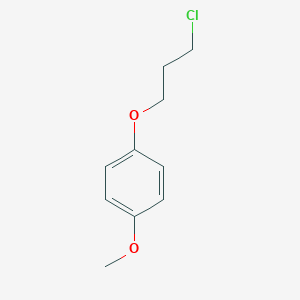
1-(3-クロロプロポキシ)-4-メトキシベンゼン
概要
説明
1-(3-Chloropropoxy)-4-methoxybenzene, also known as 1-(3-Chloropropoxy)-4-methoxybenzene, is a useful research compound. Its molecular formula is C10H13ClO2 and its molecular weight is 200.66 g/mol. The purity is usually 95%.
BenchChem offers high-quality 1-(3-Chloropropoxy)-4-methoxybenzene suitable for many research applications. Different packaging options are available to accommodate customers' requirements. Please inquire for more information about 1-(3-Chloropropoxy)-4-methoxybenzene including the price, delivery time, and more detailed information at info@benchchem.com.
科学的研究の応用
分子自己組織化とグラフェンおよびフラーレン
“1-(3-クロロプロポキシ)-4-メトキシベンゼン”は、グラフェンおよびフラーレンとの分子自己組織化を形成する能力について研究されています。 これらの集合体は物理化学的特性を強化し、表面増強ラマン分光法(SERS)による薬理学的サンプル中の薬物の検出に使用することができます .
光起電力効率研究
“1-(3-クロロプロポキシ)-4-メトキシベンゼン”とグラフェンおよびフラーレンの相互作用は、光起電力用途のために調査されてきました。 この化合物の電子スペクトルは、太陽エネルギー変換における潜在的な使用を示唆しています .
生物活性増強
“1-(3-クロロプロポキシ)-4-メトキシベンゼン”がグラフェンまたはフラーレン表面に吸着されると、生物活性が増加することが示されています。 これは、より効果的な医薬品用途につながる可能性があります .
水酸化触媒作用
研究によると、 “1-(3-クロロプロポキシ)-4-メトキシベンゼン”は、特に有機合成において重要な、一次C-H結合の効率的な触媒作用において、水酸化反応に関与する可能性があります.
揮発性有機化合物の速度論研究
原子状塩素を含む反応におけるこの化合物の挙動が研究されており、さまざまな化学プロセスにおけるその速度論と反応性を理解するために関連しています.
塩素化プロセス
シクロヘキサン誘導体の塩素化方法が “1-(3-クロロプロポキシ)-4-メトキシベンゼン”に適用されており、穏やかな条件下で効率的な塩素化技術の開発におけるその使用を示唆しています.
作用機序
Target of Action
The primary targets of 1-(3-Chloropropoxy)-4-methoxybenzene are insect odorant binding proteins and/or acetylcholinesterase . These proteins play a crucial role in the nervous system of insects, where odorant binding proteins are involved in the detection of environmental chemicals, and acetylcholinesterase is responsible for the termination of nerve impulses by breaking down the neurotransmitter acetylcholine.
Mode of Action
1-(3-Chloropropoxy)-4-methoxybenzene interacts with its targets by forming stable complexes . This interaction can inhibit the normal function of the target proteins, leading to changes in the physiological processes of the insects.
Biochemical Pathways
The affected pathways are those involved in the detection of environmental chemicals and the transmission of nerve impulses in insects. The inhibition of odorant binding proteins can disrupt the insects’ ability to detect food sources or mates, while the inhibition of acetylcholinesterase can lead to an overaccumulation of acetylcholine, causing continuous nerve impulses that can lead to paralysis or death .
Result of Action
The molecular and cellular effects of 1-(3-Chloropropoxy)-4-methoxybenzene’s action include the disruption of normal physiological processes in insects, potentially leading to paralysis or death . It’s worth noting that some derivatives of this compound have been found to be less toxic to human cells than the naturally-derived starting materials , suggesting potential for selective toxicity.
特性
IUPAC Name |
1-(3-chloropropoxy)-4-methoxybenzene | |
---|---|---|
Source | PubChem | |
URL | https://pubchem.ncbi.nlm.nih.gov | |
Description | Data deposited in or computed by PubChem | |
InChI |
InChI=1S/C10H13ClO2/c1-12-9-3-5-10(6-4-9)13-8-2-7-11/h3-6H,2,7-8H2,1H3 | |
Source | PubChem | |
URL | https://pubchem.ncbi.nlm.nih.gov | |
Description | Data deposited in or computed by PubChem | |
InChI Key |
SJZQJMFKQYZZAT-UHFFFAOYSA-N | |
Source | PubChem | |
URL | https://pubchem.ncbi.nlm.nih.gov | |
Description | Data deposited in or computed by PubChem | |
Canonical SMILES |
COC1=CC=C(C=C1)OCCCCl | |
Source | PubChem | |
URL | https://pubchem.ncbi.nlm.nih.gov | |
Description | Data deposited in or computed by PubChem | |
Molecular Formula |
C10H13ClO2 | |
Source | PubChem | |
URL | https://pubchem.ncbi.nlm.nih.gov | |
Description | Data deposited in or computed by PubChem | |
Molecular Weight |
200.66 g/mol | |
Source | PubChem | |
URL | https://pubchem.ncbi.nlm.nih.gov | |
Description | Data deposited in or computed by PubChem | |
Synthesis routes and methods I
Procedure details
Synthesis routes and methods II
Procedure details
試験管内研究製品の免責事項と情報
BenchChemで提示されるすべての記事および製品情報は、情報提供を目的としています。BenchChemで購入可能な製品は、生体外研究のために特別に設計されています。生体外研究は、ラテン語の "in glass" に由来し、生物体の外で行われる実験を指します。これらの製品は医薬品または薬として分類されておらず、FDAから任何の医療状態、病気、または疾患の予防、治療、または治癒のために承認されていません。これらの製品を人間または動物に体内に導入する形態は、法律により厳格に禁止されています。これらのガイドラインに従うことは、研究と実験において法的および倫理的な基準の遵守を確実にするために重要です。