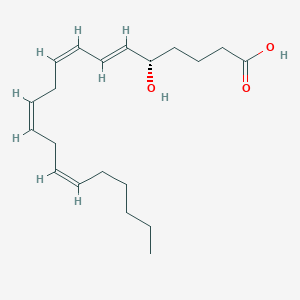
5(S)-Hete
概要
説明
Synthesis Analysis
The synthesis of 5(S)-HETE involves the oxygenation of arachidonic acid by 5-lipoxygenase, leading to the formation of this hydroxyeicosatetraenoic acid derivative. Innovative synthetic methods have been developed for the selective production of octadeuterated 5-HETE, which is crucial for GC-MS quantitation and studies on its biological functions and mechanisms (Hubbard, Phillips, & Taber, 1982).
Molecular Structure Analysis
The molecular structure of this compound is characterized by its hydroxy group at the 5th carbon of the eicosatetraenoic chain, contributing to its biological activity and interactions with cellular receptors and enzymes. Structural analyses, including X-ray diffraction, have been applied to related heterocyclic compounds to understand their arrangement and properties, providing insights into the behavior and function of this compound analogs (Takimiya, Konda, Ebata, Niihara, & Otsubo, 2005).
Chemical Reactions and Properties
This compound can undergo various chemical reactions, including further oxidation and conversion into other bioactive metabolites. Its reactivity is a subject of interest for synthesizing structurally and functionally diverse heterocycles, which are critical in medicinal chemistry and biological research. Studies on the synthesis of heterocyclic compounds provide a foundation for understanding the chemical behavior and potential applications of this compound and its derivatives (Gulevich, Dudnik, Chernyak, & Gevorgyan, 2013).
Physical Properties Analysis
The physical properties of this compound, including its solubility, melting point, and stability, are influenced by its molecular structure. Research on related heterocyclic compounds and their physical and chemical properties helps to elucidate the characteristics of this compound, facilitating its use in experimental and clinical settings (Martins, Frizzo, Moreira, Zanatta, & Bonacorso, 2008).
Chemical Properties Analysis
The chemical properties of this compound, including its reactivity and interactions with biological molecules, are pivotal for its role in cellular signaling and pathophysiological processes. Understanding these properties through the study of heterocyclic chemistry and the synthesis of related compounds offers insights into the mechanisms of action of this compound and its potential therapeutic applications (Isambert & Lavilla, 2008).
科学的研究の応用
Prostate Cancer Research : 5-HETE acts as a survival factor for human prostate cancer cells, suggesting a link between dietary fat and prostate cancer progression (Ghosh & Myers, 1998).
Immunological Studies : It stimulates human polymorphonuclear neutrophils to mobilize intracellular calcium, differing from mechanisms used by leukotriene B4 and platelet-activating factor (O’Flaherty, Jacobson, & Redman, 1988).
Analytical Applications : 5-HETE analysis is used for assessing the activation of the 5-lipoxygenase pathway in biological fluids like lung perfusates (Strife, Voelkel, & Murphy, 1987).
Obstetrics and Gynecology : It influences uterine contractility in vitro and is found in increased concentrations in human amniotic fluid at term, linking it to parturition processes (Romero et al., 1989).
Cellular Metabolism : 5(S)-HETE is esterified into phospholipids and triglycerides in stimulated neutrophils, potentially altering their membrane characteristics (Stenson & Parker, 1979).
Molecular Signaling : It acts via a down-regulatable, G protein-linked mechanism in human neutrophils (O’Flaherty & Rossi, 1993).
Biochemical Pathways : 5-HETE and 5-HEPE increase the nuclear translocation of Nrf2 and upregulate the expression of enzymes regulated by Nrf2 (Nagahora et al., 2017).
Neutrophil Function : It influences neutrophil function by elevating cytosolic Ca2+ and promoting protein kinase C mobilization (O’Flaherty & Nishihira, 1987).
Analytical Chemistry : It is an index of 5-lipoxygenation of arachidonic acid in biological fluids, detectable by GC-MS (Ogletree et al., 1982).
Infection and Allergic Responses : this compound plays key roles in bacterial infection and allergic responses (Clark et al., 2011).
特性
IUPAC Name |
(5S,6E,8Z,11Z,14Z)-5-hydroxyicosa-6,8,11,14-tetraenoic acid | |
---|---|---|
Source | PubChem | |
URL | https://pubchem.ncbi.nlm.nih.gov | |
Description | Data deposited in or computed by PubChem | |
InChI |
InChI=1S/C20H32O3/c1-2-3-4-5-6-7-8-9-10-11-12-13-14-16-19(21)17-15-18-20(22)23/h6-7,9-10,12-14,16,19,21H,2-5,8,11,15,17-18H2,1H3,(H,22,23)/b7-6-,10-9-,13-12-,16-14+/t19-/m1/s1 | |
Source | PubChem | |
URL | https://pubchem.ncbi.nlm.nih.gov | |
Description | Data deposited in or computed by PubChem | |
InChI Key |
KGIJOOYOSFUGPC-JGKLHWIESA-N | |
Source | PubChem | |
URL | https://pubchem.ncbi.nlm.nih.gov | |
Description | Data deposited in or computed by PubChem | |
Canonical SMILES |
CCCCCC=CCC=CCC=CC=CC(CCCC(=O)O)O | |
Source | PubChem | |
URL | https://pubchem.ncbi.nlm.nih.gov | |
Description | Data deposited in or computed by PubChem | |
Isomeric SMILES |
CCCCC/C=C\C/C=C\C/C=C\C=C\[C@H](CCCC(=O)O)O | |
Source | PubChem | |
URL | https://pubchem.ncbi.nlm.nih.gov | |
Description | Data deposited in or computed by PubChem | |
Molecular Formula |
C20H32O3 | |
Source | PubChem | |
URL | https://pubchem.ncbi.nlm.nih.gov | |
Description | Data deposited in or computed by PubChem | |
DSSTOX Substance ID |
DTXSID701017281 | |
Record name | 5-Hydroxy-6,8,11,14-eicosatetraenoic acid | |
Source | EPA DSSTox | |
URL | https://comptox.epa.gov/dashboard/DTXSID701017281 | |
Description | DSSTox provides a high quality public chemistry resource for supporting improved predictive toxicology. | |
Molecular Weight |
320.5 g/mol | |
Source | PubChem | |
URL | https://pubchem.ncbi.nlm.nih.gov | |
Description | Data deposited in or computed by PubChem | |
Physical Description |
Solid | |
Record name | 5-HETE | |
Source | Human Metabolome Database (HMDB) | |
URL | http://www.hmdb.ca/metabolites/HMDB0011134 | |
Description | The Human Metabolome Database (HMDB) is a freely available electronic database containing detailed information about small molecule metabolites found in the human body. | |
Explanation | HMDB is offered to the public as a freely available resource. Use and re-distribution of the data, in whole or in part, for commercial purposes requires explicit permission of the authors and explicit acknowledgment of the source material (HMDB) and the original publication (see the HMDB citing page). We ask that users who download significant portions of the database cite the HMDB paper in any resulting publications. | |
CAS RN |
330796-62-8, 70608-72-9 | |
Record name | 6,8,11,14-Eicosatetraenoic-d8 acid, 5-hydroxy-, (5S,6E,8Z,11Z,14Z)- | |
Source | CAS Common Chemistry | |
URL | https://commonchemistry.cas.org/detail?cas_rn=330796-62-8 | |
Description | CAS Common Chemistry is an open community resource for accessing chemical information. Nearly 500,000 chemical substances from CAS REGISTRY cover areas of community interest, including common and frequently regulated chemicals, and those relevant to high school and undergraduate chemistry classes. This chemical information, curated by our expert scientists, is provided in alignment with our mission as a division of the American Chemical Society. | |
Explanation | The data from CAS Common Chemistry is provided under a CC-BY-NC 4.0 license, unless otherwise stated. | |
Record name | 5-Hydroxyeicosatetraenoic acid | |
Source | CAS Common Chemistry | |
URL | https://commonchemistry.cas.org/detail?cas_rn=70608-72-9 | |
Description | CAS Common Chemistry is an open community resource for accessing chemical information. Nearly 500,000 chemical substances from CAS REGISTRY cover areas of community interest, including common and frequently regulated chemicals, and those relevant to high school and undergraduate chemistry classes. This chemical information, curated by our expert scientists, is provided in alignment with our mission as a division of the American Chemical Society. | |
Explanation | The data from CAS Common Chemistry is provided under a CC-BY-NC 4.0 license, unless otherwise stated. | |
Record name | 5-HETE | |
Source | ChemIDplus | |
URL | https://pubchem.ncbi.nlm.nih.gov/substance/?source=chemidplus&sourceid=0070608729 | |
Description | ChemIDplus is a free, web search system that provides access to the structure and nomenclature authority files used for the identification of chemical substances cited in National Library of Medicine (NLM) databases, including the TOXNET system. | |
Record name | 5-Hydroxy-6,8,11,14-eicosatetraenoic acid | |
Source | EPA DSSTox | |
URL | https://comptox.epa.gov/dashboard/DTXSID701017281 | |
Description | DSSTox provides a high quality public chemistry resource for supporting improved predictive toxicology. | |
Record name | (+)-5(S)-Hydroxy-(6E,8Z,11Z,14Z)-eicosatetraenoic acid | |
Source | European Chemicals Agency (ECHA) | |
URL | https://echa.europa.eu/information-on-chemicals | |
Description | The European Chemicals Agency (ECHA) is an agency of the European Union which is the driving force among regulatory authorities in implementing the EU's groundbreaking chemicals legislation for the benefit of human health and the environment as well as for innovation and competitiveness. | |
Explanation | Use of the information, documents and data from the ECHA website is subject to the terms and conditions of this Legal Notice, and subject to other binding limitations provided for under applicable law, the information, documents and data made available on the ECHA website may be reproduced, distributed and/or used, totally or in part, for non-commercial purposes provided that ECHA is acknowledged as the source: "Source: European Chemicals Agency, http://echa.europa.eu/". Such acknowledgement must be included in each copy of the material. ECHA permits and encourages organisations and individuals to create links to the ECHA website under the following cumulative conditions: Links can only be made to webpages that provide a link to the Legal Notice page. | |
Record name | 5-HETE | |
Source | FDA Global Substance Registration System (GSRS) | |
URL | https://gsrs.ncats.nih.gov/ginas/app/beta/substances/467RNW8T91 | |
Description | The FDA Global Substance Registration System (GSRS) enables the efficient and accurate exchange of information on what substances are in regulated products. Instead of relying on names, which vary across regulatory domains, countries, and regions, the GSRS knowledge base makes it possible for substances to be defined by standardized, scientific descriptions. | |
Explanation | Unless otherwise noted, the contents of the FDA website (www.fda.gov), both text and graphics, are not copyrighted. They are in the public domain and may be republished, reprinted and otherwise used freely by anyone without the need to obtain permission from FDA. Credit to the U.S. Food and Drug Administration as the source is appreciated but not required. | |
Record name | 5-HETE | |
Source | Human Metabolome Database (HMDB) | |
URL | http://www.hmdb.ca/metabolites/HMDB0011134 | |
Description | The Human Metabolome Database (HMDB) is a freely available electronic database containing detailed information about small molecule metabolites found in the human body. | |
Explanation | HMDB is offered to the public as a freely available resource. Use and re-distribution of the data, in whole or in part, for commercial purposes requires explicit permission of the authors and explicit acknowledgment of the source material (HMDB) and the original publication (see the HMDB citing page). We ask that users who download significant portions of the database cite the HMDB paper in any resulting publications. | |
Synthesis routes and methods I
Procedure details
Synthesis routes and methods II
Procedure details
Synthesis routes and methods III
Procedure details
Synthesis routes and methods IV
Procedure details
Synthesis routes and methods V
Procedure details
Retrosynthesis Analysis
AI-Powered Synthesis Planning: Our tool employs the Template_relevance Pistachio, Template_relevance Bkms_metabolic, Template_relevance Pistachio_ringbreaker, Template_relevance Reaxys, Template_relevance Reaxys_biocatalysis model, leveraging a vast database of chemical reactions to predict feasible synthetic routes.
One-Step Synthesis Focus: Specifically designed for one-step synthesis, it provides concise and direct routes for your target compounds, streamlining the synthesis process.
Accurate Predictions: Utilizing the extensive PISTACHIO, BKMS_METABOLIC, PISTACHIO_RINGBREAKER, REAXYS, REAXYS_BIOCATALYSIS database, our tool offers high-accuracy predictions, reflecting the latest in chemical research and data.
Strategy Settings
Precursor scoring | Relevance Heuristic |
---|---|
Min. plausibility | 0.01 |
Model | Template_relevance |
Template Set | Pistachio/Bkms_metabolic/Pistachio_ringbreaker/Reaxys/Reaxys_biocatalysis |
Top-N result to add to graph | 6 |
Feasible Synthetic Routes
試験管内研究製品の免責事項と情報
BenchChemで提示されるすべての記事および製品情報は、情報提供を目的としています。BenchChemで購入可能な製品は、生体外研究のために特別に設計されています。生体外研究は、ラテン語の "in glass" に由来し、生物体の外で行われる実験を指します。これらの製品は医薬品または薬として分類されておらず、FDAから任何の医療状態、病気、または疾患の予防、治療、または治癒のために承認されていません。これらの製品を人間または動物に体内に導入する形態は、法律により厳格に禁止されています。これらのガイドラインに従うことは、研究と実験において法的および倫理的な基準の遵守を確実にするために重要です。