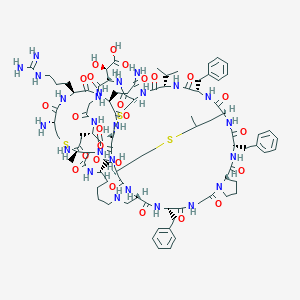
Cinnamycin
説明
Cinnamycin is a tetracyclic antibacterial peptide produced by Streptomyces cinnamoneus. It contains 19 amino acid residues including the unusual amino acids threo-3-methyl-lanthionine, meso-lanthionine, lysinoalanine, and 3-hydroxyaspartic acid . It belongs to the class of molecules known as lantibiotics, which are ribosomally synthesized post-translationally modified peptides .
Synthesis Analysis
The cinnamycin biosynthetic gene (cin) cluster of Streptomyces cinnamoneus DSM 40005 T contains 21 open reading frames (ORFs), among which cinA, cinM, cinX and cinorf7 are essential for cinnamycin synthesis . A novel mechanism of immunity has been discovered in which the producing organism methylates its entire complement of PE prior to the onset of cinnamycin production .Molecular Structure Analysis
The IUPAC name of Cinnamycin is quite complex, indicating its intricate molecular structure . The molecular weight of Cinnamycin is 2041.31 g·mol−1 .科学的研究の応用
Antimicrobial Activity
Cinnamycin exhibits potent antimicrobial properties , particularly against drug-resistant pathogens. Its mechanism involves binding to the membrane lipid phosphatidylethanolamine (PE) to elicit its activity . This makes it a valuable candidate for developing new antibiotics to combat the rising threat of antibiotic resistance.
Antiviral Applications
Beyond its antibacterial properties, cinnamycin has shown potential in antiviral therapy . Lanthipeptides like cinnamycin can be effective against a wide variety of viruses, offering a promising avenue for the treatment of viral infections .
Cystic Fibrosis Treatment
Cinnamycin may contribute to the treatment of cystic fibrosis . Its biological activities are not restricted to antimicrobials, and it has been demonstrated to alleviate symptoms associated with this condition .
Cancer Therapy
In the realm of oncology, cinnamycin has potential applications in cancer therapy . Its ability to interact with cell membranes and affect cellular processes can be harnessed to target cancerous cells .
Pain Management
The lanthipeptide has also been explored for its efficacy in pain management . Cinnamycin and similar compounds can modulate pain symptoms, providing an alternative to traditional painkillers .
Anti-inflammatory Properties
Cinnamycin possesses anti-inflammatory properties , making it useful in controlling inflammation. This application is particularly relevant in the treatment of chronic inflammatory diseases .
Analytical Tool in Cell Biology
Interestingly, cinnamycin can also serve as an analytical tool in cell biology research. It has been used to analyze the distribution and location of PE in target cells and tissues, providing insights into cellular dynamics .
将来の方向性
Antimicrobial peptides like Cinnamycin have gained significant attention for their clinical potential as a new class of antibiotics to combat antimicrobial resistance . Future research directions may include developing novel AMP structures, including hybrids, antimicrobial dendrimers and polypeptides, peptidomimetics, and AMP–drug conjugates .
特性
IUPAC Name |
(1S,4S,13S,16S,19S,22S,25S,28R,31S,37S,40S,41S,44R,47S,50S,53S,56R,65S,70S)-44-amino-37-(2-amino-2-oxoethyl)-50-(3-amino-3-oxopropyl)-4,16,22-tribenzyl-47-(3-carbamimidamidopropyl)-31-[(R)-carboxy(hydroxy)methyl]-41,70-dimethyl-2,5,8,14,17,20,23,26,29,32,35,38,45,48,51,54,57,67-octadecaoxo-25-propan-2-yl-42,69,72-trithia-3,6,9,15,18,21,24,27,30,33,36,39,46,49,52,55,58,60,66-nonadecazapentacyclo[38.18.9.319,56.328,53.09,13]triheptacontane-65-carboxylic acid | |
---|---|---|
Source | PubChem | |
URL | https://pubchem.ncbi.nlm.nih.gov | |
Description | Data deposited in or computed by PubChem | |
InChI |
InChI=1S/C89H125N25O25S3/c1-43(2)66-84(133)109-59-41-140-40-58-79(128)108-60-42-142-45(4)68(86(135)105-55(75(124)110-66)34-48-22-12-7-13-23-48)111-76(125)54(33-47-20-10-6-11-21-47)104-82(131)61-26-17-31-114(61)65(118)38-98-72(121)53(32-46-18-8-5-9-19-46)103-78(127)57(106-80(60)129)36-95-29-15-14-24-52(87(136)137)102-85(134)67(112-77(126)56(35-63(92)116)99-64(117)37-97-83(132)69(113-81(59)130)70(119)88(138)139)44(3)141-39-49(90)71(120)100-50(25-16-30-96-89(93)94)73(122)101-51(74(123)107-58)27-28-62(91)115/h5-13,18-23,43-45,49-61,66-70,95,119H,14-17,24-42,90H2,1-4H3,(H2,91,115)(H2,92,116)(H,97,132)(H,98,121)(H,99,117)(H,100,120)(H,101,122)(H,102,134)(H,103,127)(H,104,131)(H,105,135)(H,106,129)(H,107,123)(H,108,128)(H,109,133)(H,110,124)(H,111,125)(H,112,126)(H,113,130)(H,136,137)(H,138,139)(H4,93,94,96)/t44-,45-,49-,50-,51-,52-,53-,54-,55-,56-,57-,58+,59-,60-,61-,66-,67+,68+,69-,70+/m0/s1 | |
Source | PubChem | |
URL | https://pubchem.ncbi.nlm.nih.gov | |
Description | Data deposited in or computed by PubChem | |
InChI Key |
QJDWKBINWOWJNZ-OURZNGJWSA-N | |
Source | PubChem | |
URL | https://pubchem.ncbi.nlm.nih.gov | |
Description | Data deposited in or computed by PubChem | |
Canonical SMILES |
CC1C2C(=O)NC(C(=O)NC(C(=O)NC3CSCC4C(=O)NC(CS1)C(=O)NC(CNCCCCC(NC(=O)C(C(SCC(C(=O)NC(C(=O)NC(C(=O)N4)CCC(=O)N)CCCNC(=N)N)N)C)NC(=O)C(NC(=O)CNC(=O)C(NC3=O)C(C(=O)O)O)CC(=O)N)C(=O)O)C(=O)NC(C(=O)NCC(=O)N5CCCC5C(=O)NC(C(=O)N2)CC6=CC=CC=C6)CC7=CC=CC=C7)C(C)C)CC8=CC=CC=C8 | |
Source | PubChem | |
URL | https://pubchem.ncbi.nlm.nih.gov | |
Description | Data deposited in or computed by PubChem | |
Isomeric SMILES |
C[C@H]1[C@@H]2C(=O)N[C@H](C(=O)N[C@H](C(=O)N[C@H]3CSC[C@@H]4C(=O)N[C@@H](CS1)C(=O)N[C@@H](CNCCCC[C@H](NC(=O)[C@@H]([C@@H](SC[C@@H](C(=O)N[C@H](C(=O)N[C@H](C(=O)N4)CCC(=O)N)CCCNC(=N)N)N)C)NC(=O)[C@@H](NC(=O)CNC(=O)[C@@H](NC3=O)[C@H](C(=O)O)O)CC(=O)N)C(=O)O)C(=O)N[C@H](C(=O)NCC(=O)N5CCC[C@H]5C(=O)N[C@H](C(=O)N2)CC6=CC=CC=C6)CC7=CC=CC=C7)C(C)C)CC8=CC=CC=C8 | |
Source | PubChem | |
URL | https://pubchem.ncbi.nlm.nih.gov | |
Description | Data deposited in or computed by PubChem | |
Molecular Formula |
C89H125N25O25S3 | |
Source | PubChem | |
URL | https://pubchem.ncbi.nlm.nih.gov | |
Description | Data deposited in or computed by PubChem | |
Molecular Weight |
2041.3 g/mol | |
Source | PubChem | |
URL | https://pubchem.ncbi.nlm.nih.gov | |
Description | Data deposited in or computed by PubChem | |
Product Name |
(1S,4S,13S,16S,19S,22S,25S,28R,31S,37S,40S,41S,44R,47S,50S,53S,56R,65S,70S)-44-amino-37-(2-amino-2-oxoethyl)-50-(3-amino-3-oxopropyl)-4,16,22-tribenzyl-47-(3-carbamimidamidopropyl)-31-[(R)-carboxy(hydroxy)methyl]-41,70-dimethyl-2,5,8,14,17,20,23,26,29,32,35,38,45,48,51,54,57,67-octadecaoxo-25-propan-2-yl-42,69,72-trithia-3,6,9,15,18,21,24,27,30,33,36,39,46,49,52,55,58,60,66-nonadecazapentacyclo[38.18.9.319,56.328,53.09,13]triheptacontane-65-carboxylic acid | |
CAS RN |
110655-58-8 | |
Record name | CINNAMYCIN | |
Source | FDA Global Substance Registration System (GSRS) | |
URL | https://gsrs.ncats.nih.gov/ginas/app/beta/substances/UM32RAQ8SC | |
Description | The FDA Global Substance Registration System (GSRS) enables the efficient and accurate exchange of information on what substances are in regulated products. Instead of relying on names, which vary across regulatory domains, countries, and regions, the GSRS knowledge base makes it possible for substances to be defined by standardized, scientific descriptions. | |
Explanation | Unless otherwise noted, the contents of the FDA website (www.fda.gov), both text and graphics, are not copyrighted. They are in the public domain and may be republished, reprinted and otherwise used freely by anyone without the need to obtain permission from FDA. Credit to the U.S. Food and Drug Administration as the source is appreciated but not required. | |
Q & A
Q1: What is the primary target of Cinnamycin and how does it exert its biological activity?
A1: Cinnamycin specifically binds to Phosphatidylethanolamine (PE), a phospholipid commonly found in bacterial membranes. This binding is highly selective and requires both the PE headgroup and at least one fatty acyl chain. [] This interaction disrupts the membrane integrity, leading to cell death. [, , , , ]
Q2: How does membrane curvature affect Cinnamycin's binding to PE?
A2: Research shows that Cinnamycin exhibits curvature-dependent binding to PE-containing membranes. It binds more efficiently to small vesicles with higher curvature compared to larger liposomes with lower curvature. [, , ] This suggests that Cinnamycin might induce membrane deformation to enhance its binding to PE.
Q3: Does Cinnamycin's binding to PE influence the transbilayer movement of lipids?
A3: Yes, Cinnamycin binding induces transbilayer lipid movement, exposing inner leaflet PE to the extracellular environment. This process contributes to its cytotoxicity and promotes its own binding. []
Q4: Beyond its antimicrobial activity, what other cellular processes are affected by Cinnamycin?
A4: Cinnamycin has been shown to inhibit Phospholipase A2 activity due to its binding to PE. [, ] Additionally, it has demonstrated an ability to block the internalization of PE at the cleavage furrow during mitosis, potentially impacting cell division. []
Q5: What is the molecular formula and weight of Cinnamycin?
A5: Cinnamycin has a molecular formula of C98H138N26O32S4 and a molecular weight of 2145.5 g/mol. []
Q6: What are the key structural features of Cinnamycin?
A6: Cinnamycin is a tetracyclic peptide antibiotic composed of 19 amino acids. It contains one lanthionine and two methyllanthionine residues, characteristic of lantibiotics. [] Cinnamycin also possesses a unique lysinoalanine bridge and an erythro-3-hydroxy-l-aspartic acid. [] These modifications play a crucial role in its interaction with PE. [, ]
Q7: How does the structure of Cinnamycin influence its binding to PE?
A7: Cinnamycin possesses a hydrophobic pocket formed by residues Phenylalanine-7 to Alanine(S)-14, which accommodates the PE headgroup. [] The specific geometry of this pocket allows for the strict binding specificity observed with PE. []
Q8: What insights do we gain from the solution structures of Cinnamycin and its analogs?
A8: NMR studies of Cinnamycin and its analogs, duramycin B and C, reveal a U-shaped topology stabilized by thioether bridges. [] This clamp-like conformation contributes to their amphiphilic nature and ability to interact with membranes. []
Q9: How does the conformation of Cinnamycin change in different environments?
A9: While Cinnamycin adopts a specific conformation in DMSO, its structure changes significantly in aqueous solutions, SDS micelles, and lipid bilayers. [] These conformational changes are likely influenced by the polarity and hydrophobic interactions within each environment.
Q10: What is the impact of different environments on the stability of Cinnamycin?
A10: The stability of Cinnamycin is influenced by factors like temperature, pH, and the presence of other molecules. For example, complex formation between Cinnamycin and PE is enthalpy-driven in octyl glucoside micelles but involves both enthalpy and entropy contributions in bilayer membranes. [] This suggests that the surrounding environment significantly impacts the thermodynamic properties of Cinnamycin interactions.
Q11: Are there any specific challenges associated with the formulation and delivery of Cinnamycin?
A11: While specific data on formulation challenges are limited in the provided research, Cinnamycin's peptide nature and its tendency to interact with membranes may pose challenges in achieving optimal stability, solubility, and bioavailability.
Q12: Does Cinnamycin itself exhibit any catalytic activity?
A12: Cinnamycin itself is not an enzyme and does not possess intrinsic catalytic activity. Its biological activity primarily stems from its ability to bind specifically to PE and disrupt membrane integrity.
Q13: Have computational methods been used to study Cinnamycin and its interactions?
A13: Yes, molecular dynamics simulations have been employed to study the structure of Cinnamycin-lipid complexes and understand the basis for its selective binding to PE. [] These simulations provide valuable insights into the molecular interactions governing Cinnamycin's activity.
Q14: What is the role of the lysinoalanine bridge in Cinnamycin's activity?
A14: The lysinoalanine bridge, formed between Lysine-19 and a dehydrated Serine residue at position 6, is crucial for Cinnamycin's biological activity. []
Q15: How do modifications to the Cinnamycin peptide sequence affect its activity?
A15: Modifications to the Cinnamycin peptide sequence, such as amino acid substitutions, can significantly impact its activity. For example, replacing Phenylalanine-10 with Leucine results in Duramycin B, while Duramycin C harbors several other amino acid changes compared to Cinnamycin. [] These alterations likely influence their binding affinity and specificity for PE.
Q16: Can non-canonical amino acids be incorporated into Cinnamycin, and how does it affect its activity?
A16: Yes, using the pyrrolysyl-tRNA synthetase/tRNAPyl pair, researchers successfully incorporated non-canonical amino acids into Cinnamycin. [] The introduction of these non-canonical amino acids led to the generation of deoxycinnamycin derivatives with altered biological activity. [] This highlights the potential for engineering Cinnamycin variants with tailored properties.
Q17: What approaches have been explored to improve the production of Cinnamycin and its analogues?
A17: Researchers have investigated genetic engineering strategies to enhance Cinnamycin production. [, ] Additionally, the use of inorganic talc microparticles has been shown to significantly boost the production of Cinnamycin and other natural products in various actinobacteria, including Streptomyces. [] These approaches highlight the ongoing efforts to optimize the production of these valuable compounds.
Q18: What analytical techniques are commonly used to study Cinnamycin?
A18: Various techniques are employed to characterize and quantify Cinnamycin, including:
- High-performance liquid chromatography (HPLC): Used to purify and analyze Cinnamycin from complex mixtures. []
- Mass spectrometry (MS): Determines the molecular weight and analyzes the structure of Cinnamycin and its derivatives. [, , , ]
- Nuclear magnetic resonance (NMR): Provides detailed information about the three-dimensional structure and dynamics of Cinnamycin in different environments. [, , ]
- Circular dichroism (CD) spectroscopy: Used to study the secondary structure and conformational changes of Cinnamycin in solution. []
- Isothermal titration calorimetry (ITC): Measures the thermodynamic parameters of Cinnamycin binding to PE. [, ]
- Atomic force microscopy (AFM): Used to investigate the interaction forces and adhesion properties of Cinnamycin with model membranes. [, ]
試験管内研究製品の免責事項と情報
BenchChemで提示されるすべての記事および製品情報は、情報提供を目的としています。BenchChemで購入可能な製品は、生体外研究のために特別に設計されています。生体外研究は、ラテン語の "in glass" に由来し、生物体の外で行われる実験を指します。これらの製品は医薬品または薬として分類されておらず、FDAから任何の医療状態、病気、または疾患の予防、治療、または治癒のために承認されていません。これらの製品を人間または動物に体内に導入する形態は、法律により厳格に禁止されています。これらのガイドラインに従うことは、研究と実験において法的および倫理的な基準の遵守を確実にするために重要です。