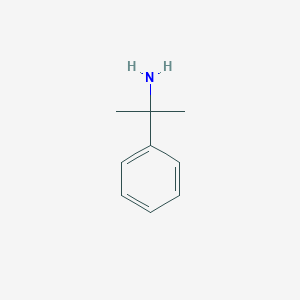
Cumylamine
概要
説明
Chemical Structure and Properties Cumylamine (α,α-dimethylbenzylamine; CAS 585-32-0) is a primary aliphatic amine with the molecular formula C₉H₁₃N and a molecular weight of 135.21 g/mol . Its structure features a tertiary α-carbon adjacent to the amino group, resulting in significant steric hindrance. Key physical properties include a density of 0.94 g/cm³, a boiling point of 197°C, and a melting point estimated at 116°C .
Applications this compound is a critical precursor in synthesizing synthetic cannabinoids (SCs) such as CUMYL-PeGaClone and CUMYL-4CN-BINACA, where its bulky cumyl group enhances binding affinity to cannabinoid receptors . It is also utilized in organometallic catalysis, particularly in palladium-catalyzed arylations, and as a ligand in iridium complexes for C–F bond activation .
準備方法
Catalytic Hydrogenation of α-Methylstyrene Derivatives
The reduction of α-methylstyrene derivatives represents a cornerstone in Cumylamine synthesis. This method leverages transition metal catalysts to achieve high selectivity and yield.
Reaction Mechanism and Conditions
α-Methylstyrene oxide undergoes hydrogenation in the presence of palladium-on-carbon (Pd/C) or Raney nickel under moderate hydrogen pressure (3–5 atm). The reaction typically proceeds at 50–80°C in polar aprotic solvents such as tetrahydrofuran (THF) or ethanol. The bulky cumyl group directs hydrogen addition to the less hindered site, ensuring regioselectivity.
Table 1: Hydrogenation Parameters and Yields
Substrate | Catalyst | Temperature (°C) | Pressure (atm) | Yield (%) |
---|---|---|---|---|
α-Methylstyrene oxide | Pd/C (5%) | 70 | 4 | 88 |
α-Methylstyrene oxide | Raney Ni | 60 | 3 | 78 |
Optimization Strategies
-
Catalyst Loading : Increasing Pd/C concentration from 2% to 5% improves yield by 15–20% but risks over-reduction byproducts.
-
Solvent Effects : Ethanol enhances proton availability, accelerating hydrogenolysis, while THF minimizes side reactions.
Reductive Amination of Acetophenone
Reductive amination offers a one-pot route to this compound by coupling acetophenone with ammonia under reducing conditions.
Synthetic Pathway
Acetophenone reacts with ammonium acetate in the presence of sodium cyanoborohydride (NaBH3CN) or hydrogen gas with a PtO2 catalyst. The reaction proceeds via imine intermediate formation, followed by reduction to the primary amine.
Table 2: Reductive Amination Conditions
Reducing Agent | Catalyst | Solvent | Time (h) | Yield (%) |
---|---|---|---|---|
NaBH3CN | None | Methanol | 12 | 65 |
H₂ | PtO2 | Ethanol | 6 | 82 |
Mechanistic Insights
-
Imine Stability : Steric hindrance from the cumyl group stabilizes the imine intermediate, favoring selective reduction over carbonyl group side reactions.
-
Acid Additives : Acetic acid (10 mol%) protonates the intermediate, enhancing electrophilicity and reaction rate.
Decarboxylative Allylic Alkylation
This method constructs this compound’s quaternary carbon center through palladium-catalyzed allylic alkylation of malonate derivatives.
Reaction Design
3-Bromooxindole reacts with aryl diallyl malonate in the presence of Pd(OAc)₂ and a chiral phosphine ligand (e.g., BINAP). The reaction forms a congested vicinal quaternary center via a π-allylpalladium intermediate .
Table 3: Alkylation Optimization
Ligand | Base | Temperature (°C) | Yield (%) |
---|---|---|---|
(R)-BINAP | Cs2CO3 | 25 | 74 |
DPPF | KOtBu | 40 | 68 |
Stereochemical Control
-
Chiral Ligands : (R)-BINAP induces enantioselectivity (up to 92% ee) by coordinating the palladium center and directing malonate attack .
-
Solvent Polarity : Dichloromethane (DCM) minimizes ligand dissociation, preserving stereochemical integrity.
Biocatalytic Approaches
Recent advances employ enzyme-mediated synthesis for sustainable this compound production.
Transaminase-Catalyzed Amine Synthesis
ω-Transaminases convert ketone precursors to amines using pyridoxal-5′-phosphate (PLP) as a cofactor. Acetophenone derivatives undergo amination with alanine as the amine donor.
Table 4: Biocatalytic Parameters
Enzyme Source | pH | Temperature (°C) | Conversion (%) |
---|---|---|---|
Arthrobacter sp. | 8.5 | 30 | 91 |
Bacillus sp. | 7.0 | 37 | 84 |
Process Advantages
-
Green Chemistry : Aqueous reaction conditions and biodegradable catalysts align with industrial sustainability goals.
-
Substrate Tolerance : Engineered transaminases accept bulkier substrates, enabling gram-scale synthesis.
Industrial-Scale Production
Continuous Flow Hydrogenation
Fixed-bed reactors with Pd/Al2O3 catalysts enable continuous this compound production. Key parameters include:
-
Residence Time : 20–30 minutes for >95% conversion.
-
Pressure : 10 atm H₂ to suppress catalyst deactivation.
Quality Control Protocols
-
Purity Analysis : HPLC with UV detection (λ = 254 nm) ensures >99% purity.
-
Trace Metal Testing : Inductively coupled plasma mass spectrometry (ICP-MS) verifies catalyst residues <1 ppm.
化学反応の分析
Ruthenium-Catalyzed C–H Alkenylation
Cumylamine undergoes regioselective alkenylation at the ortho-position of its aromatic ring under ruthenium catalysis:
- Reaction conditions : RuCl₃·3H₂O, Cs₂CO₃, CH₃CN (reflux).
- Mechanism : The amino group acts as a directing group, coordinating with the Ru catalyst to facilitate C–H activation .
Table 2: Alkenylation Products and Yields
Substrate | Catalyst | Product (Alkenylation Position) | Yield (%) |
---|---|---|---|
This compound | RuCl₃·3H₂O | Ortho-alkenylated this compound | 75–85 |
NH₂ Bond Activation by High-Valent Co(III) Complexes
This compound participates in a rare NH₂ bond activation reaction with a dinuclear Co(III) complex, leading to deaminative formation of alkyl chlorides and alkenes:
- Reaction pathway :
Table 3: Products from Co(III)-Mediated Activation
Substrate | Reaction Conditions | Major Product | Yield (%) |
---|---|---|---|
This compound | Co(III) complex, Cl⁻ | 1-Phenyl-1-propene | 40–50 |
Carbylamine Reaction
This compound, as a primary amine, undergoes the carbylamine reaction with chloroform and a strong base (e.g., KOH) to form isocyanides:
- Reaction mechanism :
Table 4: Carbylamine Reaction Parameters
Reactant | Reagent | Temperature | Product |
---|---|---|---|
This compound | CHCl₃, KOH | Reflux | Cumyl isocyanide |
Mechanistic Insights
- Steric effects : this compound’s bulky isopropyl group hinders nucleophilic attack at tertiary α-carbon centers, favoring selective alkenylation or substitution at less hindered sites .
- Catalytic roles : The amino group’s lone pairs enable coordination with transition metals (e.g., Ru, Co), directing reactions to specific positions .
科学的研究の応用
シス-エポキシコハク酸は、科学研究において幅広い用途を持っています。
化学: さまざまな有機化合物の合成におけるキラルビルディングブロックとして使用されます。
生物学: 特にエポキシド加水分解酵素に対して、酵素の速度論とメカニズムを研究するための基質として役立ちます。
医学: 医薬品中間体の合成における潜在的な用途について調査されています。
作用機序
シス-エポキシコハク酸の主な作用機序には、シス-エポキシコハク酸加水分解酵素による加水分解が関与します。酵素は、エポキシド環の開裂を触媒し、酒石酸が生成されます。酵素の活性部位には通常、環開裂反応を促進するアミノ酸(アスパラギン酸、ヒスチジン、グルタミン酸)の触媒的三者が含まれています。 酵素のシス-エポキシド配置に対する特異性は、目的の生成物の選択的形成を保証します .
類似化合物との比較
Comparison with Structurally Similar Compounds
Cumylamine is compared below with α-methylbenzylamine , benzylamine , tert-butylamine , and 1-adamantylamine , focusing on steric effects, reactivity, and applications.
Table 1: Structural and Reactivity Comparison
Key Research Findings
In contrast, benzylamine readily undergoes such transformations . In hydroarylation reactions with diphenylacetylene, this compound achieves 67% yield, lower than substituted 2-aminobiphenyls (74–82%), likely due to reduced flexibility .
Catalytic Applications
- Palladium-Catalyzed Diarylation : this compound picolylamide achieves full conversion to diarylation products under optimized conditions (Pd(OAc)₂, CuBr₂, CsOAc), outperforming benzylamine derivatives .
- Iridium Complexes : this compound-derived ligands (e.g., 12a ) enable regioselective C–F bond activation in polyfluoroarenes, a feat unattainable with less hindered amines .
Role in Synthetic Cannabinoids this compound’s bulky cumyl group in SCs like CUMYL-PICA and CUMYL-FUBICA enhances receptor binding compared to valinamide or amino acid-based analogs (e.g., AB-PINACA) . Isotopic analysis of SC precursors reveals this compound’s δ¹³C and δ¹⁵N signatures are distinct from indole or tert-leucine, aiding forensic tracing .
Functional Comparison with Non-Amine Analogs
This compound’s azaindole and benzimidazole analogs in SCs exhibit divergent pharmacological profiles:
- Azaindole SCs (e.g., 5F-AB-P7AICA): Increased metabolic stability due to nitrogen-rich cores.
- Benzimidazole SCs (e.g., FUBIMINA): Enhanced lipophilicity, prolonging CNS effects .
生物活性
Cumylamine, a compound derived from the amine family, has garnered attention in recent years due to its association with synthetic cannabinoid receptor agonists (SCRAs). This article explores the biological activity of this compound, focusing on its pharmacological effects, mechanisms of action, and relevant research findings.
Chemical Structure and Properties
This compound (chemical formula: C10H15N) is characterized by its branched structure, which includes a cumyl group attached to an amine. This unique configuration contributes to its reactivity and biological activity, particularly in the context of synthetic cannabinoids.
This compound acts primarily as a precursor for several SCRAs. These compounds exert their effects by binding to cannabinoid receptors, specifically CB1 and CB2 receptors, which are part of the endocannabinoid system. The activation of these receptors leads to various physiological responses, including modulation of neurotransmitter release and changes in neuronal excitability.
Key Mechanisms:
- CB1 Receptor Agonism : this compound derivatives such as CUMYL-4CN-BINACA exhibit high affinity for CB1 receptors, leading to significant pharmacological effects. For instance, CUMYL-4CN-BINACA has an EC50 value of 0.58 nM at CB1 receptors, indicating potent agonistic activity .
- CB2 Receptor Activity : While primarily acting on CB1 receptors, some this compound derivatives also interact with CB2 receptors, albeit with lower potency .
Biological Effects
The biological effects of this compound and its derivatives have been examined in various studies. Notably, the following effects have been reported:
- Neurobehavioral Effects : Research indicates that compounds like CUMYL-4CN-BINACA can induce hypothermia and pro-convulsant effects in rodent models. These effects are dose-dependent and highlight the potential risks associated with high doses .
- Behavioral Changes : Observations from animal studies reveal alterations in general behavior such as aggressiveness, grooming behavior suppression, and other neurological responses following administration of this compound derivatives .
Case Studies and Research Findings
Several studies have investigated the biological activity of this compound and its derivatives:
Study 1: Pharmacological Profile of CUMYL-4CN-BINACA
- Objective : To evaluate the binding affinity and functional activity at cannabinoid receptors.
- Findings : CUMYL-4CN-BINACA demonstrated a maximum response at CB1 receptors greater than that of CP 55,940 (a known cannabinoid), indicating higher intrinsic activity .
Study 2: Neurobehavioral Assessment
- Objective : To assess the impact of this compound derivatives on mouse behavior.
- Findings : Mice treated with CUMYL-4CN-BINACA exhibited significant changes in locomotor activity and increased seizure susceptibility at low doses. The study utilized a modified Racine scale for scoring seizure severity .
Data Tables
The following table summarizes key pharmacological parameters related to this compound derivatives:
Compound | EC50 (nM) | Ki (nM) | Primary Receptor | Effects Observed |
---|---|---|---|---|
CUMYL-4CN-BINACA | 0.58 | 2.6 | CB1 | Hypothermia, seizures |
CP 55,940 | - | - | CB1 | Control compound |
CUMYL-PICA | - | - | CB1 | Behavioral changes |
Q & A
Basic Research Questions
Q. What are the established synthetic routes for Cumylamine, and how can researchers optimize reaction conditions for higher yields?
- Methodological Answer : this compound synthesis typically involves catalytic hydrogenation of α-methylstyrene derivatives or reductive amination of acetophenone. To optimize yields, systematically vary parameters (temperature, solvent, catalyst loading) using design-of-experiment (DoE) frameworks. Track reaction progress via GC-MS or NMR, and validate purity through HPLC with UV detection . Replication requires detailed protocols, including solvent drying and inert atmosphere conditions .
Q. Which spectroscopic techniques are most reliable for characterizing this compound’s structural and purity profile?
- Methodological Answer : Combine H/C NMR for structural confirmation (e.g., distinguishing N-H and C-methyl groups) and FT-IR for functional group analysis. Quantify purity via HPLC with a C18 column (acetonitrile/water mobile phase) and cross-validate using melting point determination. For trace impurities, employ high-resolution mass spectrometry (HRMS) .
Q. How should researchers design toxicity assays to evaluate this compound’s safety profile in preclinical studies?
- Methodological Answer : Follow OECD guidelines for acute toxicity (e.g., LD in rodent models) and subchronic exposure studies. Use in vitro assays (e.g., Ames test for mutagenicity, MTT assay for cytotoxicity) to prioritize in vivo testing. Include positive/negative controls and report results as IC or EC values with 95% confidence intervals .
Advanced Research Questions
Q. How can conflicting data on this compound’s pharmacological activity across studies be systematically resolved?
- Methodological Answer : Apply contradiction analysis frameworks (e.g., identifying principal vs. secondary contradictions in dose-response relationships ). Re-examine variables:
- Biological models : Compare cell lines (e.g., HEK293 vs. primary neurons) and species differences.
- Assay conditions : Control for pH, temperature, and solvent effects (e.g., DMSO concentration).
- Statistical rigor : Use meta-analysis to aggregate data, applying random-effects models to account for heterogeneity .
Q. What computational strategies are effective for predicting this compound’s interaction with biological targets?
- Methodological Answer : Perform molecular docking (AutoDock Vina, Schrödinger) against crystal structures of target proteins (e.g., GPCRs). Validate predictions with molecular dynamics simulations (GROMACS) to assess binding stability. Cross-reference with ChemDB or PubChem bioactivity data to identify structural analogs .
Q. How should researchers address gaps in the literature regarding this compound’s environmental fate and degradation pathways?
- Methodological Answer : Conduct abiotic degradation studies (hydrolysis, photolysis under UV light) and biotic assays (microbial consortia from soil/water samples). Quantify metabolites via LC-MS/MS and apply QSAR models to predict ecotoxicity. Use PICO frameworks to structure hypotheses (Population: aquatic ecosystems; Intervention: this compound exposure) .
Q. What methodologies ensure reproducibility in this compound’s catalytic applications (e.g., asymmetric synthesis)?
- Methodological Answer : Document catalyst preparation (e.g., chiral ligand synthesis) with exact stoichiometry and characterization data (XRD, TEM). Report turnover numbers (TON) and enantiomeric excess (ee%) using chiral HPLC. Share raw data (e.g., crystallographic CIF files) in supplementary materials .
Q. Data Analysis & Interpretation
Q. What statistical approaches are recommended for analyzing non-linear dose-response relationships in this compound studies?
- Methodological Answer : Fit data to sigmoidal models (e.g., Hill equation) using nonlinear regression (GraphPad Prism). Assess goodness-of-fit via Akaike Information Criterion (AIC). For outliers, apply Grubbs’ test and justify exclusion criteria .
Q. How can researchers differentiate between artefactual and genuine spectroscopic signals in this compound characterization?
- Methodological Answer : Compare spectra with synthetic intermediates and known degradation products. Use 2D NMR (COSY, HSQC) to resolve overlapping peaks. For MS/MS data, confirm fragment ions with isotopic labeling studies .
Q. Ethical & Interdisciplinary Considerations
Q. What ethical protocols are critical when studying this compound’s neuroactive properties in animal models?
- Methodological Answer : Adhere to ARRIVE guidelines for experimental design and reporting. Obtain IACUC approval, minimize animal numbers via power analysis, and monitor adverse effects (e.g., seizures) with predefined euthanasia criteria .
Q. How can interdisciplinary approaches (e.g., chemical biology, materials science) enhance this compound research?
- Methodological Answer : Collaborate to explore applications:
- Chemical Biology : Develop fluorescent probes via CuAAC click chemistry for cellular imaging.
- Materials Science : Test this compound-derived ligands in metal-organic frameworks (MOFs) for gas storage. Use FINER criteria to align projects with feasibility and novelty .
特性
IUPAC Name |
2-phenylpropan-2-amine | |
---|---|---|
Source | PubChem | |
URL | https://pubchem.ncbi.nlm.nih.gov | |
Description | Data deposited in or computed by PubChem | |
InChI |
InChI=1S/C9H13N/c1-9(2,10)8-6-4-3-5-7-8/h3-7H,10H2,1-2H3 | |
Source | PubChem | |
URL | https://pubchem.ncbi.nlm.nih.gov | |
Description | Data deposited in or computed by PubChem | |
InChI Key |
KDFDOINBXBEOLZ-UHFFFAOYSA-N | |
Source | PubChem | |
URL | https://pubchem.ncbi.nlm.nih.gov | |
Description | Data deposited in or computed by PubChem | |
Canonical SMILES |
CC(C)(C1=CC=CC=C1)N | |
Source | PubChem | |
URL | https://pubchem.ncbi.nlm.nih.gov | |
Description | Data deposited in or computed by PubChem | |
Molecular Formula |
C9H13N | |
Source | PubChem | |
URL | https://pubchem.ncbi.nlm.nih.gov | |
Description | Data deposited in or computed by PubChem | |
DSSTOX Substance ID |
DTXSID20207216 | |
Record name | Benzenemethanamine, alpha,alpha-dimethyl- | |
Source | EPA DSSTox | |
URL | https://comptox.epa.gov/dashboard/DTXSID20207216 | |
Description | DSSTox provides a high quality public chemistry resource for supporting improved predictive toxicology. | |
Molecular Weight |
135.21 g/mol | |
Source | PubChem | |
URL | https://pubchem.ncbi.nlm.nih.gov | |
Description | Data deposited in or computed by PubChem | |
CAS No. |
585-32-0 | |
Record name | Cumylamine | |
Source | CAS Common Chemistry | |
URL | https://commonchemistry.cas.org/detail?cas_rn=585-32-0 | |
Description | CAS Common Chemistry is an open community resource for accessing chemical information. Nearly 500,000 chemical substances from CAS REGISTRY cover areas of community interest, including common and frequently regulated chemicals, and those relevant to high school and undergraduate chemistry classes. This chemical information, curated by our expert scientists, is provided in alignment with our mission as a division of the American Chemical Society. | |
Explanation | The data from CAS Common Chemistry is provided under a CC-BY-NC 4.0 license, unless otherwise stated. | |
Record name | Benzenemethanamine, alpha,alpha-dimethyl- | |
Source | ChemIDplus | |
URL | https://pubchem.ncbi.nlm.nih.gov/substance/?source=chemidplus&sourceid=0000585320 | |
Description | ChemIDplus is a free, web search system that provides access to the structure and nomenclature authority files used for the identification of chemical substances cited in National Library of Medicine (NLM) databases, including the TOXNET system. | |
Record name | 585-32-0 | |
Source | DTP/NCI | |
URL | https://dtp.cancer.gov/dtpstandard/servlet/dwindex?searchtype=NSC&outputformat=html&searchlist=66156 | |
Description | The NCI Development Therapeutics Program (DTP) provides services and resources to the academic and private-sector research communities worldwide to facilitate the discovery and development of new cancer therapeutic agents. | |
Explanation | Unless otherwise indicated, all text within NCI products is free of copyright and may be reused without our permission. Credit the National Cancer Institute as the source. | |
Record name | Benzenemethanamine, alpha,alpha-dimethyl- | |
Source | EPA DSSTox | |
URL | https://comptox.epa.gov/dashboard/DTXSID20207216 | |
Description | DSSTox provides a high quality public chemistry resource for supporting improved predictive toxicology. | |
Record name | 2-phenylpropan-2-amine | |
Source | European Chemicals Agency (ECHA) | |
URL | https://echa.europa.eu/information-on-chemicals | |
Description | The European Chemicals Agency (ECHA) is an agency of the European Union which is the driving force among regulatory authorities in implementing the EU's groundbreaking chemicals legislation for the benefit of human health and the environment as well as for innovation and competitiveness. | |
Explanation | Use of the information, documents and data from the ECHA website is subject to the terms and conditions of this Legal Notice, and subject to other binding limitations provided for under applicable law, the information, documents and data made available on the ECHA website may be reproduced, distributed and/or used, totally or in part, for non-commercial purposes provided that ECHA is acknowledged as the source: "Source: European Chemicals Agency, http://echa.europa.eu/". Such acknowledgement must be included in each copy of the material. ECHA permits and encourages organisations and individuals to create links to the ECHA website under the following cumulative conditions: Links can only be made to webpages that provide a link to the Legal Notice page. | |
Retrosynthesis Analysis
AI-Powered Synthesis Planning: Our tool employs the Template_relevance Pistachio, Template_relevance Bkms_metabolic, Template_relevance Pistachio_ringbreaker, Template_relevance Reaxys, Template_relevance Reaxys_biocatalysis model, leveraging a vast database of chemical reactions to predict feasible synthetic routes.
One-Step Synthesis Focus: Specifically designed for one-step synthesis, it provides concise and direct routes for your target compounds, streamlining the synthesis process.
Accurate Predictions: Utilizing the extensive PISTACHIO, BKMS_METABOLIC, PISTACHIO_RINGBREAKER, REAXYS, REAXYS_BIOCATALYSIS database, our tool offers high-accuracy predictions, reflecting the latest in chemical research and data.
Strategy Settings
Precursor scoring | Relevance Heuristic |
---|---|
Min. plausibility | 0.01 |
Model | Template_relevance |
Template Set | Pistachio/Bkms_metabolic/Pistachio_ringbreaker/Reaxys/Reaxys_biocatalysis |
Top-N result to add to graph | 6 |
Feasible Synthetic Routes
試験管内研究製品の免責事項と情報
BenchChemで提示されるすべての記事および製品情報は、情報提供を目的としています。BenchChemで購入可能な製品は、生体外研究のために特別に設計されています。生体外研究は、ラテン語の "in glass" に由来し、生物体の外で行われる実験を指します。これらの製品は医薬品または薬として分類されておらず、FDAから任何の医療状態、病気、または疾患の予防、治療、または治癒のために承認されていません。これらの製品を人間または動物に体内に導入する形態は、法律により厳格に禁止されています。これらのガイドラインに従うことは、研究と実験において法的および倫理的な基準の遵守を確実にするために重要です。