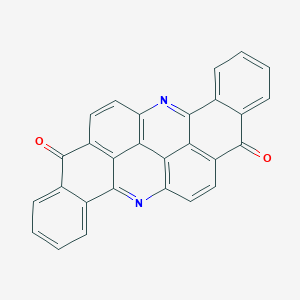
Flavanthrone
概要
説明
Flavanthrone (CAS 475-71-8), also known as Pigment Yellow 24 or Vat Yellow 1, is an anthraquinoid vat dye with the molecular formula C₂₈H₁₂N₂O₂ and a molecular weight of 408.41 g/mol . Structurally, it consists of a fused polycyclic aromatic system with two carbonyl groups, classified as a periazaacene derivative . This compound is synthesized via the chlorination and condensation of anthraquinone derivatives in aromatic solvents like nitrobenzene, followed by alkaline cyclization .
準備方法
Historical and Traditional Synthesis Pathways
Antimony Pentachloride-Mediated Cyclization
The classical synthesis of flavanthrone involves the treatment of 2-aminoanthraquinone with antimony pentachloride (SbCl₅) in nitrobenzene. This reaction facilitates intramolecular cyclization, forming the this compound core structure. Critical to this process is the electron-deficient nature of SbCl₅, which acts as a Lewis acid to promote dehydration and ring closure . The crude product obtained is typically contaminated with residual nitrobenzene and antimony byproducts, necessitating extensive purification via recrystallization or solvent extraction.
Ullmann Reaction-Based Approaches
An alternative route employs the Ullmann reaction, wherein acylated 1-chloro-2-aminoanthraquinone undergoes coupling in the presence of a copper catalyst. This method proceeds via a nucleophilic aromatic substitution mechanism, followed by alkaline hydrolysis to remove acetyl protecting groups . While this pathway offers improved regioselectivity compared to SbCl₅-mediated cyclization, it requires stringent temperature control (120–140°C) and prolonged reaction times (>24 hours), limiting industrial scalability.
Acid Pasting for Particle Size Reduction
Post-synthesis conditioning of crude this compound traditionally involves "acid pasting," a process where the pigment is treated with concentrated sulfuric acid (85–98% assay) and subsequently drowned in ice-water. This step achieves dual objectives: (1) particle size reduction to the submicron scale, enhancing pigment dispersion, and (2) removal of residual organic solvents . The resultant material exhibits a characteristic X-ray diffraction pattern with prominent peaks at 7.34, 7.00, 3.72, 3.67, 3.52, 3.39, and 3.31 dÅ, indicative of the α-polymorph .
Novel Polymorphic Form Synthesis via Nitric Acid and Sulfonate Modifications
Nitric Acid Conditioning for β-Polymorph Formation
A breakthrough in this compound technology emerged with the discovery that treatment with nitric acid (specific gravity 1.49–1.50) induces a polymorphic transition to the β-form. As detailed in Example 1 of the foundational patent , this method involves:
-
Gradual addition of crude this compound (50 parts) to 300 parts nitric acid at 25°C
-
Agitation for 1 hour to ensure homogeneous mixing
-
Quenching in an ice-water mixture (0–10°C) to precipitate the pigment
-
Sequential heating to 95–100°C for 2 hours, followed by oxidative treatment with sodium hypochlorite
The β-polymorph exhibits distinct X-ray characteristics, notably a single prominent peak between 7.00–8.00 dÅ and only two peaks in the 3.30–3.75 dÅ range, contrasting sharply with the α-form's five peaks in this region .
Table 1: X-Ray Diffraction Peak Comparison Between α- and β-Flavanthrone
Polymorph | 7.00–8.00 dÅ Peaks | 3.30–3.75 dÅ Peaks | Prominent Peaks (Intensity ≥20) |
---|---|---|---|
α-Form | 2 | 5 | 7.34, 7.00, 3.72, 3.67, 3.52 |
β-Form | 1 | 2 | 7.00, 3.72, 3.39 |
Sulfuric Acid-Aromatic Sulfonate Hybrid Process
The synergistic use of sulfuric acid and aromatic sulfonates (e.g., sodium xylene sulfonate) represents another advancement in polymorph control. As demonstrated in Example 2 :
-
35 parts crude this compound are treated with 350 parts H₂SO₄ (85%) and 70 parts sodium xylene sulfonate
-
Reaction at 65–70°C for 2.5 hours induces sulfonic acid group complexation
-
Subsequent drowning and oxidative bleaching yield a material with 37% increased transparency compared to traditional forms
This method leverages the sulfonate's surfactant-like properties to direct crystal growth, achieving particle sizes below 200 nm without mechanical milling .
Comparative Analysis of Synthesis Methodologies
Chromatic Performance Differences
The β-polymorph produced via nitric acid conditioning exhibits a reddish-yellow shade (CIE Lab*: 85.2, 12.3, 89.4) versus the greener-yellow α-form (82.7, -4.5, 83.1) . This color shift arises from altered π-π stacking distances in the crystalline lattice, modifying the electronic transition energies responsible for light absorption.
Table 2: Key Process Parameters Across Methods
Parameter | SbCl₅ Method | Ullmann Route | HNO₃ Conditioning | H₂SO₄-Sulfonate |
---|---|---|---|---|
Reaction Time (h) | 6–8 | 24–36 | 3–5 | 4–6 |
Yield (%) | 68–72 | 75–80 | 89–92 | 84–88 |
Particle Size (nm) | 300–500 | 200–400 | 80–120 | 70–100 |
Modern Advancements in Reduction Chemistry
Recent studies demonstrate this compound's capacity to form charge-transfer complexes through controlled reduction. Work by Konarev et al. reveals that sodium fluorenone ketyl in dichlorobenzene reduces this compound to a monoanionic radical state ({Crypt(Na⁺)}(this compound⁻)·2C₆H₄Cl₂), while stronger reductants like vanadocene yield dianionic species. Though primarily explored for electronic applications, these findings suggest potential for tailoring this compound's redox properties during synthesis.
Industrial Scale-Up Challenges and Solutions
Temperature Control in Polymorphic Transitions
Maintaining reaction temperatures within ±2°C during nitric acid treatment is critical for polymorph purity. Industrial reactors now employ jacketed vessels with PID-controlled cooling to achieve this precision.
Oxidation State Management
Post-drowning oxidation with sodium hypochlorite (0.5–1.5% active chlorine) must be carefully titrated to avoid over-oxidation, which degrades chromophore intensity. In-line UV-Vis monitoring at 450 nm enables real-time adjustment of bleach concentrations .
化学反応の分析
Types of Reactions: Flavanthrone undergoes several types of chemical reactions, including reduction, oxidation, and complex formation. The reduction of this compound is accompanied by the elongation of the carbonyl C=O bonds and shortening of the C-C(O) bonds .
Common Reagents and Conditions:
Complex Formation: Vanadocene is used to form zwitterionic complexes with this compound.
Major Products:
Radical Anion: this compound˙−
Dianion: Flavanthrone2−
Zwitterionic Complex: {(Cp2V+)2(Flavanthrone2−)}·2C6H4Cl2
科学的研究の応用
Organic Electronics
Electroluminescent Materials
Flavanthrone and its derivatives have been extensively studied for their potential use in organic light-emitting diodes (OLEDs). These compounds demonstrate efficient electroluminescence, making them suitable as guest molecules in host-guest systems. For instance, research has shown that soluble this compound derivatives can be incorporated into a 4,4′-bis(N-carbazolyl)-1,1′-biphenyl host matrix, enhancing the performance of OLEDs significantly .
Case Study: Synthesis and Performance
A systematic study focused on synthesizing new soluble this compound derivatives revealed their high photoluminescence quantum yields (PLQY), reaching up to 80% . These materials exhibit distinct electrochemical properties and can self-assemble into ordered structures, which are crucial for device performance. The following table summarizes key properties of selected this compound derivatives used in OLED applications:
Derivative | PLQY (%) | Emission Wavelength (nm) | Host Material |
---|---|---|---|
FC-1 | 80 | 554 | 4,4′-bis(N-carbazolyl)-1,1′-biphenyl |
FC-2 | 75 | 570 | 4,4′-bis(N-carbazolyl)-1,1′-biphenyl |
FC-3 | 78 | 560 | 4,4′-bis(N-carbazolyl)-1,1′-biphenyl |
Photonic Applications
Optical Properties
This compound exhibits notable optical properties that make it suitable for various photonic applications. Its ability to form zwitterionic complexes upon reduction allows for the exploration of its magnetic interactions with other compounds. For example, complexes formed with vanadocene have been studied to understand the role of π-conjugated molecules in magnetic interactions .
Case Study: Magnetic Interaction Studies
The reduction of this compound using vanadocene leads to the formation of a dianion state that can be analyzed for its magnetic properties. This study reveals how this compound can be utilized in advanced materials for spintronic applications.
Medicinal Chemistry
Anticancer and Antimicrobial Activities
Research indicates that this compound derivatives possess promising anticancer and antimicrobial properties. The compound has been evaluated against various cancer cell lines and has shown significant cytotoxic effects.
Case Study: Anticancer Activity Assessment
In vitro studies demonstrated that specific this compound derivatives exhibit IC50 values in the low micromolar range against cancer cell lines such as MCF-7 (breast cancer) and HeLa (cervical cancer) cells. The following table presents the anticancer activity profile of selected this compound derivatives:
Derivative | Cell Line | IC50 (µM) | Mechanism of Action |
---|---|---|---|
FC-A | MCF-7 | 5.2 | Induction of apoptosis |
FC-B | HeLa | 6.8 | Inhibition of cell proliferation |
FC-C | A549 (lung) | 4.5 | Cell cycle arrest |
作用機序
The mechanism of action of flavanthrone involves its ability to undergo redox transitions. The reduction of this compound is accompanied by significant changes in its molecular structure, including the elongation of carbonyl C=O bonds and the shortening of C-C(O) bonds . These structural changes are crucial for its function as a ligand and its ability to form complexes with other molecules.
類似化合物との比較
Key Properties :
- Physical State : Yellow to brownish-yellow crystalline powder .
- Thermal Stability : Heat resistance up to 180°C .
- Lightfastness : Rated 7 (excellent resistance to fading under light exposure) .
- Solubility : Insoluble in water but soluble in concentrated sulfuric acid and alkaline solutions .
- Applications : Primarily used in textile dyeing, with emerging applications in organic electronics due to its electroactive properties .
Flavanthrone vs. Indanthrone (C.I. Vat Blue 4)
Structural Differences :
- This compound: Features a benzo[h]benz[5,6]acridino[2,1,9,8-klmna]acridine-8,16-dione backbone with two carbonyl groups .
- Indanthrone : Contains a dibenzanthrone structure with additional fused benzene rings, resulting in a blue color compared to this compound’s yellow hue .
Derivatives :
- This compound derivatives modified via carbonyl reduction and O-alkylation exhibit enhanced solubility for solution-processed organic electronics .
- Indanthrone derivatives, such as 3,3′-dimethyl variants, show improved thermal stability but reduced color intensity compared to the parent compound .
This compound vs. Fluoranthene-Based Dyes
Structural Contrast :
- This compound: Anthraquinoid structure with nitrogen and oxygen heteroatoms .
- Fluoranthene Derivatives : Polycyclic aromatic hydrocarbons (PAHs) without heteroatoms (e.g., fluoranthene dihydrodiols in ) .
Functional Differences :
Research Findings and Innovations
- Coordination Chemistry : this compound forms stable coordination complexes with transition metals (e.g., Mn²⁺, Cr³⁺) and lanthanides (e.g., Dy³⁺), enabling applications in magnetic and optical materials .
- Sustainability Challenges : Both this compound and Indanthrone face scalability issues due to toxic solvents (e.g., nitrobenzene) and energy-intensive synthesis .
生物活性
Flavanthrone, a member of the flavonoid family, has garnered attention for its diverse biological activities. This article delves into the mechanisms, effects, and research findings related to the biological activity of this compound, supported by data tables and relevant case studies.
Overview of this compound
This compound is characterized by its unique chemical structure, which includes a chromone backbone. It is primarily recognized for its antioxidant properties and potential therapeutic applications in various diseases, including cancer and cardiovascular disorders.
Antioxidant Activity
This compound exhibits significant antioxidant activity , which is a hallmark of many flavonoids. This activity is attributed to its ability to scavenge reactive oxygen species (ROS) and chelate metal ions, thus preventing oxidative stress-related damage. The antioxidant capacity of flavonoids like this compound is influenced by:
- Hydroxyl Group Arrangement : The configuration and number of hydroxyl groups on the flavonoid structure play a crucial role in its radical scavenging ability.
- Mechanisms of Action :
Biological Activities
This compound has been studied for various biological activities beyond its antioxidant properties:
- Anticancer Activity : Research indicates that this compound can induce apoptosis in cancer cells. For instance, studies have shown that certain derivatives of this compound exhibit potent inhibitory effects against lung cancer cell lines (A549), with IC50 values significantly lower than standard chemotherapeutic agents like 5-fluorouracil .
- Anti-inflammatory Effects : this compound has demonstrated the ability to reduce pro-inflammatory cytokines, contributing to its potential in managing inflammatory diseases .
- Antimicrobial Properties : Some studies suggest that this compound possesses antimicrobial activity against various pathogens, making it a candidate for developing new antimicrobial agents .
Case Studies and Research Findings
- Anticancer Efficacy :
- Inflammation Reduction :
- Antimicrobial Activity :
Data Summary
The following table summarizes key biological activities associated with this compound:
Q & A
Basic Research Questions
Q. What established synthetic methodologies are used to produce flavanthrone, and how do reaction parameters (e.g., solvent, temperature) influence yield and purity?
- Methodological Answer : this compound synthesis typically involves condensation reactions under controlled conditions. Key parameters include solvent polarity (e.g., dimethylformamide for high-temperature stability), catalyst selection (e.g., Lewis acids for regioselectivity), and inert atmospheres to prevent oxidation. Post-synthesis purification via column chromatography or recrystallization ensures purity. Yield optimization requires iterative adjustments to stoichiometry and reaction time. Analytical validation using HPLC (≥95% purity thresholds) and NMR (to confirm structural integrity) is critical .
Q. What spectroscopic and chromatographic techniques are recommended for characterizing this compound’s structural and optical properties?
- Methodological Answer : UV-Vis spectroscopy (λmax in visible range for quinoid structures) and FT-IR (C=O and C-H aromatic stretching) provide preliminary characterization. High-resolution mass spectrometry (HRMS) confirms molecular weight, while XRD resolves crystalline phase purity. For optical properties, fluorescence spectroscopy quantifies quantum yield, and HPLC-PDA (photodiode array detection) monitors degradation byproducts. Cross-referencing with literature databases (e.g., SciFinder) ensures data consistency .
Q. How does pH affect this compound’s stability in aqueous solutions, and what protocols ensure reproducibility in degradation studies?
- Methodological Answer : Conduct accelerated stability testing under varying pH (2–12) using buffered solutions. Monitor degradation kinetics via UV-Vis absorbance shifts and LC-MS to identify breakdown products. Control temperature (±0.5°C) and light exposure to isolate pH effects. Statistical validation (e.g., ANOVA for replicate measurements) minimizes experimental error .
Advanced Research Questions
Q. What mechanistic insights can computational modeling (e.g., DFT) provide into this compound’s electronic structure and redox behavior?
- Methodological Answer : Density Functional Theory (DFT) calculations predict frontier molecular orbitals (HOMO/LUMO) to explain charge-transfer properties. Solvation models (e.g., COSMO) simulate solvent effects on redox potentials. Validate predictions with cyclic voltammetry (scan rates 10–100 mV/s) and compare experimental vs. computed band gaps. Use software suites like Gaussian or ORCA with benchmarked functionals (e.g., B3LYP/6-311+G(d,p)) .
Q. How can researchers reconcile contradictory reports on this compound’s catalytic activity in organic transformations?
- Methodological Answer : Perform controlled replicate studies under standardized conditions (e.g., solvent, substrate ratio). Use Arrhenius plots to assess temperature-dependent activity variations. Cross-analyze with XPS (X-ray photoelectron spectroscopy) to detect surface oxidation states influencing catalytic efficiency. Meta-analysis of literature data should account for instrument sensitivity (e.g., GC-MS detection limits) and reaction scalability .
Q. What experimental designs are optimal for studying this compound’s interactions with biomolecules (e.g., proteins or DNA)?
- Methodological Answer : Employ fluorescence quenching assays to quantify binding constants (e.g., Stern-Volmer plots). Use circular dichroism (CD) to monitor conformational changes in biomolecules. Molecular docking simulations (AutoDock Vina) predict binding sites, validated by mutagenesis studies. Include negative controls (e.g., denatured proteins) and triplicate measurements to ensure statistical robustness .
Q. Data Analysis & Interpretation
Q. How should researchers address discrepancies in reported XRD patterns for this compound polymorphs?
- Methodological Answer : Compare experimental XRD data with Cambridge Structural Database entries. Use Rietveld refinement to account for crystallographic variations. Control for sample preparation artifacts (e.g., solvent evaporation rates). Collaborative cross-lab validation reduces instrumentation bias .
Q. What statistical approaches are recommended for analyzing dose-response relationships in this compound toxicity studies?
- Methodological Answer : Apply nonlinear regression models (e.g., Hill equation) to EC50 calculations. Use Kolmogorov-Smirnov tests to assess data normality and Mann-Whitney U tests for nonparametric comparisons. Report confidence intervals (95%) and p-values adjusted for multiple comparisons (e.g., Bonferroni correction) .
Q. Experimental Reproducibility
Q. What steps ensure reproducibility in this compound’s electrochemical synthesis across laboratories?
- Methodological Answer : Standardize electrode materials (e.g., Pt vs. carbon), electrolyte composition, and potentiostat calibration. Document ohmic drop corrections and reference electrode potentials. Share raw cyclic voltammograms in open-access repositories (e.g., Zenodo) for cross-validation .
Q. How can researchers mitigate batch-to-batch variability in this compound nanoparticle fabrication?
- Methodological Answer : Implement strict colloidal synthesis protocols (e.g., controlled nucleation via hot-injection). Characterize batches using DLS (dynamic light scattering) for size distribution and TEM for morphology. Use Design of Experiments (DoE) to optimize surfactant ratios and reaction times .
特性
IUPAC Name |
16,30-diazaoctacyclo[15.11.1.14,28.02,15.03,12.05,10.018,23.025,29]triaconta-1(28),2(15),3(12),4(30),5,7,9,13,16,18,20,22,25(29),26-tetradecaene-11,24-dione | |
---|---|---|
Source | PubChem | |
URL | https://pubchem.ncbi.nlm.nih.gov | |
Description | Data deposited in or computed by PubChem | |
InChI |
InChI=1S/C28H12N2O2/c31-27-15-7-3-1-5-13(15)25-21-17(27)9-12-20-23(21)24-19(29-25)11-10-18-22(24)26(30-20)14-6-2-4-8-16(14)28(18)32/h1-12H | |
Source | PubChem | |
URL | https://pubchem.ncbi.nlm.nih.gov | |
Description | Data deposited in or computed by PubChem | |
InChI Key |
KJPJZBYFYBYKPK-UHFFFAOYSA-N | |
Source | PubChem | |
URL | https://pubchem.ncbi.nlm.nih.gov | |
Description | Data deposited in or computed by PubChem | |
Canonical SMILES |
C1=CC=C2C(=C1)C3=NC4=C5C6=C(C=C4)C(=O)C7=CC=CC=C7C6=NC8=C5C3=C(C2=O)C=C8 | |
Source | PubChem | |
URL | https://pubchem.ncbi.nlm.nih.gov | |
Description | Data deposited in or computed by PubChem | |
Molecular Formula |
C28H12N2O2 | |
Source | PubChem | |
URL | https://pubchem.ncbi.nlm.nih.gov | |
Description | Data deposited in or computed by PubChem | |
DSSTOX Substance ID |
DTXSID6060056 | |
Record name | Benzo[h]benz[5,6]acridino[2,1,9,8-klmna]acridine-8,16-dione | |
Source | EPA DSSTox | |
URL | https://comptox.epa.gov/dashboard/DTXSID6060056 | |
Description | DSSTox provides a high quality public chemistry resource for supporting improved predictive toxicology. | |
Molecular Weight |
408.4 g/mol | |
Source | PubChem | |
URL | https://pubchem.ncbi.nlm.nih.gov | |
Description | Data deposited in or computed by PubChem | |
CAS No. |
475-71-8 | |
Record name | Flavanthrone | |
Source | CAS Common Chemistry | |
URL | https://commonchemistry.cas.org/detail?cas_rn=475-71-8 | |
Description | CAS Common Chemistry is an open community resource for accessing chemical information. Nearly 500,000 chemical substances from CAS REGISTRY cover areas of community interest, including common and frequently regulated chemicals, and those relevant to high school and undergraduate chemistry classes. This chemical information, curated by our expert scientists, is provided in alignment with our mission as a division of the American Chemical Society. | |
Explanation | The data from CAS Common Chemistry is provided under a CC-BY-NC 4.0 license, unless otherwise stated. | |
Record name | C. I. Vat Yellow 1 | |
Source | ChemIDplus | |
URL | https://pubchem.ncbi.nlm.nih.gov/substance/?source=chemidplus&sourceid=0000475718 | |
Description | ChemIDplus is a free, web search system that provides access to the structure and nomenclature authority files used for the identification of chemical substances cited in National Library of Medicine (NLM) databases, including the TOXNET system. | |
Record name | FLAVANTHRONE | |
Source | DTP/NCI | |
URL | https://dtp.cancer.gov/dtpstandard/servlet/dwindex?searchtype=NSC&outputformat=html&searchlist=39910 | |
Description | The NCI Development Therapeutics Program (DTP) provides services and resources to the academic and private-sector research communities worldwide to facilitate the discovery and development of new cancer therapeutic agents. | |
Explanation | Unless otherwise indicated, all text within NCI products is free of copyright and may be reused without our permission. Credit the National Cancer Institute as the source. | |
Record name | FLAVANTHRONE | |
Source | DTP/NCI | |
URL | https://dtp.cancer.gov/dtpstandard/servlet/dwindex?searchtype=NSC&outputformat=html&searchlist=16091 | |
Description | The NCI Development Therapeutics Program (DTP) provides services and resources to the academic and private-sector research communities worldwide to facilitate the discovery and development of new cancer therapeutic agents. | |
Explanation | Unless otherwise indicated, all text within NCI products is free of copyright and may be reused without our permission. Credit the National Cancer Institute as the source. | |
Record name | Benzo[h]benz[5,6]acridino[2,1,9,8-klmna]acridine-8,16-dione | |
Source | EPA Chemicals under the TSCA | |
URL | https://www.epa.gov/chemicals-under-tsca | |
Description | EPA Chemicals under the Toxic Substances Control Act (TSCA) collection contains information on chemicals and their regulations under TSCA, including non-confidential content from the TSCA Chemical Substance Inventory and Chemical Data Reporting. | |
Record name | Benzo[h]benz[5,6]acridino[2,1,9,8-klmna]acridine-8,16-dione | |
Source | EPA DSSTox | |
URL | https://comptox.epa.gov/dashboard/DTXSID6060056 | |
Description | DSSTox provides a high quality public chemistry resource for supporting improved predictive toxicology. | |
Record name | Benzo[h]benz[5,6]acridino[2,1,9,8-klmna]acridine-8,16-dione | |
Source | European Chemicals Agency (ECHA) | |
URL | https://echa.europa.eu/substance-information/-/substanceinfo/100.006.818 | |
Description | The European Chemicals Agency (ECHA) is an agency of the European Union which is the driving force among regulatory authorities in implementing the EU's groundbreaking chemicals legislation for the benefit of human health and the environment as well as for innovation and competitiveness. | |
Explanation | Use of the information, documents and data from the ECHA website is subject to the terms and conditions of this Legal Notice, and subject to other binding limitations provided for under applicable law, the information, documents and data made available on the ECHA website may be reproduced, distributed and/or used, totally or in part, for non-commercial purposes provided that ECHA is acknowledged as the source: "Source: European Chemicals Agency, http://echa.europa.eu/". Such acknowledgement must be included in each copy of the material. ECHA permits and encourages organisations and individuals to create links to the ECHA website under the following cumulative conditions: Links can only be made to webpages that provide a link to the Legal Notice page. | |
Record name | FLAVANTHRONE | |
Source | FDA Global Substance Registration System (GSRS) | |
URL | https://gsrs.ncats.nih.gov/ginas/app/beta/substances/5FA52JAB7C | |
Description | The FDA Global Substance Registration System (GSRS) enables the efficient and accurate exchange of information on what substances are in regulated products. Instead of relying on names, which vary across regulatory domains, countries, and regions, the GSRS knowledge base makes it possible for substances to be defined by standardized, scientific descriptions. | |
Explanation | Unless otherwise noted, the contents of the FDA website (www.fda.gov), both text and graphics, are not copyrighted. They are in the public domain and may be republished, reprinted and otherwise used freely by anyone without the need to obtain permission from FDA. Credit to the U.S. Food and Drug Administration as the source is appreciated but not required. | |
Retrosynthesis Analysis
AI-Powered Synthesis Planning: Our tool employs the Template_relevance Pistachio, Template_relevance Bkms_metabolic, Template_relevance Pistachio_ringbreaker, Template_relevance Reaxys, Template_relevance Reaxys_biocatalysis model, leveraging a vast database of chemical reactions to predict feasible synthetic routes.
One-Step Synthesis Focus: Specifically designed for one-step synthesis, it provides concise and direct routes for your target compounds, streamlining the synthesis process.
Accurate Predictions: Utilizing the extensive PISTACHIO, BKMS_METABOLIC, PISTACHIO_RINGBREAKER, REAXYS, REAXYS_BIOCATALYSIS database, our tool offers high-accuracy predictions, reflecting the latest in chemical research and data.
Strategy Settings
Precursor scoring | Relevance Heuristic |
---|---|
Min. plausibility | 0.01 |
Model | Template_relevance |
Template Set | Pistachio/Bkms_metabolic/Pistachio_ringbreaker/Reaxys/Reaxys_biocatalysis |
Top-N result to add to graph | 6 |
Feasible Synthetic Routes
試験管内研究製品の免責事項と情報
BenchChemで提示されるすべての記事および製品情報は、情報提供を目的としています。BenchChemで購入可能な製品は、生体外研究のために特別に設計されています。生体外研究は、ラテン語の "in glass" に由来し、生物体の外で行われる実験を指します。これらの製品は医薬品または薬として分類されておらず、FDAから任何の医療状態、病気、または疾患の予防、治療、または治癒のために承認されていません。これらの製品を人間または動物に体内に導入する形態は、法律により厳格に禁止されています。これらのガイドラインに従うことは、研究と実験において法的および倫理的な基準の遵守を確実にするために重要です。