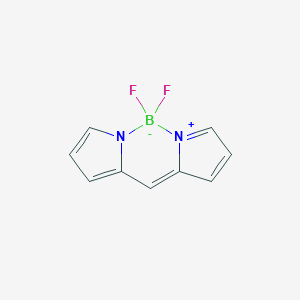
Bodipy
概要
説明
BODIPY (Boron-dipyrromethene) is a type of fluorescent dye that is particularly useful for preparing conjugates of peptides, nucleotides, oligonucleotides, drugs, toxins, sphingolipids, and other low molecular weight ligands that contain aliphatic amines .
Synthesis Analysis
BODIPY dyes have emerged as a valuable category of luminogens for optoelectronic applications due to their properties such as good fluorescence quantum yield, broad absorption with high molar extinction coefficient, excellent photo-chemical and thermal stability, remarkable redox properties, easy structural modifications, and good solubility . BODIPY dyes have three main reactive sites: α-, β-pyrrolic and meso positions, which enable modifications for the synthesis of various donor–acceptor BODIPY dyes .Molecular Structure Analysis
The polarity and structure of BODIPYs have been analyzed using a fragment approach and the NASAWIN software . The database for the analysis comprises 110 compounds, each characterized by the spectral data on the absorption and fluorescence in 8–30 solvents .Chemical Reactions Analysis
BODIPY-derived triplet photosensitizers have been reviewed and classified based on ISC mechanisms, including the heavy atom effect, exciton coupling, and charge recombination (CR)-induced ISC, using a spin converter and radical enhanced ISC .Physical And Chemical Properties Analysis
BODIPY dyes have been investigated for their photophysical properties and photostability . Absorption and emission spectra for a wide group of solvents of different properties for the analyzed BODIPY derivatives were investigated in order to verify their suitability for photopharmacological applications .科学的研究の応用
Dye-Sensitized Solar Cells (DSSCs)
BODIPY dyes have been used in dye-sensitized solar cells (DSSCs). Their good fluorescence quantum yield, broad absorption with high molar extinction coefficient, excellent photo-chemical and thermal stability, remarkable redox properties, easy structural modifications, and good solubility make them an important class of chromophores for application in DSSCs .
Photodynamic Therapy (PDT)
BODIPY dyes have also found applications in photodynamic therapy (PDT). Their strong absorption and emission in the visible and near-infrared regions of the electromagnetic spectrum, strong fluorescence, and supreme photostability make them suitable for PDT .
Bioimaging
BODIPY-based molecules have attracted attention as probes in applications like imaging due to their unique properties. They have been employed in areas like bioimaging .
Chemosensors
BODIPY dyes have been used in the development of chemosensors. Their excellent photo-chemical and thermal stability, remarkable redox properties, and easy structural modifications make them suitable for this application .
Electron-Transporting Materials
BODIPY dyes have been used in the development of electron-transporting materials. Their good fluorescence quantum yield, broad absorption with high molar extinction coefficient, and excellent photo-chemical and thermal stability make them suitable for this application .
Nonlinear Optics
BODIPY dyes have been used in nonlinear optics. Their good fluorescence quantum yield, broad absorption with high molar extinction coefficient, excellent photo-chemical and thermal stability, remarkable redox properties, easy structural modifications, and good solubility make them an important class of chromophores for application in nonlinear optics .
作用機序
Target of Action
BODIPY (Boron-dipyrromethene) is a class of organic dyes that have been extensively used as photosensitizers . The primary targets of BODIPY are intracellular organelles and tumor markers in tumor cells, which it targets for the purpose of early tumor detection .
Mode of Action
BODIPY interacts with its targets through a process known as Photodynamic Therapy (PDT). PDT relies on three key elements: light, a photosensitizer (in this case, BODIPY), and oxygen . Upon light irradiation, BODIPY generates reactive oxygen species, especially singlet oxygen from oxygen, to damage target diseases . The electronic-rich property possessed by the BODIPY core makes it useful for chemical modification, especially the electrophilic aromatic substitution with halogens (Br, I), which converts the highly fluorescent or low triplet transition molecule to a potent photosensitizer with efficient intersystem crossing capacity due to spin orbit coupling by the heavy atom effect .
Biochemical Pathways
BODIPY affects the biochemical pathways involved in the generation of reactive oxygen species. These reactive species then interact with various cellular components, leading to cell damage and death . Furthermore, BODIPY dyes can be engineered to emit fluorescence, allowing for real-time monitoring of their distribution and therapeutic effects .
Pharmacokinetics
It’s known that bodipy dyes have potent photostability and beneficial photophysical characteristics , which may influence their bioavailability and pharmacokinetic properties.
Result of Action
The result of BODIPY’s action is the damage and death of target cells, such as tumor cells . This is achieved through the generation of reactive oxygen species that cause cellular damage . BODIPY-based molecules have even emerged as candidates for cancer treatments .
Action Environment
The action of BODIPY can be influenced by environmental factors such as light and oxygen, which are essential components of its mechanism of action . BODIPY-based photocages that absorb visible/near-infrared (Vis/NIR) light offer advantages such as deeper tissue penetration and reduced bio-autofluorescence, making them highly suitable for various biomedical applications .
Safety and Hazards
将来の方向性
BODIPY dyes and their derivatives have been the focus of considerable research interest and rapidly growing . Future research will likely continue to explore the design of BODIPY dyes as triplet photosensitizers, with electronic properties tailored for solar energy conversion, photoredox catalysis, and photodynamic therapy .
特性
IUPAC Name |
2,2-difluoro-3-aza-1-azonia-2-boranuidatricyclo[7.3.0.03,7]dodeca-1(12),4,6,8,10-pentaene | |
---|---|---|
Source | PubChem | |
URL | https://pubchem.ncbi.nlm.nih.gov | |
Description | Data deposited in or computed by PubChem | |
InChI |
InChI=1S/C9H7BF2N2/c11-10(12)13-5-1-3-8(13)7-9-4-2-6-14(9)10/h1-7H | |
Source | PubChem | |
URL | https://pubchem.ncbi.nlm.nih.gov | |
Description | Data deposited in or computed by PubChem | |
InChI Key |
GUHHEAYOTAJBPT-UHFFFAOYSA-N | |
Source | PubChem | |
URL | https://pubchem.ncbi.nlm.nih.gov | |
Description | Data deposited in or computed by PubChem | |
Canonical SMILES |
[B-]1(N2C=CC=C2C=C3[N+]1=CC=C3)(F)F | |
Source | PubChem | |
URL | https://pubchem.ncbi.nlm.nih.gov | |
Description | Data deposited in or computed by PubChem | |
Molecular Formula |
C9H7BF2N2 | |
Source | PubChem | |
URL | https://pubchem.ncbi.nlm.nih.gov | |
Description | Data deposited in or computed by PubChem | |
Molecular Weight |
191.98 g/mol | |
Source | PubChem | |
URL | https://pubchem.ncbi.nlm.nih.gov | |
Description | Data deposited in or computed by PubChem | |
Product Name |
Bodipy |
Synthesis routes and methods
Procedure details
Retrosynthesis Analysis
AI-Powered Synthesis Planning: Our tool employs the Template_relevance Pistachio, Template_relevance Bkms_metabolic, Template_relevance Pistachio_ringbreaker, Template_relevance Reaxys, Template_relevance Reaxys_biocatalysis model, leveraging a vast database of chemical reactions to predict feasible synthetic routes.
One-Step Synthesis Focus: Specifically designed for one-step synthesis, it provides concise and direct routes for your target compounds, streamlining the synthesis process.
Accurate Predictions: Utilizing the extensive PISTACHIO, BKMS_METABOLIC, PISTACHIO_RINGBREAKER, REAXYS, REAXYS_BIOCATALYSIS database, our tool offers high-accuracy predictions, reflecting the latest in chemical research and data.
Strategy Settings
Precursor scoring | Relevance Heuristic |
---|---|
Min. plausibility | 0.01 |
Model | Template_relevance |
Template Set | Pistachio/Bkms_metabolic/Pistachio_ringbreaker/Reaxys/Reaxys_biocatalysis |
Top-N result to add to graph | 6 |
Feasible Synthetic Routes
Q & A
Q1: What is the basic structure of a BODIPY dye, and what are its key spectroscopic characteristics?
A1: BODIPY dyes consist of a dipyrrin core complexed with a boron difluoride (BF2) unit. [] They exhibit strong absorption in the visible region, narrow emission bands with high fluorescence quantum yields, good photostability, and often small Stokes shifts. []
Q2: How does the molecular formula and weight of a BODIPY dye relate to its spectroscopic properties?
A2: Specific substitutions on the dipyrrin core and modifications to the BF2 unit can significantly alter the molecular formula and weight of BODIPY dyes, leading to tailored spectroscopic properties. For instance, incorporating heavy atoms like iodine can redshift absorption and emission spectra. [, ]
Q3: Can you elaborate on the effect of substituents on the absorption and emission properties of BODIPY dyes?
A3: Absolutely! Studies show that electron-donating groups tend to redshift the spectra, while electron-withdrawing groups generally cause a blueshift. [, ] For example, introducing a dimethylaminostyryl group at the meso-position redshifts the absorption and emission spectra, as demonstrated in the synthesis of dimethylaminostyryl BODIPY-perylene tetracarboxylic derivative dyads. []
Q4: What is the significance of a large Stokes shift in BODIPYs for bioimaging applications?
A4: A large Stokes shift minimizes the overlap between the excitation and emission spectra, reducing self-quenching and background interference. This is particularly beneficial for bioimaging applications, where high signal-to-noise ratios are crucial. [, ]
Q5: How are BODIPY dyes employed in studying lipid dynamics?
A5: BODIPY-labeled lipids, like phosphatidylethanolamines, serve as fluorescent probes to track lipid movement and interactions within biological membranes. Their distribution in lipid bilayers provides insights into membrane curvature and fluidity. [, ]
Q6: Can BODIPY dyes be used to study intracellular processes other than lipid dynamics?
A6: Yes, by conjugating BODIPY dyes to molecules like brefeldin A (BFA), researchers can selectively stain organelles like the endoplasmic reticulum (ER) and Golgi complex in living cells. This facilitates the visualization and study of intracellular trafficking pathways. []
Q7: How do researchers achieve near-infrared (NIR) emission in BODIPY dyes, and what are the advantages of NIR probes for in vivo imaging?
A7: NIR emission can be achieved by extending the π-conjugation system of the BODIPY core, often through the incorporation of donor groups or annulation reactions. [, , ] NIR probes offer advantages for in vivo imaging due to deeper tissue penetration and reduced background autofluorescence. [, ]
Q8: How are BODIPY dyes being explored for photodynamic therapy (PDT)?
A8: BODIPY dyes can function as photosensitizers in PDT, generating singlet oxygen upon light irradiation, which leads to the destruction of targeted cells. Research is ongoing to enhance their singlet oxygen generation efficiency, for example, by introducing heavy atoms or creating twisted BODIPY derivatives. [, , , ]
Q9: Can you provide an example of how the structure of a BODIPY dye influences its application in PDT?
A9: In one study, a twisted BODIPY derivative with a long-lived triplet state displayed efficient singlet oxygen generation even under hypoxic conditions, making it a promising candidate for PDT. []
Q10: How does computational chemistry contribute to the understanding and development of BODIPY dyes?
A10: Density functional theory (DFT) calculations are valuable for predicting and rationalizing the spectroscopic properties of BODIPY derivatives. They provide insights into electronic structures, energy levels, and the impact of structural modifications. [, , , , , ]
Q11: What are the future prospects for BODIPY dyes in materials science and biomedical applications?
A11: BODIPYs hold significant promise for developing advanced materials like light-harvesting antennae, organic solar cells, and fluorescent sensors. In the biomedical realm, their potential extends to targeted drug delivery, bioimaging, and photodynamic therapy. [, , , , , , , ]
試験管内研究製品の免責事項と情報
BenchChemで提示されるすべての記事および製品情報は、情報提供を目的としています。BenchChemで購入可能な製品は、生体外研究のために特別に設計されています。生体外研究は、ラテン語の "in glass" に由来し、生物体の外で行われる実験を指します。これらの製品は医薬品または薬として分類されておらず、FDAから任何の医療状態、病気、または疾患の予防、治療、または治癒のために承認されていません。これらの製品を人間または動物に体内に導入する形態は、法律により厳格に禁止されています。これらのガイドラインに従うことは、研究と実験において法的および倫理的な基準の遵守を確実にするために重要です。