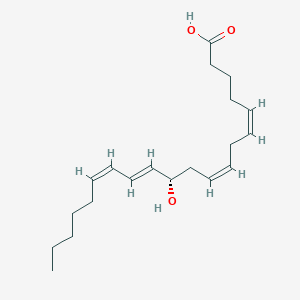
11S-HETE
概要
説明
(5Z,8Z,11S,12E,14Z)-11-hydroxyicosa-5,8,12,14-tetraenoic acid is a complex organic compound with significant importance in various scientific fields. This compound is characterized by its unique structure, which includes multiple double bonds and a hydroxyl group, making it a subject of interest in organic chemistry and biochemistry.
科学的研究の応用
Chemistry
In chemistry, (5Z,8Z,11S,12E,14Z)-11-hydroxyicosa-5,8,12,14-tetraenoic acid is used as a building block for the synthesis of more complex molecules. Its unique structure makes it a valuable intermediate in organic synthesis.
Biology
In biological research, this compound is studied for its potential role in cellular signaling pathways. Its multiple double bonds and hydroxyl group suggest it may interact with various biological molecules.
Medicine
In medicine, (5Z,8Z,11S,12E,14Z)-11-hydroxyicosa-5,8,12,14-tetraenoic acid is investigated for its potential therapeutic effects. It may have anti-inflammatory properties and could be used in the development of new drugs.
Industry
In industry, this compound is used in the production of specialty chemicals and materials. Its unique properties make it suitable for use in high-performance polymers and coatings.
作用機序
Target of Action
It is known that 11(s)-hete is a metabolite produced during a metabolic reaction in a mouse (mus musculus)
Mode of Action
It is known that 11(S)-HETE is formed non-enzymatically from arachidonic acid . This suggests that it may interact with its targets through non-enzymatic mechanisms. More research is needed to fully understand the interaction of 11(S)-HETE with its targets and the resulting changes.
Biochemical Pathways
11(S)-HETE is involved in the lipoxygenase branches of the eicosanoid pathways . These pathways involve the synthesis of hydroxyeicosatetraenoic acids (HETEs), lipoxins, and hepoxilins, with arachidonic acid as the starting point
Pharmacokinetics
It is known that levels of 11(s)-hete are higher than those of 11®-hete in isolated human plasma and serum . This suggests that 11(S)-HETE may have different pharmacokinetic properties compared to its R-enantiomer.
Result of Action
It is known that 11(s)-hete is involved in numerous biological roles, including homeostasis of blood pressure and blood flow, perception of pain, cell survival, initiation and resolution of inflammation, and progression of numerous disease states .
Action Environment
Given that 11(s)-hete is formed non-enzymatically from arachidonic acid , it is possible that factors affecting the availability of arachidonic acid or the conditions for non-enzymatic reactions could influence the action of 11(S)-HETE.
準備方法
Synthetic Routes and Reaction Conditions
The synthesis of (5Z,8Z,11S,12E,14Z)-11-hydroxyicosa-5,8,12,14-tetraenoic acid typically involves multiple steps, including the formation of the carbon backbone and the introduction of double bonds and functional groups. One common method involves the use of palladium-catalyzed cross-coupling reactions to form the carbon-carbon bonds, followed by selective hydrogenation and hydroxylation steps to introduce the desired functional groups.
Industrial Production Methods
Industrial production of this compound may involve large-scale organic synthesis techniques, including the use of continuous flow reactors to ensure precise control over reaction conditions. The use of advanced purification methods, such as chromatography and crystallization, is essential to obtain the compound in high purity.
化学反応の分析
Types of Reactions
(5Z,8Z,11S,12E,14Z)-11-hydroxyicosa-5,8,12,14-tetraenoic acid undergoes various chemical reactions, including:
Oxidation: The hydroxyl group can be oxidized to form a ketone or aldehyde.
Reduction: The double bonds can be reduced to single bonds using hydrogenation.
Substitution: The hydroxyl group can be substituted with other functional groups through nucleophilic substitution reactions.
Common Reagents and Conditions
Oxidation: Common oxidizing agents include potassium permanganate and chromium trioxide.
Reduction: Hydrogen gas in the presence of a palladium catalyst is often used for hydrogenation.
Substitution: Nucleophiles such as halides or amines can be used in substitution reactions.
Major Products
The major products formed from these reactions depend on the specific conditions and reagents used. For example, oxidation can yield ketones or aldehydes, while reduction can produce saturated hydrocarbons.
類似化合物との比較
Similar Compounds
Arachidonic Acid: A polyunsaturated fatty acid with a similar carbon backbone but different functional groups.
Eicosapentaenoic Acid: Another polyunsaturated fatty acid with a similar structure but different double bond positions.
Uniqueness
(5Z,8Z,11S,12E,14Z)-11-hydroxyicosa-5,8,12,14-tetraenoic acid is unique due to its specific arrangement of double bonds and the presence of a hydroxyl group. This unique structure gives it distinct chemical and biological properties compared to other similar compounds.
生物活性
11(S)-Hydroxyeicosatetraenoic acid (11(S)-HETE) is a bioactive lipid derived from arachidonic acid (AA) through the action of various enzymes, primarily cytochrome P450 (CYP) and cyclooxygenases (COX). This compound has garnered attention due to its significant biological activities, including roles in cellular hypertrophy, inflammation, and cancer progression. This article reviews the biological activity of 11(S)-HETE, synthesizing findings from recent studies and case reports.
Biosynthesis of 11(S)-HETE
11(S)-HETE is predominantly formed via:
- Cyclooxygenase Pathway : COX-1 and COX-2 convert AA to 11-HpETE, which is then further reduced to 11(S)-HETE.
- Cytochrome P450 Pathway : CYP enzymes, particularly CYP1B1, catalyze the formation of 11(S)-HETE from AA. This pathway is critical in various tissues and has implications in cardiovascular health and disease.
Cellular Hypertrophy
Recent studies have demonstrated that both enantiomers of 11-HETE induce cellular hypertrophy in cardiomyocytes. A study using RL-14 human cardiomyocyte cells revealed that:
- Treatment with 20 µM of (R) or (S) 11-HETE for 24 hours resulted in significant increases in cell size and hypertrophic markers .
- (S)-11-HETE exhibited a more pronounced effect on the upregulation of CYP1B1 , suggesting an enantioselective mechanism of action .
Enantiomer | Effect on Cell Size | CYP1B1 mRNA Level | CYP1B1 Protein Level | Catalytic Activity |
---|---|---|---|---|
(R)-11-HETE | Moderate | Increased | Increased | No significant change |
(S)-11-HETE | Significant | Markedly increased | Markedly increased | Increased |
Inflammatory Responses
11(S)-HETE plays a role in mediating inflammatory responses. It has been implicated in:
- Eosinophilic Esophagitis (EoE) : Elevated levels of 15(S)-HETE, another hydroxyeicosatetraenoic acid, have been associated with EoE, indicating a potential role for HETEs in eosinophil recruitment and degranulation .
- Asthma : Similar pathways involving HETEs may contribute to asthma pathogenesis through Th2-mediated inflammation .
The biological effects of 11(S)-HETE are mediated through several signaling pathways:
- CYP Enzyme Activation : The compound enhances the expression and activity of various CYP enzymes, notably CYP1B1, which is linked to cardiovascular diseases.
- Cell Signaling Pathways : It activates pathways such as ERK1/2 and PI3K, which are crucial for cell proliferation and survival .
Case Studies
A notable case study involved the assessment of 11(S)-HETE's impact on human cardiomyocytes. Researchers found that:
特性
IUPAC Name |
(5Z,8Z,11S,12E,14Z)-11-hydroxyicosa-5,8,12,14-tetraenoic acid | |
---|---|---|
Source | PubChem | |
URL | https://pubchem.ncbi.nlm.nih.gov | |
Description | Data deposited in or computed by PubChem | |
InChI |
InChI=1S/C20H32O3/c1-2-3-4-5-7-10-13-16-19(21)17-14-11-8-6-9-12-15-18-20(22)23/h6-7,9-11,13-14,16,19,21H,2-5,8,12,15,17-18H2,1H3,(H,22,23)/b9-6-,10-7-,14-11-,16-13+/t19-/m1/s1 | |
Source | PubChem | |
URL | https://pubchem.ncbi.nlm.nih.gov | |
Description | Data deposited in or computed by PubChem | |
InChI Key |
GCZRCCHPLVMMJE-YZGNWCGPSA-N | |
Source | PubChem | |
URL | https://pubchem.ncbi.nlm.nih.gov | |
Description | Data deposited in or computed by PubChem | |
Canonical SMILES |
CCCCCC=CC=CC(CC=CCC=CCCCC(=O)O)O | |
Source | PubChem | |
URL | https://pubchem.ncbi.nlm.nih.gov | |
Description | Data deposited in or computed by PubChem | |
Isomeric SMILES |
CCCCC/C=C\C=C\[C@H](C/C=C\C/C=C\CCCC(=O)O)O | |
Source | PubChem | |
URL | https://pubchem.ncbi.nlm.nih.gov | |
Description | Data deposited in or computed by PubChem | |
Molecular Formula |
C20H32O3 | |
Source | PubChem | |
URL | https://pubchem.ncbi.nlm.nih.gov | |
Description | Data deposited in or computed by PubChem | |
Molecular Weight |
320.5 g/mol | |
Source | PubChem | |
URL | https://pubchem.ncbi.nlm.nih.gov | |
Description | Data deposited in or computed by PubChem | |
Retrosynthesis Analysis
AI-Powered Synthesis Planning: Our tool employs the Template_relevance Pistachio, Template_relevance Bkms_metabolic, Template_relevance Pistachio_ringbreaker, Template_relevance Reaxys, Template_relevance Reaxys_biocatalysis model, leveraging a vast database of chemical reactions to predict feasible synthetic routes.
One-Step Synthesis Focus: Specifically designed for one-step synthesis, it provides concise and direct routes for your target compounds, streamlining the synthesis process.
Accurate Predictions: Utilizing the extensive PISTACHIO, BKMS_METABOLIC, PISTACHIO_RINGBREAKER, REAXYS, REAXYS_BIOCATALYSIS database, our tool offers high-accuracy predictions, reflecting the latest in chemical research and data.
Strategy Settings
Precursor scoring | Relevance Heuristic |
---|---|
Min. plausibility | 0.01 |
Model | Template_relevance |
Template Set | Pistachio/Bkms_metabolic/Pistachio_ringbreaker/Reaxys/Reaxys_biocatalysis |
Top-N result to add to graph | 6 |
Feasible Synthetic Routes
試験管内研究製品の免責事項と情報
BenchChemで提示されるすべての記事および製品情報は、情報提供を目的としています。BenchChemで購入可能な製品は、生体外研究のために特別に設計されています。生体外研究は、ラテン語の "in glass" に由来し、生物体の外で行われる実験を指します。これらの製品は医薬品または薬として分類されておらず、FDAから任何の医療状態、病気、または疾患の予防、治療、または治癒のために承認されていません。これらの製品を人間または動物に体内に導入する形態は、法律により厳格に禁止されています。これらのガイドラインに従うことは、研究と実験において法的および倫理的な基準の遵守を確実にするために重要です。