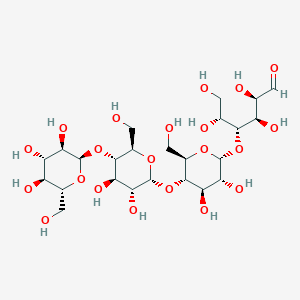
Maltotetraose
- 専門家チームからの見積もりを受け取るには、QUICK INQUIRYをクリックしてください。
- 品質商品を競争力のある価格で提供し、研究に集中できます。
説明
Maltotetraose, also known as Amylotetraose, is a tetrasaccharide composed of glucose molecules linked by α-1,4 glycosidic bonds . It is commonly found in B. stearothermophilus and has been shown to increase the α-amylase synthesis rate in B. stearothermophilus .
Synthesis Analysis
Maltotetraose is primarily derived from the hydrolysis of starch or maltodextrin . Traditional methods for its production encompass acid hydrolysis, enzyme hydrolysis, and acid-enzyme combinations, with enzyme hydrolysis being the predominant approach .
Molecular Structure Analysis
The molecular structure of Maltotetraose consists of four glucose units linked by α-1,4 glycosidic bonds . The chemical formula of Maltotetraose is C24H42O21, and its average molecular weight is 666.579 .
Chemical Reactions Analysis
Maltotetraose belongs to the class of organic compounds known as oligosaccharides. These are carbohydrates made up of 3 to 10 monosaccharide units linked to each other through glycosidic bonds .
Physical And Chemical Properties Analysis
Maltotetraose has a molecular weight of 666.58 . It is highly soluble in water and has a sweetness of one-fifth that of sucrose . It demonstrates superior moisture retention capability, produces much fewer Maillard reaction products in comparison to glucose or maltose, and exhibits high resistance to the retrogradation of starchy foods .
科学的研究の応用
Food Processing
Maltotetraose demonstrates remarkable versatility in food processing . It enhances dough rheology and baking quality , and it has superior moisture retention capability . It produces much less Maillard reaction products in comparison to glucose or maltose, and exhibits high resistance to the retrogradation of starchy foods . Because of these beneficial properties, maltotetraose has been used to improve the quality of numerous food products, including ice cream, chocolate, beverage, and bread .
Medical Applications
Maltotetraose exhibits specific physiological functions, suggesting promising applications in the medical sector . It can inhibit the early stages of atherosclerosis and suppress the growth of certain pathogenic bacteria . It also inhibits TNF-α-induced expression of intercellular adhesion molecule-1 (ICAM-1) .
Chemical Industry
Maltotetraose holds significant promise in the chemical industry . It is highly soluble in water and has a sweetness of one-fifth that of sucrose .
Energy Supply
Maltotetraose offers various benefits such as sustaining continuous energy supply to the human body .
Biochemical Research
Maltotetraose can be used as a substrate for the enzyme-coupled determination of amylase activity in biological fluids . This finds application in metabolomics and biochemical research .
Industrial Production
Maltotetraose is industrially produced through the hydrolysis of amylaceous polysaccharides catalyzed by maltotetraose amylase . A novel and efficient bio-physical method was developed to produce high-purity maltotetraose . This method underwent meticulous design and optimization, ensuring a production process that is environmentally friendly, safe, and efficient .
作用機序
Maltotetraose, also known as Amylotetraose or alpha-maltotetraose, is a linear maltooligosaccharide composed of four glucose units linked by α-1,4 glycosidic bonds . It demonstrates remarkable versatility in food processing and exhibits specific physiological functions .
Target of Action
Maltotetraose’s primary targets are the enzymes Glucan 1,4-alpha-maltotetraohydrolase in Pseudomonas stutzeri and Neopullulanase 2 in Thermoactinomyces vulgaris . These enzymes play a crucial role in the hydrolysis of amylaceous polysaccharides into maltooligosaccharides.
Mode of Action
Maltotetraose interacts with its target enzymes, catalyzing the hydrolysis of amylaceous polysaccharides into maltooligosaccharides, with maltotetraose being the main product . This interaction results in changes in the structure of the polysaccharides, breaking them down into simpler sugars.
Biochemical Pathways
The primary biochemical pathway affected by maltotetraose is the hydrolysis of amylaceous polysaccharides. This process is catalyzed by maltotetraose amylase, resulting in the production of maltooligosaccharides . The downstream effects include the production of simpler sugars that can be used in various metabolic processes.
Pharmacokinetics
It is known that maltotetraose is highly soluble in water , which could influence its absorption and distribution in the body.
Result of Action
The molecular and cellular effects of maltotetraose’s action include the breakdown of complex carbohydrates into simpler sugars. This can provide a continuous energy supply to the human body . Furthermore, maltotetraose can suppress the growth of several putrefactive bacteria while enhancing the intestinal environment .
Action Environment
Environmental factors such as temperature and pH can influence the action, efficacy, and stability of maltotetraose. For instance, the enzyme that interacts with maltotetraose, Pp-Mta∆CBM, has been found to have maximum activity at 55 ℃ and pH 7.0 . Therefore, these conditions could potentially enhance the efficacy of maltotetraose.
Safety and Hazards
Maltotetraose is considered safe for R&D use only. It is not intended for medicinal, household, or other uses . It is recommended to avoid dust formation, breathing mist, gas, or vapors, and contact with skin and eyes . Use of personal protective equipment and chemical impermeable gloves is advised .
将来の方向性
The escalating market demand for high-purity Maltotetraose underscores the need for more refined purification processes . Innovative and efficient production technology for oligosaccharides synthesis holds paramount importance . Genetic engineering and reaction optimization can enhance the production of oligosaccharides .
特性
IUPAC Name |
(2R,3R,4S,5S,6R)-2-[(2R,3S,4R,5R,6R)-6-[(2R,3S,4R,5R,6R)-4,5-dihydroxy-2-(hydroxymethyl)-6-[(2R,3S,4R,5R,6S)-4,5,6-trihydroxy-2-(hydroxymethyl)oxan-3-yl]oxyoxan-3-yl]oxy-4,5-dihydroxy-2-(hydroxymethyl)oxan-3-yl]oxy-6-(hydroxymethyl)oxane-3,4,5-triol |
Source
|
---|---|---|
Source | PubChem | |
URL | https://pubchem.ncbi.nlm.nih.gov | |
Description | Data deposited in or computed by PubChem | |
InChI |
InChI=1S/C24H42O21/c25-1-5-9(29)10(30)15(35)22(40-5)44-19-7(3-27)42-24(17(37)12(19)32)45-20-8(4-28)41-23(16(36)13(20)33)43-18-6(2-26)39-21(38)14(34)11(18)31/h5-38H,1-4H2/t5-,6-,7-,8-,9-,10+,11-,12-,13-,14-,15-,16-,17-,18-,19-,20-,21+,22-,23-,24-/m1/s1 |
Source
|
Source | PubChem | |
URL | https://pubchem.ncbi.nlm.nih.gov | |
Description | Data deposited in or computed by PubChem | |
InChI Key |
LUEWUZLMQUOBSB-ZLBHSGTGSA-N |
Source
|
Source | PubChem | |
URL | https://pubchem.ncbi.nlm.nih.gov | |
Description | Data deposited in or computed by PubChem | |
Canonical SMILES |
C(C1C(C(C(C(O1)OC2C(OC(C(C2O)O)OC3C(OC(C(C3O)O)OC4C(OC(C(C4O)O)O)CO)CO)CO)O)O)O)O |
Source
|
Source | PubChem | |
URL | https://pubchem.ncbi.nlm.nih.gov | |
Description | Data deposited in or computed by PubChem | |
Isomeric SMILES |
C([C@@H]1[C@H]([C@@H]([C@H]([C@H](O1)O[C@@H]2[C@H](O[C@@H]([C@@H]([C@H]2O)O)O[C@@H]3[C@H](O[C@@H]([C@@H]([C@H]3O)O)O[C@@H]4[C@H](O[C@@H]([C@@H]([C@H]4O)O)O)CO)CO)CO)O)O)O)O |
Source
|
Source | PubChem | |
URL | https://pubchem.ncbi.nlm.nih.gov | |
Description | Data deposited in or computed by PubChem | |
Molecular Formula |
C24H42O21 |
Source
|
Source | PubChem | |
URL | https://pubchem.ncbi.nlm.nih.gov | |
Description | Data deposited in or computed by PubChem | |
Molecular Weight |
666.6 g/mol |
Source
|
Source | PubChem | |
URL | https://pubchem.ncbi.nlm.nih.gov | |
Description | Data deposited in or computed by PubChem | |
CAS RN |
34612-38-9 |
Source
|
Record name | O-α-D-glucopyranosyl-(1→4)-O-α-D-glucopyranosyl-(1→4)-O-α-D-glucopyranosyl-(1→4)-D-glucose | |
Source | European Chemicals Agency (ECHA) | |
URL | https://echa.europa.eu/substance-information/-/substanceinfo/100.047.359 | |
Description | The European Chemicals Agency (ECHA) is an agency of the European Union which is the driving force among regulatory authorities in implementing the EU's groundbreaking chemicals legislation for the benefit of human health and the environment as well as for innovation and competitiveness. | |
Explanation | Use of the information, documents and data from the ECHA website is subject to the terms and conditions of this Legal Notice, and subject to other binding limitations provided for under applicable law, the information, documents and data made available on the ECHA website may be reproduced, distributed and/or used, totally or in part, for non-commercial purposes provided that ECHA is acknowledged as the source: "Source: European Chemicals Agency, http://echa.europa.eu/". Such acknowledgement must be included in each copy of the material. ECHA permits and encourages organisations and individuals to create links to the ECHA website under the following cumulative conditions: Links can only be made to webpages that provide a link to the Legal Notice page. | |
試験管内研究製品の免責事項と情報
BenchChemで提示されるすべての記事および製品情報は、情報提供を目的としています。BenchChemで購入可能な製品は、生体外研究のために特別に設計されています。生体外研究は、ラテン語の "in glass" に由来し、生物体の外で行われる実験を指します。これらの製品は医薬品または薬として分類されておらず、FDAから任何の医療状態、病気、または疾患の予防、治療、または治癒のために承認されていません。これらの製品を人間または動物に体内に導入する形態は、法律により厳格に禁止されています。これらのガイドラインに従うことは、研究と実験において法的および倫理的な基準の遵守を確実にするために重要です。