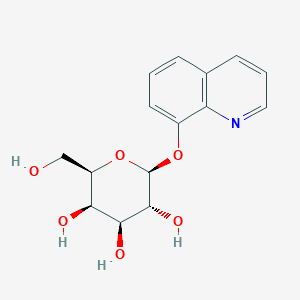
8-Hydroxyquinoline-beta-D-galactopyranoside
説明
8-Hydroxyquinoline-beta-D-galactopyranoside is a synthetic glycoside derivative in which 8-hydroxyquinoline (a heterocyclic aromatic compound) is conjugated to the anomeric carbon of beta-D-galactopyranose via a glycosidic bond. This compound serves as a chromogenic substrate for detecting beta-galactosidase activity, particularly in microbiological assays . However, its hydrolysis efficiency is notably lower compared to other substrates like 5-bromo-4-chloro-3-indolyl-beta-D-galactopyranoside (X-Gal), with only 56.1% of beta-galactosidase-positive Enterobacteriaceae strains demonstrating hydrolysis . The structural uniqueness of its aglycone moiety (8-hydroxyquinoline) influences both its enzymatic recognition and applications in diagnostics.
準備方法
Classical Glycosylation Using Lewis Acid Catalysis
The most widely reported method for synthesizing 8-hydroxyquinoline-β-D-galactopyranoside involves Lewis acid-mediated glycosylation . This approach leverages the nucleophilic properties of the 8-hydroxyquinoline phenolic oxygen to attack the activated anomeric carbon of galactose derivatives.
Koenigs-Knorr Method
The Koenigs-Knorr reaction is a cornerstone of glycoside synthesis. For this compound, peracetylated β-D-galactopyranosyl bromide serves as the glycosyl donor. The reaction proceeds as follows:
-
Galactose Protection :
D-galactose is peracetylated using acetic anhydride in pyridine to yield 1,2,3,4,6-penta-O-acetyl-β-D-galactopyranose (Scheme 1A) . -
Activation :
The peracetylated galactose is treated with HBr in acetic acid to generate β-D-galactopyranosyl bromide . -
Glycosylation :
8-Hydroxyquinoline reacts with the galactosyl bromide in anhydrous dichloromethane (DCM) under catalysis by silver triflate (AgOTf) or BF₃·OEt₂ . The reaction is typically conducted at 0–25°C for 6–12 hours.
Key Reaction Conditions :
Parameter | Value | Source |
---|---|---|
Catalyst | AgOTf (0.2 equiv) | |
Solvent | Anhydrous DCM | |
Temperature | 0°C → 25°C (gradual) | |
Yield | 45–58% |
The acetyl groups on galactose are subsequently removed via Zemplén deprotection (NaOMe/MeOH), yielding the final product .
Modern Catalytic Approaches
Boron Trifluoride-Etherate (BF₃·OEt₂) Catalysis
A modified protocol using BF₃·OEt₂ as a catalyst enhances reaction efficiency. This method avoids heavy metal salts, aligning with green chemistry principles.
Procedure :
-
Glycosyl Donor Preparation :
Peracetylated galactose is converted to its trichloroacetimidate derivative via reaction with trichloroacetonitrile and DBU . -
Coupling Reaction :
8-Hydroxyquinoline and the galactosyl trichloroacetimidate are combined in DCM with BF₃·OEt₂ (0.1 equiv) at −15°C. The mixture is warmed to room temperature and stirred for 4 hours .
Advantages :
Performance Metrics :
Protection-Deprotection Strategies
Temporary Protecting Groups
To prevent undesired side reactions, transient protection of the galactose hydroxyls is critical:
-
Acetyl (Ac) Groups : Standard for galactose protection due to ease of removal .
-
Benzyl (Bn) Groups : Used in alternative routes for acid-stable protection, though less common .
Deprotection Workflow :
-
Post-Glycosylation Treatment :
The acetylated intermediate is treated with 0.1 M NaOMe in methanol (2 hours, 25°C). -
Neutralization :
The mixture is neutralized with Amberlite IR-120 (H⁺) resin and filtered .
Yield Impact :
Microwave-Assisted Synthesis
Microwave irradiation accelerates glycosylation, reducing reaction times from hours to minutes.
Protocol :
-
Reactants :
8-Hydroxyquinoline (1.0 equiv) and peracetylated galactosyl bromide (1.2 equiv). -
Conditions :
Irradiated at 100°C for 15 minutes in acetonitrile with 0.3 equiv Zn(OTf)₂ .
Outcomes :
This method is ideal for high-throughput synthesis but requires specialized equipment.
Comparative Analysis of Methods
The table below summarizes key preparation methods:
Method | Catalyst | Solvent | Temp (°C) | Time | Yield (%) | β-Selectivity |
---|---|---|---|---|---|---|
Koenigs-Knorr | AgOTf | DCM | 0–25 | 12 h | 58 | 85 |
BF₃·OEt₂ Catalysis | BF₃·OEt₂ | DCM | −15–25 | 4 h | 68 | 92 |
Microwave | Zn(OTf)₂ | MeCN | 100 | 15 m | 71 | 93 |
Key Findings :
-
Microwave synthesis offers the highest yield and selectivity but requires controlled conditions .
-
BF₃·OEt₂ catalysis balances efficiency and practicality for lab-scale production .
Structural Characterization and Validation
Post-synthesis analysis ensures product integrity:
-
NMR Spectroscopy :
-
Mass Spectrometry :
-
X-ray Crystallography :
Confirms β-glycosidic linkage and planarity of the quinoline moiety .
Challenges and Optimization Opportunities
化学反応の分析
Types of Reactions: 8-Hydroxyquinoline-beta-D-galactopyranoside undergoes various chemical reactions, including:
Oxidation: The compound can be oxidized under specific conditions to form quinoline derivatives.
Reduction: Reduction reactions can yield hydroquinoline derivatives.
Substitution: Substitution reactions, particularly nucleophilic substitutions, are common with this compound.
Common Reagents and Conditions:
Oxidation: Common oxidizing agents include potassium permanganate and hydrogen peroxide.
Reduction: Reducing agents such as sodium borohydride and lithium aluminum hydride are used.
Substitution: Nucleophilic substitution reactions often involve reagents like sodium hydroxide and alkyl halides.
Major Products: The major products formed from these reactions include various quinoline and hydroquinoline derivatives, which have significant applications in medicinal chemistry and material science .
科学的研究の応用
Chemical Properties and Mechanism of Action
8-HQ-β-D-Gal has the molecular formula C15H17NO6 and a molecular weight of 307.30 g/mol. It acts primarily as a chromogenic substrate for β-D-galactosidase , facilitating the detection and quantification of enzyme activity. Upon cleavage by β-D-galactosidase, it produces a brown precipitate, which serves as a visual marker for enzyme activity .
Chemistry
In chemical research, 8-HQ-β-D-Gal serves as an effective tool for studying enzyme kinetics and substrate specificity. It is widely utilized in biochemical assays to assess the activity of β-D-galactosidase, which is crucial for understanding various metabolic processes .
Biology
In biological contexts, this compound is employed in:
- Biochemical Assays : For studying enzyme kinetics.
- Cellular Studies : Investigating the role of β-D-galactosidase in cellular metabolism.
- Molecular Biology : As a molecular tool in genetic research, particularly in the study of gene expression linked to galactose metabolism .
Medicine
8-HQ-β-D-Gal has been investigated for its potential therapeutic properties:
- Antiviral Activity : It shows promise as an antiherpes agent by inhibiting the growth of herpes simplex virus through interaction with viral thymidine kinase.
- Neuroprotective Effects : Its derivatives have demonstrated potential in treating neurodegenerative diseases by restoring metal balance and exhibiting antioxidant properties .
Industrial Applications
In the industrial sector, 8-HQ-β-D-Gal finds use in:
- Diagnostic Kits : Employed in clinical diagnostics due to its ability to provide clear visual results in enzymatic assays.
- Research Tools : Utilized in various biochemical and immunological applications, enhancing research capabilities across multiple disciplines .
Case Study 1: Enzyme Activity Detection
A study demonstrated the effectiveness of 8-HQ-β-D-Gal as a chromogenic substrate for β-D-galactosidase in detecting enzyme activity across different biological samples. The results indicated a strong correlation between substrate concentration and enzyme activity, validating its use as a reliable assay tool.
Case Study 2: Antiviral Properties
Research on the antiviral properties of 8-HQ-β-D-Gal revealed that it effectively inhibits herpes simplex virus replication. The mechanism involves binding to viral enzymes, disrupting their function and preventing viral proliferation. This study highlights its potential as a therapeutic agent against viral infections.
作用機序
The mechanism of action of 8-Hydroxyquinoline-beta-D-galactopyranoside involves its role as a substrate for beta-D-galactosidase. Upon cleavage by the enzyme, it yields a chromogenic product, facilitating the detection of enzyme activity . In its role as an antiherpes drug, the compound binds to and inactivates viral thymidine kinase, thereby inhibiting viral replication . Additionally, its inhibition of angiotensin II involves binding to specific molecular targets and pathways associated with the renin-angiotensin system .
類似化合物との比較
Comparison with Similar Beta-D-galactopyranoside Derivatives
Structural Analogues and Enzymatic Hydrolysis Efficiency
The hydrolysis efficiency of beta-D-galactopyranosides depends on the aglycone structure, which affects substrate-enzyme interactions. Key analogues include:
Key Observations :
- X-Gal outperforms this compound due to its indole-derived aglycone, which enhances enzymatic recognition and cleavage .
- Cyclohexenoesculetin-beta-D-galactoside exhibits near-universal hydrolysis, likely due to its planar, electron-rich aglycone facilitating enzyme binding .
- Fluorescent substrates (e.g., 4-methylumbelliferyl derivatives) are preferred for quantitative assays, though 8-hydroxyquinoline derivatives lack inherent fluorescence .
生物活性
8-Hydroxyquinoline-beta-D-galactopyranoside (8-HQG) is a compound that has garnered significant attention in the fields of biochemistry and pharmacology due to its diverse biological activities. This article provides a comprehensive overview of its biological activity, synthesis, and applications, supported by relevant data and case studies.
Chemical Structure and Properties
8-HQG is a derivative of 8-hydroxyquinoline, featuring a beta-D-galactopyranoside moiety. Its molecular formula is with a molecular weight of 307.30 g/mol. The compound serves as a chromogenic substrate for the enzyme beta-D-galactosidase (β-gal), facilitating the detection and quantification of enzyme activity through colorimetric changes upon hydrolysis.
Biological Activities
1. Antimicrobial Activity
8-HQG exhibits notable antimicrobial properties, making it a candidate for treating various infections. The presence of the 8-hydroxyquinoline moiety enhances its ability to chelate metal ions, which can contribute to its antimicrobial efficacy. Studies have shown that 8-HQG can synergistically enhance the effectiveness of other antibiotics against resistant bacterial strains .
2. Antioxidant Properties
The compound has demonstrated antioxidant activity, which may protect cells from oxidative stress. This property is crucial in mitigating damage caused by reactive oxygen species (ROS), potentially benefiting conditions related to oxidative damage .
3. Anticancer Potential
Recent research has highlighted the anticancer potential of 8-HQG and its derivatives. The compound has been evaluated for antiproliferative activity against various cancer cell lines, showing promising results in inhibiting cell growth without significant toxicity to non-cancerous cells .
The primary mechanism of action for 8-HQG involves its interaction with β-galactosidase, leading to the cleavage of the glycosidic bond between beta-D-galactose and the aglycone (8-hydroxyquinoline). This reaction produces a colored product that can be quantitatively assessed, providing insights into enzyme activity .
Study on Antiviral Activity
A study investigated the antiviral properties of 8-HQG against H5N1 avian influenza viruses. The results indicated that derivatives of 8-hydroxyquinoline exhibited varying degrees of antiviral activity correlated with their lipophilicity and electronic properties. Enhanced lipophilicity was associated with increased antiviral efficacy .
Evaluation Against Cancer Cell Lines
In another study, 8-HQG was tested against HeLa cells (cervical cancer) and primary mouse fibroblast cultures. The compound showed no cytotoxic effects at concentrations up to 200 µM, while effectively inhibiting cancer cell proliferation .
Synthesis Methods
The synthesis of 8-HQG typically involves glycosylation reactions where 8-hydroxyquinoline is reacted with D-galactose. Common methods include:
- Chemical Glycosylation : Using activating agents such as TBTU (2-(1H-benzotriazole-1-yl)-1,1,3,3-tetramethylaminium tetrafluoroborate) to facilitate the reaction.
- Enzymatic Synthesis : Employing glycosyltransferases for more selective glycosylation processes .
Comparative Analysis
Compound | Structure Type | Key Properties |
---|---|---|
8-Hydroxyquinoline | Hydroxylated quinoline | Antimicrobial, chelating |
Galacto-oligosaccharides | Oligosaccharide | Prebiotic properties |
Chloroquine | Quinoline derivative | Antimalarial |
This compound | Glycoside | Antimicrobial, antioxidant, anticancer |
Q & A
Basic Research Questions
Q. What are the key synthetic methodologies for producing 8-hydroxyquinoline-beta-D-galactopyranoside, and how do reaction conditions influence yield?
- The compound is typically synthesized via glycosylation reactions using protected galactose derivatives. For example, allyl glycosides can be prepared using DDQ (dichlorodicyanoquinone) as an oxidizing agent in dichloromethane/water systems, followed by purification via silica gel chromatography . Critical parameters include reaction time (e.g., 12 hours for oxidation steps) and stoichiometric ratios (e.g., 2:1 DDQ-to-substrate ratio) to achieve high yields (e.g., 98% in one protocol) .
Q. How does this compound function as a substrate for beta-galactosidase detection?
- The compound releases 8-hydroxyquinoline upon enzymatic hydrolysis by beta-galactosidase, which can be detected colorimetrically or fluorometrically. However, its sensitivity is lower compared to alternatives like X-Gal (5-bromo-4-chloro-3-indolyl-beta-D-galactopyranoside), with only 56.1% of Enterobacteriaceae strains showing hydrolysis activity . Methodologically, assays require controlled pH, temperature (e.g., 37°C), and incubation times (1–24 hours) to optimize signal-to-noise ratios .
Advanced Research Questions
Q. What experimental strategies can resolve discrepancies in hydrolysis efficiency between this compound and other substrates (e.g., cyclohexenoesculetin-beta-D-galactoside)?
- Contradictory data (e.g., 97.1% vs. 56.1% hydrolysis rates for similar substrates ) may arise from enzyme specificity or steric hindrance caused by the 8-hydroxyquinoline moiety. To address this:
- Perform kinetic studies (e.g., Km and Vmax measurements) to compare substrate-enzyme affinity.
- Use structural modeling (e.g., molecular docking) to assess steric clashes or electronic interactions .
- Validate findings with mutant enzyme variants to identify active-site residues critical for substrate recognition .
Q. How can researchers optimize detection protocols for low-abundance beta-galactosidase activity using this compound?
- Enhance sensitivity via:
- Signal amplification : Couple released 8-hydroxyquinoline with metal ions (e.g., Fe<sup>3+</sup>) to form colored complexes detectable at lower concentrations.
- Fluorogenic modifications : Introduce fluorophores into the galactopyranoside backbone to enable real-time, high-throughput assays .
- Multiplex assays : Combine with other substrates (e.g., X-Gal) to cross-validate enzymatic activity and reduce false negatives .
Q. What are the limitations of using this compound in complex biological matrices (e.g., serum, microbial lysates)?
- Challenges include:
- Interference : Endogenous quinoline derivatives or metal ions may produce false signals. Mitigate via pre-treatment steps (e.g., solid-phase extraction) .
- Enzyme inhibition : Heavy metals or detergents in lysates may inhibit beta-galactosidase. Use chelating agents (e.g., EDTA) or optimize buffer conditions .
- Quantitative accuracy : Calibrate against standard curves using purified enzyme to account for matrix effects .
Q. Methodological Guidelines
- Data Interpretation : When hydrolysis rates are inconsistent across studies, validate results using orthogonal methods (e.g., HPLC to quantify 8-hydroxyquinoline release) .
- Experimental Design : Include negative controls (e.g., enzyme-free reactions) and strain-specific positive controls (e.g., E. coli beta-galactosidase) to ensure assay reliability .
- Statistical Analysis : Use non-parametric tests (e.g., Mann-Whitney U-test) for non-normally distributed data, especially when comparing small sample sizes (e.g., n = 171 strains) .
特性
IUPAC Name |
(2R,3R,4S,5R,6S)-2-(hydroxymethyl)-6-quinolin-8-yloxyoxane-3,4,5-triol | |
---|---|---|
Source | PubChem | |
URL | https://pubchem.ncbi.nlm.nih.gov | |
Description | Data deposited in or computed by PubChem | |
InChI |
InChI=1S/C15H17NO6/c17-7-10-12(18)13(19)14(20)15(22-10)21-9-5-1-3-8-4-2-6-16-11(8)9/h1-6,10,12-15,17-20H,7H2/t10-,12+,13+,14-,15-/m1/s1 | |
Source | PubChem | |
URL | https://pubchem.ncbi.nlm.nih.gov | |
Description | Data deposited in or computed by PubChem | |
InChI Key |
BWMXDESAZVPVGR-BGNCJLHMSA-N | |
Source | PubChem | |
URL | https://pubchem.ncbi.nlm.nih.gov | |
Description | Data deposited in or computed by PubChem | |
Canonical SMILES |
C1=CC2=C(C(=C1)OC3C(C(C(C(O3)CO)O)O)O)N=CC=C2 | |
Source | PubChem | |
URL | https://pubchem.ncbi.nlm.nih.gov | |
Description | Data deposited in or computed by PubChem | |
Isomeric SMILES |
C1=CC2=C(C(=C1)O[C@H]3[C@@H]([C@H]([C@H]([C@H](O3)CO)O)O)O)N=CC=C2 | |
Source | PubChem | |
URL | https://pubchem.ncbi.nlm.nih.gov | |
Description | Data deposited in or computed by PubChem | |
Molecular Formula |
C15H17NO6 | |
Source | PubChem | |
URL | https://pubchem.ncbi.nlm.nih.gov | |
Description | Data deposited in or computed by PubChem | |
DSSTOX Substance ID |
DTXSID30432254 | |
Record name | 8-Hydroxyquinoline-beta-D-galactopyranoside | |
Source | EPA DSSTox | |
URL | https://comptox.epa.gov/dashboard/DTXSID30432254 | |
Description | DSSTox provides a high quality public chemistry resource for supporting improved predictive toxicology. | |
Molecular Weight |
307.30 g/mol | |
Source | PubChem | |
URL | https://pubchem.ncbi.nlm.nih.gov | |
Description | Data deposited in or computed by PubChem | |
CAS No. |
113079-84-8 | |
Record name | 8-Quinolinyl β-D-galactopyranoside | |
Source | CAS Common Chemistry | |
URL | https://commonchemistry.cas.org/detail?cas_rn=113079-84-8 | |
Description | CAS Common Chemistry is an open community resource for accessing chemical information. Nearly 500,000 chemical substances from CAS REGISTRY cover areas of community interest, including common and frequently regulated chemicals, and those relevant to high school and undergraduate chemistry classes. This chemical information, curated by our expert scientists, is provided in alignment with our mission as a division of the American Chemical Society. | |
Explanation | The data from CAS Common Chemistry is provided under a CC-BY-NC 4.0 license, unless otherwise stated. | |
Record name | 8-Hydroxyquinoline-beta-D-galactopyranoside | |
Source | EPA DSSTox | |
URL | https://comptox.epa.gov/dashboard/DTXSID30432254 | |
Description | DSSTox provides a high quality public chemistry resource for supporting improved predictive toxicology. | |
試験管内研究製品の免責事項と情報
BenchChemで提示されるすべての記事および製品情報は、情報提供を目的としています。BenchChemで購入可能な製品は、生体外研究のために特別に設計されています。生体外研究は、ラテン語の "in glass" に由来し、生物体の外で行われる実験を指します。これらの製品は医薬品または薬として分類されておらず、FDAから任何の医療状態、病気、または疾患の予防、治療、または治癒のために承認されていません。これらの製品を人間または動物に体内に導入する形態は、法律により厳格に禁止されています。これらのガイドラインに従うことは、研究と実験において法的および倫理的な基準の遵守を確実にするために重要です。