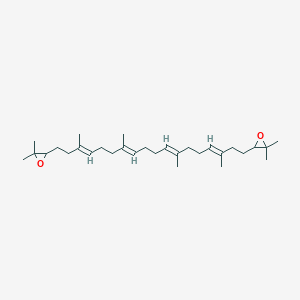
2,3-オキシドスクアレン
概要
説明
科学的研究の応用
Dioxidosqualene has a wide range of scientific research applications:
Chemistry: Used as a precursor in the synthesis of various triterpenoids and sterols.
Biology: Studied for its role in cell membrane integrity and function.
Industry: Utilized in the production of biofuels and bioproducts through microbial fermentation.
作用機序
Target of Action
The primary target of Dioxidosqualene is the enzyme Oxidosqualene Cyclase (OSC) . OSCs are key rate-limiting enzymes in the biosynthesis of triterpenes . They catalyze the cyclization of 2,3-oxidosqualene into sterols and triterpenes with different skeletons .
Mode of Action
Dioxidosqualene interacts with its target, OSC, by serving as a substrate for the enzyme . In the enzymatic mechanism, the 2,3-oxidosqualene substrate adopts a chair–chair–chair conformation, leading to the formation of the dammarenyl carbocation intermediate before cyclization . This gives rise to various triterpenoid types such as ursane, oleanane, lupane, and other diverse skeletal structures .
Biochemical Pathways
Dioxidosqualene plays a crucial role in the triterpene biosynthetic pathway . It is involved in the initial steps of triterpene biosynthesis, subsequently influencing defense against certain biotic stressors through the modulation of downstream triterpene glycoside compounds . The cyclization of 2,3-oxidosqualene is a pivotal step in triterpenoid biosynthesis, catalyzed by OSC enzymes in plants .
Pharmacokinetics
Computational methods can significantly advance the study of chemical admet properties, offering both an economical and a time-efficient approach .
Result of Action
The molecular and cellular effects of Dioxidosqualene’s action involve the production of various triterpenoids . For instance, in cancer cell lines, the inhibition of SQLE, a target of Dioxidosqualene, caused a toxic accumulation of the substrate squalene . Moreover, certain triterpenes within clusters displayed a significant negative correlation with larval mass, indicating potential toxic effects .
生化学分析
Biochemical Properties
Dioxidosqualene is involved in the biosynthesis of triterpenes, a class of bioactive compounds with diverse biological functions . It is a substrate for oxidosqualene cyclases (OSCs), enzymes that catalyze the cyclization of 2,3-oxidosqualene to form various triterpenoid types . The interaction between dioxidosqualene and OSCs is pivotal in the initial steps of triterpene biosynthesis .
Cellular Effects
The effects of dioxidosqualene on cells and cellular processes are largely related to its role in triterpene biosynthesis. Triterpenes, the products of this biosynthesis, play pivotal roles in plant defense against biotic stressors . Therefore, the presence of dioxidosqualene and its conversion into triterpenes can influence cell function, including impacts on cell signaling pathways, gene expression, and cellular metabolism .
Molecular Mechanism
The mechanism of action of dioxidosqualene involves its conversion into various triterpenoids through the action of OSCs . This enzymatic mechanism involves the 2,3-oxidosqualene substrate adopting a specific conformation, leading to the formation of an intermediate before cyclization, giving rise to various triterpenoid types . This process involves binding interactions with OSCs, and can lead to changes in gene expression related to triterpene biosynthesis .
Metabolic Pathways
Dioxidosqualene is involved in the metabolic pathway of triterpene biosynthesis . It interacts with OSCs, which serve as gatekeepers in this biosynthesis . The interaction between dioxidosqualene and these enzymes can influence metabolic flux and metabolite levels .
準備方法
Synthetic Routes and Reaction Conditions
Dioxidosqualene can be synthesized through the epoxidation of squalene. One common method involves the use of N-bromosuccinimide (NBS) in tetrahydrofuran (THF) under a nitrogen atmosphere. The reaction is typically carried out at low temperatures (0°C) to ensure the selective formation of the epoxide .
Industrial Production Methods
Industrial production of dioxidosqualene often involves biotechnological approaches, such as the genetic engineering of microorganisms like Saccharomyces cerevisiae. By manipulating the ergosterol synthesis pathway, researchers can enhance the production of dioxidosqualene and other triterpenoids .
化学反応の分析
Types of Reactions
Dioxidosqualene undergoes various chemical reactions, including:
Oxidation: Conversion to triterpenoids and sterols.
Cyclization: Formation of complex triterpene structures through the action of oxidosqualene cyclase enzymes.
Common Reagents and Conditions
Oxidation: Hydrogen peroxide (H₂O₂) or peracids are commonly used oxidizing agents.
Cyclization: Enzymatic reactions involving oxidosqualene cyclase under physiological conditions.
Major Products Formed
Triterpenoids: Such as β-amyrin, lupeol, and dammarenediol II.
Sterols: Including cholesterol and ergosterol.
類似化合物との比較
Similar Compounds
2,3-Oxidosqualene: A precursor to dioxidosqualene, involved in similar biosynthetic pathways.
Squalene: The parent compound, widely used in cosmetics and pharmaceuticals.
Lanosterol: A sterol derived from the cyclization of 2,3-oxidosqualene.
Uniqueness
Dioxidosqualene is unique due to its dual epoxide groups, which allow for the formation of a diverse array of triterpenoid and sterol structures. This dual functionality makes it a valuable intermediate in both natural biosynthetic pathways and industrial applications .
特性
IUPAC Name |
3-[(3E,7E,11E,15E)-18-(3,3-dimethyloxiran-2-yl)-3,7,12,16-tetramethyloctadeca-3,7,11,15-tetraenyl]-2,2-dimethyloxirane | |
---|---|---|
Source | PubChem | |
URL | https://pubchem.ncbi.nlm.nih.gov | |
Description | Data deposited in or computed by PubChem | |
InChI |
InChI=1S/C30H50O2/c1-23(15-11-17-25(3)19-21-27-29(5,6)31-27)13-9-10-14-24(2)16-12-18-26(4)20-22-28-30(7,8)32-28/h13-14,17-18,27-28H,9-12,15-16,19-22H2,1-8H3/b23-13+,24-14+,25-17+,26-18+ | |
Source | PubChem | |
URL | https://pubchem.ncbi.nlm.nih.gov | |
Description | Data deposited in or computed by PubChem | |
InChI Key |
KABSNIWLJXCBGG-TXLDAEQNSA-N | |
Source | PubChem | |
URL | https://pubchem.ncbi.nlm.nih.gov | |
Description | Data deposited in or computed by PubChem | |
Canonical SMILES |
CC(=CCCC=C(C)CCC=C(C)CCC1C(O1)(C)C)CCC=C(C)CCC2C(O2)(C)C | |
Source | PubChem | |
URL | https://pubchem.ncbi.nlm.nih.gov | |
Description | Data deposited in or computed by PubChem | |
Isomeric SMILES |
C/C(=C\CC/C=C(/CC/C=C(/CCC1OC1(C)C)\C)\C)/CC/C=C(/CCC2OC2(C)C)\C | |
Source | PubChem | |
URL | https://pubchem.ncbi.nlm.nih.gov | |
Description | Data deposited in or computed by PubChem | |
Molecular Formula |
C30H50O2 | |
Source | PubChem | |
URL | https://pubchem.ncbi.nlm.nih.gov | |
Description | Data deposited in or computed by PubChem | |
Molecular Weight |
442.7 g/mol | |
Source | PubChem | |
URL | https://pubchem.ncbi.nlm.nih.gov | |
Description | Data deposited in or computed by PubChem | |
CAS No. |
31063-19-1 | |
Record name | 2,3,22,23-Dioxidosqualene | |
Source | ChemIDplus | |
URL | https://pubchem.ncbi.nlm.nih.gov/substance/?source=chemidplus&sourceid=0031063191 | |
Description | ChemIDplus is a free, web search system that provides access to the structure and nomenclature authority files used for the identification of chemical substances cited in National Library of Medicine (NLM) databases, including the TOXNET system. | |
Retrosynthesis Analysis
AI-Powered Synthesis Planning: Our tool employs the Template_relevance Pistachio, Template_relevance Bkms_metabolic, Template_relevance Pistachio_ringbreaker, Template_relevance Reaxys, Template_relevance Reaxys_biocatalysis model, leveraging a vast database of chemical reactions to predict feasible synthetic routes.
One-Step Synthesis Focus: Specifically designed for one-step synthesis, it provides concise and direct routes for your target compounds, streamlining the synthesis process.
Accurate Predictions: Utilizing the extensive PISTACHIO, BKMS_METABOLIC, PISTACHIO_RINGBREAKER, REAXYS, REAXYS_BIOCATALYSIS database, our tool offers high-accuracy predictions, reflecting the latest in chemical research and data.
Strategy Settings
Precursor scoring | Relevance Heuristic |
---|---|
Min. plausibility | 0.01 |
Model | Template_relevance |
Template Set | Pistachio/Bkms_metabolic/Pistachio_ringbreaker/Reaxys/Reaxys_biocatalysis |
Top-N result to add to graph | 6 |
Feasible Synthetic Routes
試験管内研究製品の免責事項と情報
BenchChemで提示されるすべての記事および製品情報は、情報提供を目的としています。BenchChemで購入可能な製品は、生体外研究のために特別に設計されています。生体外研究は、ラテン語の "in glass" に由来し、生物体の外で行われる実験を指します。これらの製品は医薬品または薬として分類されておらず、FDAから任何の医療状態、病気、または疾患の予防、治療、または治癒のために承認されていません。これらの製品を人間または動物に体内に導入する形態は、法律により厳格に禁止されています。これらのガイドラインに従うことは、研究と実験において法的および倫理的な基準の遵守を確実にするために重要です。