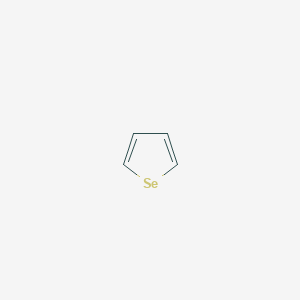
セレノフェン
概要
説明
Selenophene is an organic compound with the chemical formula C4H4Se. It is an unsaturated compound containing a five-member ring with four carbon atoms and one selenium atom. This compound is a selenium analog of furan (C4H4O) and thiophene (C4H4S). Selenophene is a colorless liquid and is one of the more common selenium heterocycles .
Synthetic Routes and Reaction Conditions:
Historical Synthesis: The first confirmed synthesis of selenophene was reported by Mazza and Solazzo in 1927.
Fiesselman Procedure: Substituted selenophenes can be synthesized using a Fiesselman procedure, where a β-chloro-aldehyde reacts with sodium selenide, followed by ethyl bromoacetate.
Cyclization-Based Strategies: Recent advances have employed cyclization-based synthetic strategies involving specific reaction partners and particularities.
Industrial Production Methods:
- Industrial production methods for selenophene are not extensively documented, but they likely involve scalable versions of the synthetic routes mentioned above, optimized for yield and cost-effectiveness.
Types of Reactions:
Electrophilic Substitution: Selenophene undergoes electrophilic substitution reactions. Electrophiles tend to attack at the carbon positions next to the selenium atom.
Oxidation: Selenophene can be oxidized to form selenophene 1,1-dioxide.
Common Reagents and Conditions:
Electrophilic Substitution: Common reagents include halogens and other electrophiles. Reaction conditions typically involve the presence of a catalyst and a suitable solvent.
Oxidation: Oxidizing agents such as hydrogen peroxide can be used to oxidize selenophene.
Major Products:
Electrophilic Substitution Products: Various substituted selenophenes depending on the electrophile used.
Oxidation Products: Selenophene 1,1-dioxide.
科学的研究の応用
Organic Electronics
1.1 Solar Cells
Selenophene has been extensively studied for its application in organic solar cells (OSCs). The incorporation of selenophene into conjugated polymers enhances the performance of these devices. For instance, polymers like poly(3-hexylselenophene) (P3HS) have shown improved charge transport properties compared to their thiophene counterparts. Research indicates that devices based on selenophene-containing materials can achieve power conversion efficiencies (PCE) exceeding 16% .
Table 1: Performance Comparison of Selenophene-Based OSCs
Polymer | PCE (%) | Remarks |
---|---|---|
PM6:Y6Se | 16.02 | Improved molecular packing |
PM6:SePTTT-2F | 12.24 | Enhanced electron mobility |
PM6:MQ6 | 16.39 | Strong π-π stacking and good transport |
1.2 Field-Effect Transistors (FETs)
Selenophene derivatives have also been utilized in the fabrication of organic field-effect transistors (OFETs). The unique electronic characteristics of selenophene contribute to higher charge carrier mobilities, making them suitable for high-performance electronic devices. Studies show that selenophene-based polymers can achieve mobilities comparable to traditional materials like pentacene .
Materials Science
2.1 Conductive Polymers
The synthesis of selenophene-based conductive polymers has opened new avenues for material applications. These polymers exhibit lower optical band gaps compared to thiophene derivatives, which is beneficial for applications requiring efficient light absorption . The development of selenophene-linked polymers has led to materials with enhanced stability and conductivity.
2.2 Non-Fullerene Acceptors
Recent advancements include the creation of non-fullerene acceptors based on selenophene, such as IDSe, which demonstrate extended light absorption capabilities up to 800 nm. These materials exhibit balanced charge mobility and low dark current densities, making them promising candidates for next-generation organic photodetectors .
Biological Activities
3.1 Anticancer Properties
Selenophenes have been studied for their potential biological activities, particularly in cancer treatment. Certain selenophenes exhibit cytotoxic effects against various cancer cell lines, suggesting their utility as therapeutic agents . The mechanism often involves the induction of oxidative stress within cancer cells.
Table 2: Biological Activity of Selected Selenophenes
Compound | Activity Type | Target Cancer Type |
---|---|---|
Benzoselenophenes | Cytotoxicity | Breast and colon cancer |
Selenoamino acids | Antioxidant properties | Various cancers |
作用機序
Target of Action
Selenophene, a selenium-based heterocyclic compound, plays a significant role in the development of new drugs and light-emitting materials . It is an important class of organoselenium compounds that have demonstrated effectiveness against several disorders
Mode of Action
The selenophene molecule is flat and aromatic, undergoing electrophilic substitution reactions . Electrophiles tend to attack at the carbon positions next to the selenium atom . This reaction is slower than that of furan, but faster than thiophene .
Biochemical Pathways
Selenophene derivatives have been widely studied due to their intrinsic biological activity , such as antidepressant , antioxidant , anticonvulsant , antibacterial , antitumor , antinociceptive , hepatoprotective , and antiapoptotic agents .
Result of Action
Selenophene and its derivatives have demonstrated powerful biological activities . They have been widely studied due to their intrinsic biological activity , such as antidepressant , antioxidant , anticonvulsant , antibacterial , antitumor , antinociceptive , hepatoprotective , and antiapoptotic agents .
Action Environment
Selenophene substitution of photovoltaics materials can improve their intermolecular interactions and thus offer a good opportunity to finely optimize their phase separation morphology to an ideal state . This substitution strategy has shown promise in liquid crystalline donors, which have demonstrated high efficiency . The combination of these structural advantages may help to achieve state-of-the-art device performance .
生化学分析
Biochemical Properties
Selenophene is a flat molecule and, being aromatic, it undergoes electrophilic substitution reactions . As for thiophene, electrophiles tend to attack at the carbon positions next to the chalcogen . Such reactions are slower than that of furan, but faster than thiophene . The selenophene derivatives play an important role in prospecting new drugs, as well as in the development of new light-emitting materials .
Cellular Effects
In the structure of small molecules, selenophene has a force with the sulfur atom or oxygen atom next to the selenium atom in the molecule, which can reduce its band gap, improve the surface smoothness of the active layer, and increase the mutual solubility between the small molecular materials and acceptors or donors .
Molecular Mechanism
The molecular mechanism of Selenophene involves its aromatic nature and the ability to undergo electrophilic substitution reactions . Electrophiles tend to attack at the carbon positions next to the chalcogen . This property allows Selenophene to interact with other molecules in a specific manner, influencing its biochemical properties .
Temporal Effects in Laboratory Settings
It is known that Selenophene and its derivatives play an important role in the development of new light-emitting materials , suggesting that they may have stability over time.
Metabolic Pathways
It is known that organoselenium compounds, which include Selenophene, have been linked to their synthetic applications as catalysts and building blocks, allowing for several chemo-, regio- and stereoselective transformations .
Transport and Distribution
It is known that selenophene-based compounds can effectively reduce the corresponding optical band gap of materials, improve the mutual solubility of donor recipient materials, and ultimately improve the device efficiency .
Subcellular Localization
It is known that selenophene-based compounds can effectively reduce the corresponding optical band gap of materials, improve the mutual solubility of donor recipient materials, and ultimately improve the device efficiency . This suggests that Selenophene may have specific subcellular localizations that contribute to these effects.
類似化合物との比較
Selenophene is similar to other heterocyclic compounds such as:
Furan (C4H4O): Contains an oxygen atom instead of selenium.
Thiophene (C4H4S): Contains a sulfur atom instead of selenium.
Tellurophene (C4H4Te): Contains a tellurium atom instead of selenium.
Uniqueness:
- Selenophene’s unique properties arise from the presence of selenium, which imparts different reactivity and stability compared to its oxygen, sulfur, and tellurium analogs. This makes selenophene particularly valuable in applications requiring specific electronic and catalytic properties .
生物活性
Selenophene, a five-membered heterocyclic compound containing selenium, has garnered attention for its diverse biological activities and potential applications in medicinal chemistry and materials science. This article provides a comprehensive overview of the biological activities associated with selenophene, including its anticancer, antioxidant, and antimicrobial properties, along with relevant case studies and research findings.
Overview of Selenophene
Selenophene is structurally similar to thiophene, but the presence of selenium imparts unique chemical properties. This heteroatom substitution has been explored for various applications, particularly in organic electronics and as a pharmacological agent. The biological activity of selenophene derivatives has been widely studied due to their potential therapeutic benefits.
Anticancer Activity
Recent studies have highlighted the anticancer potential of selenophene-based compounds. For instance, a study synthesized several selenophene-based chalcone analogs and evaluated their efficacy against human colorectal adenocarcinoma (HT-29) cells. Among the synthesized compounds, those designated as 6, 8, and 10 exhibited significant anticancer activity with IC50 values of 19.98 ± 3.38 μM, 38.23 ± 3.30 μM, and 46.95 ± 5.68 μM, respectively .
Mechanism of Action : Compound 6 was shown to induce apoptosis through mitochondrial pathways and caspase-3 activation, suggesting a promising mechanism for cancer therapy .
Antioxidant Properties
Selenophene and its derivatives have demonstrated notable antioxidant activities. Research indicates that these compounds can scavenge free radicals and reduce oxidative stress, which is crucial in preventing cellular damage linked to various diseases . The antioxidant properties are attributed to the selenium atom's ability to participate in redox reactions.
Antimicrobial Activity
Selenophene derivatives have also been investigated for their antimicrobial effects. Various studies report that these compounds exhibit antibacterial properties against a range of pathogens, including both Gram-positive and Gram-negative bacteria . The mechanism often involves disrupting bacterial cell membranes or inhibiting essential enzymatic functions.
Other Biological Activities
In addition to the aforementioned activities, selenophene has been explored for other therapeutic potentials:
- Antidepressant Effects : Some selenophene derivatives have shown promise as antidepressants in animal models .
- Anticonvulsant Activity : Certain compounds have been tested for their ability to mitigate seizures, indicating potential use in epilepsy treatment .
- Hepatoprotective Effects : Research suggests that selenophene may protect liver cells from damage induced by toxins or oxidative stress .
Table: Summary of Biological Activities of Selenophene Derivatives
特性
IUPAC Name |
selenophene | |
---|---|---|
Source | PubChem | |
URL | https://pubchem.ncbi.nlm.nih.gov | |
Description | Data deposited in or computed by PubChem | |
InChI |
InChI=1S/C4H4Se/c1-2-4-5-3-1/h1-4H | |
Source | PubChem | |
URL | https://pubchem.ncbi.nlm.nih.gov | |
Description | Data deposited in or computed by PubChem | |
InChI Key |
MABNMNVCOAICNO-UHFFFAOYSA-N | |
Source | PubChem | |
URL | https://pubchem.ncbi.nlm.nih.gov | |
Description | Data deposited in or computed by PubChem | |
Canonical SMILES |
C1=C[Se]C=C1 | |
Source | PubChem | |
URL | https://pubchem.ncbi.nlm.nih.gov | |
Description | Data deposited in or computed by PubChem | |
Molecular Formula |
C4H4Se | |
Source | PubChem | |
URL | https://pubchem.ncbi.nlm.nih.gov | |
Description | Data deposited in or computed by PubChem | |
Related CAS |
89231-09-4 | |
Record name | Polyselenophene | |
Source | CAS Common Chemistry | |
URL | https://commonchemistry.cas.org/detail?cas_rn=89231-09-4 | |
Description | CAS Common Chemistry is an open community resource for accessing chemical information. Nearly 500,000 chemical substances from CAS REGISTRY cover areas of community interest, including common and frequently regulated chemicals, and those relevant to high school and undergraduate chemistry classes. This chemical information, curated by our expert scientists, is provided in alignment with our mission as a division of the American Chemical Society. | |
Explanation | The data from CAS Common Chemistry is provided under a CC-BY-NC 4.0 license, unless otherwise stated. | |
DSSTOX Substance ID |
DTXSID20182978 | |
Record name | Selenophene | |
Source | EPA DSSTox | |
URL | https://comptox.epa.gov/dashboard/DTXSID20182978 | |
Description | DSSTox provides a high quality public chemistry resource for supporting improved predictive toxicology. | |
Molecular Weight |
131.05 g/mol | |
Source | PubChem | |
URL | https://pubchem.ncbi.nlm.nih.gov | |
Description | Data deposited in or computed by PubChem | |
CAS No. |
288-05-1 | |
Record name | Selenophene | |
Source | CAS Common Chemistry | |
URL | https://commonchemistry.cas.org/detail?cas_rn=288-05-1 | |
Description | CAS Common Chemistry is an open community resource for accessing chemical information. Nearly 500,000 chemical substances from CAS REGISTRY cover areas of community interest, including common and frequently regulated chemicals, and those relevant to high school and undergraduate chemistry classes. This chemical information, curated by our expert scientists, is provided in alignment with our mission as a division of the American Chemical Society. | |
Explanation | The data from CAS Common Chemistry is provided under a CC-BY-NC 4.0 license, unless otherwise stated. | |
Record name | Selenophene | |
Source | ChemIDplus | |
URL | https://pubchem.ncbi.nlm.nih.gov/substance/?source=chemidplus&sourceid=0000288051 | |
Description | ChemIDplus is a free, web search system that provides access to the structure and nomenclature authority files used for the identification of chemical substances cited in National Library of Medicine (NLM) databases, including the TOXNET system. | |
Record name | Selenophene | |
Source | EPA DSSTox | |
URL | https://comptox.epa.gov/dashboard/DTXSID20182978 | |
Description | DSSTox provides a high quality public chemistry resource for supporting improved predictive toxicology. | |
Record name | selenophene | |
Source | European Chemicals Agency (ECHA) | |
URL | https://echa.europa.eu/information-on-chemicals | |
Description | The European Chemicals Agency (ECHA) is an agency of the European Union which is the driving force among regulatory authorities in implementing the EU's groundbreaking chemicals legislation for the benefit of human health and the environment as well as for innovation and competitiveness. | |
Explanation | Use of the information, documents and data from the ECHA website is subject to the terms and conditions of this Legal Notice, and subject to other binding limitations provided for under applicable law, the information, documents and data made available on the ECHA website may be reproduced, distributed and/or used, totally or in part, for non-commercial purposes provided that ECHA is acknowledged as the source: "Source: European Chemicals Agency, http://echa.europa.eu/". Such acknowledgement must be included in each copy of the material. ECHA permits and encourages organisations and individuals to create links to the ECHA website under the following cumulative conditions: Links can only be made to webpages that provide a link to the Legal Notice page. | |
Record name | SELENOPHENE | |
Source | FDA Global Substance Registration System (GSRS) | |
URL | https://gsrs.ncats.nih.gov/ginas/app/beta/substances/UYZ3M5T8L7 | |
Description | The FDA Global Substance Registration System (GSRS) enables the efficient and accurate exchange of information on what substances are in regulated products. Instead of relying on names, which vary across regulatory domains, countries, and regions, the GSRS knowledge base makes it possible for substances to be defined by standardized, scientific descriptions. | |
Explanation | Unless otherwise noted, the contents of the FDA website (www.fda.gov), both text and graphics, are not copyrighted. They are in the public domain and may be republished, reprinted and otherwise used freely by anyone without the need to obtain permission from FDA. Credit to the U.S. Food and Drug Administration as the source is appreciated but not required. | |
Retrosynthesis Analysis
AI-Powered Synthesis Planning: Our tool employs the Template_relevance Pistachio, Template_relevance Bkms_metabolic, Template_relevance Pistachio_ringbreaker, Template_relevance Reaxys, Template_relevance Reaxys_biocatalysis model, leveraging a vast database of chemical reactions to predict feasible synthetic routes.
One-Step Synthesis Focus: Specifically designed for one-step synthesis, it provides concise and direct routes for your target compounds, streamlining the synthesis process.
Accurate Predictions: Utilizing the extensive PISTACHIO, BKMS_METABOLIC, PISTACHIO_RINGBREAKER, REAXYS, REAXYS_BIOCATALYSIS database, our tool offers high-accuracy predictions, reflecting the latest in chemical research and data.
Strategy Settings
Precursor scoring | Relevance Heuristic |
---|---|
Min. plausibility | 0.01 |
Model | Template_relevance |
Template Set | Pistachio/Bkms_metabolic/Pistachio_ringbreaker/Reaxys/Reaxys_biocatalysis |
Top-N result to add to graph | 6 |
Feasible Synthetic Routes
Q1: What is selenophene?
A1: Selenophene is a five-membered aromatic heterocyclic compound containing one selenium atom. It is structurally analogous to thiophene, with selenium replacing sulfur.
Q2: What is the molecular formula and weight of selenophene?
A2: The molecular formula of selenophene is C4H4Se, and its molecular weight is 119.01 g/mol.
Q3: What spectroscopic techniques are used to characterize selenophene and its derivatives?
A3: Researchers commonly employ a range of spectroscopic techniques to characterize selenophene derivatives, including:* Nuclear Magnetic Resonance (NMR) Spectroscopy: This technique provides information about the structure and dynamics of molecules by analyzing the magnetic properties of atomic nuclei. Researchers have used 1H NMR, 13C NMR, 15N NMR, and 77Se NMR to study selenophene derivatives. [] * Raman Spectroscopy: This technique is sensitive to molecular vibrations and provides information about the chemical bonds and structure of molecules. It is used to investigate the extent of π-conjugation in polyselenophenes. []* Infrared (IR) Spectroscopy: Similar to Raman spectroscopy, IR spectroscopy provides information about molecular vibrations and functional groups present in the molecule. []* UV-Vis Spectroscopy: This technique measures the absorption and transmission of light through a substance, providing information about electronic transitions and band gaps in conjugated systems. [, , , , ]* X-ray Absorption Fine Structure (XAFS) Spectroscopy: This technique provides information about the local atomic structure and electronic state of atoms in molecules. [, ]* Electron Energy Loss Spectroscopy (EELS): This technique probes the electronic structure and bonding of materials by analyzing the energy loss of electrons passing through them. []
Q4: How does the presence of selenium impact the properties of selenophene compared to thiophene?
A4: Selenium, being a heavier atom than sulfur, significantly influences the properties of selenophene compared to thiophene. These differences include:
Q5: What applications of selenophene derivatives rely on their material compatibility and stability?
A6:
Organic Solar Cells (OSCs):* Selenophene-containing polymers have demonstrated significant potential in OSCs. Their lower band gaps and enhanced charge carrier mobilities contribute to improved light absorption and charge transport, leading to higher power conversion efficiencies. [, , , , ]
Q6: What strategies can be employed to improve the stability of selenophene-based materials?
A7: * Side-Chain Engineering: Carefully selecting and incorporating appropriate side chains can significantly impact the solubility, morphology, and stability of selenophene-based polymers. For instance, introducing bulky side chains like 2-ethylhexyl can increase solubility while retaining crystallinity. []* Copolymerization: Combining selenophene with other monomers to form copolymers allows fine-tuning of the material properties and enhancing stability. This approach is often used in OSC applications to improve morphology control, light absorption, and charge transport. [, , , , ]* Encapsulation and Interface Engineering: Encapsulating selenophene-based materials with protective layers or modifying the interfaces between the active material and electrodes can help prevent degradation pathways and improve device stability.
Q7: Does selenophene exhibit catalytic properties?
A8: While selenophene itself may not be a potent catalyst, some derivatives, like 2,5-digermaselenophene, have shown remarkable catalytic activity. [] This specific derivative can activate dihydrogen and acetylene at room temperature without the need for transition-metal catalysts.
Q8: How does the structure of 2,5-digermaselenophene contribute to its catalytic activity?
A9: Unlike typical selenophenes, 2,5-digermaselenophene adopts a trans-pyramidalized structure due to its unique electronic properties. This distorted structure, along with its considerable electron-donating and electron-accepting abilities, enables it to activate small molecules like dihydrogen and acetylene. []
試験管内研究製品の免責事項と情報
BenchChemで提示されるすべての記事および製品情報は、情報提供を目的としています。BenchChemで購入可能な製品は、生体外研究のために特別に設計されています。生体外研究は、ラテン語の "in glass" に由来し、生物体の外で行われる実験を指します。これらの製品は医薬品または薬として分類されておらず、FDAから任何の医療状態、病気、または疾患の予防、治療、または治癒のために承認されていません。これらの製品を人間または動物に体内に導入する形態は、法律により厳格に禁止されています。これらのガイドラインに従うことは、研究と実験において法的および倫理的な基準の遵守を確実にするために重要です。