalyzed transacetylation of racemic alcohols with vinyl acetate . The enantioselectivity of the lipase is greatly enhanced by the presence of a terminal double bond . This suggests that 2-Ethylhex-5-en-1-ol may interact with enzymes such as lipases in biochemical reactions.
Cellular Effects
It is known that 2-Ethylhex-5-en-1-ol is used in the synthesis of phthalates, which are known endocrine disrupters . Endocrine disrupters can have significant effects on cell function, including impacts on cell signaling pathways, gene expression, and cellular metabolism.
Molecular Mechanism
The lipase-catalyzed transacetylation process suggests that 2-Ethylhex-5-en-1-ol may interact with enzymes and other biomolecules in a way that influences their activity .
準備方法
Synthetic Routes and Reaction Conditions: 2-Ethylhex-5-en-1-ol can be synthesized through several methods. One common approach involves the lipase-catalyzed transesterification of racemic alcohols with vinyl acetate. This method allows for the preparation of both ® and (S) enantiomers of 2-Ethylhex-5-en-1-ol in good yields and high enantiomeric excess . The reaction conditions typically involve the use of lipase PSL or PLF, solvents like dichloromethane, and temperatures ranging from 30°C to -30°C .
Industrial Production Methods: In industrial settings, the production of 2-Ethylhex-5-en-1-ol may involve large-scale chemical synthesis using similar enzymatic processes. The optimization of reaction conditions, such as temperature and solvent choice, is crucial to achieve high yields and purity.
化学反応の分析
Types of Reactions: 2-Ethylhex-5-en-1-ol undergoes various chemical reactions, including:
Oxidation: The primary alcohol group can be oxidized to form aldehydes or carboxylic acids.
Reduction: The double bond can be reduced to form 2-Ethylhexan-1-ol.
Substitution: The hydroxyl group can be substituted with other functional groups through nucleophilic substitution reactions.
Common Reagents and Conditions:
Oxidation: Common oxidizing agents include potassium permanganate and chromium trioxide.
Reduction: Catalytic hydrogenation using palladium or platinum catalysts is often employed.
Substitution: Reagents like thionyl chloride or phosphorus tribromide can be used for substitution reactions.
Major Products Formed:
Oxidation: 2-Ethylhex-5-enal or 2-Ethylhexanoic acid.
Reduction: 2-Ethylhexan-1-ol.
Substitution: Various substituted derivatives depending on the reagent used.
類似化合物との比較
2-Ethylhexan-1-ol: A saturated analog of 2-Ethylhex-5-en-1-ol, lacking the double bond.
2-Hexen-1-ol: A similar compound with a different alkyl chain structure.
Uniqueness: 2-Ethylhex-5-en-1-ol is unique due to the presence of both a primary alcohol group and a double bond. This combination of functional groups provides distinct chemical reactivity and makes it valuable in various applications.
特性
IUPAC Name |
2-ethylhex-5-en-1-ol | |
---|---|---|
Source | PubChem | |
URL | https://pubchem.ncbi.nlm.nih.gov | |
Description | Data deposited in or computed by PubChem | |
InChI |
InChI=1S/C8H16O/c1-3-5-6-8(4-2)7-9/h3,8-9H,1,4-7H2,2H3 | |
Source | PubChem | |
URL | https://pubchem.ncbi.nlm.nih.gov | |
Description | Data deposited in or computed by PubChem | |
InChI Key |
SKGWHSIXVYWAOD-UHFFFAOYSA-N | |
Source | PubChem | |
URL | https://pubchem.ncbi.nlm.nih.gov | |
Description | Data deposited in or computed by PubChem | |
Canonical SMILES |
CCC(CCC=C)CO | |
Source | PubChem | |
URL | https://pubchem.ncbi.nlm.nih.gov | |
Description | Data deposited in or computed by PubChem | |
Molecular Formula |
C8H16O | |
Source | PubChem | |
URL | https://pubchem.ncbi.nlm.nih.gov | |
Description | Data deposited in or computed by PubChem | |
Molecular Weight |
128.21 g/mol | |
Source | PubChem | |
URL | https://pubchem.ncbi.nlm.nih.gov | |
Description | Data deposited in or computed by PubChem | |
Retrosynthesis Analysis
AI-Powered Synthesis Planning: Our tool employs the Template_relevance Pistachio, Template_relevance Bkms_metabolic, Template_relevance Pistachio_ringbreaker, Template_relevance Reaxys, Template_relevance Reaxys_biocatalysis model, leveraging a vast database of chemical reactions to predict feasible synthetic routes.
One-Step Synthesis Focus: Specifically designed for one-step synthesis, it provides concise and direct routes for your target compounds, streamlining the synthesis process.
Accurate Predictions: Utilizing the extensive PISTACHIO, BKMS_METABOLIC, PISTACHIO_RINGBREAKER, REAXYS, REAXYS_BIOCATALYSIS database, our tool offers high-accuracy predictions, reflecting the latest in chemical research and data.
Strategy Settings
Precursor scoring | Relevance Heuristic |
---|---|
Min. plausibility | 0.01 |
Model | Template_relevance |
Template Set | Pistachio/Bkms_metabolic/Pistachio_ringbreaker/Reaxys/Reaxys_biocatalysis |
Top-N result to add to graph | 6 |
Feasible Synthetic Routes
Q1: What makes the enzymatic resolution of 2-ethylhex-5-en-1-ol particularly interesting?
A: The research highlights the impact of structural features on the enantioselectivity of lipase enzymes. Specifically, the presence of the terminal double bond in 2-ethylhex-5-en-1-ol significantly enhances the ability of lipase PS to distinguish between its enantiomers compared to its saturated counterpart, 2-ethylhexan-1-ol. [] This finding is significant because obtaining enantiomerically pure compounds is crucial in fields like pharmaceuticals and asymmetric synthesis.
Q2: How does temperature impact the enzymatic resolution of 2-ethylhex-5-en-1-ol?
A: The study demonstrates that lowering the reaction temperature to -30°C leads to increased enantioselectivity of lipase PS without compromising its catalytic activity. [] This is a valuable finding as it allows for the production of both (R)-(-) and (S)-(+) enantiomers of 2-ethylhex-5-en-1-ol with high enantiomeric excess, expanding its potential applications.
試験管内研究製品の免責事項と情報
BenchChemで提示されるすべての記事および製品情報は、情報提供を目的としています。BenchChemで購入可能な製品は、生体外研究のために特別に設計されています。生体外研究は、ラテン語の "in glass" に由来し、生物体の外で行われる実験を指します。これらの製品は医薬品または薬として分類されておらず、FDAから任何の医療状態、病気、または疾患の予防、治療、または治癒のために承認されていません。これらの製品を人間または動物に体内に導入する形態は、法律により厳格に禁止されています。これらのガイドラインに従うことは、研究と実験において法的および倫理的な基準の遵守を確実にするために重要です。