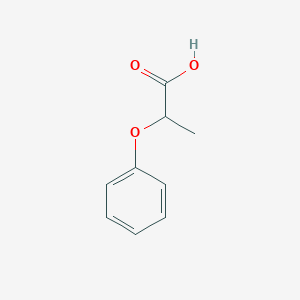
2-フェノキシプロピオン酸
概要
説明
2-Phenoxypropionic acid is an organic compound with the molecular formula C9H10O3. It is a white to almost white crystalline powder that is soluble in organic solvents like ethanol, chloroform, and ether, but only slightly soluble in water . This compound is known for its applications in various fields, including chemistry, biology, and industry.
科学的研究の応用
2-Phenoxypropionic acid has diverse applications in scientific research:
Chemistry: It is used as an intermediate in the synthesis of herbicides and other agrochemicals.
Biology: It is studied for its potential effects on biological pathways and as a tool in biochemical assays.
Medicine: It is explored for its potential therapeutic properties and as a precursor in drug synthesis.
Industry: It is used in the production of polymers, resins, and coatings.
作用機序
Target of Action
The primary target of 2-Phenoxypropionic acid is the human sweet taste receptor . This receptor is a protein that is responsible for the perception of sweetness in the human body. The compound interacts with the transmembrane domain of the T1R3 subunit (T1R3-TMD) of the receptor .
Mode of Action
2-Phenoxypropionic acid, an inhibitor of the human sweet taste receptor, has been shown to interact with the transmembrane domain of the T1R3 subunit of the receptor . The compound’s interaction with its targets results in the inhibition of the sweet taste receptor, thereby affecting the perception of sweetness.
Biochemical Pathways
It is known that the compound affects the signaling pathway of the sweet taste receptor by inhibiting its function . This could potentially affect downstream effects related to the perception of sweetness.
Result of Action
The primary result of the action of 2-Phenoxypropionic acid is the inhibition of the human sweet taste receptor . This leads to a decrease in the perception of sweetness when the compound is present. This effect could potentially be used in the development of treatments for conditions related to the overconsumption of sweet foods, such as obesity and type 2 diabetes.
生化学分析
Biochemical Properties
2-Phenoxypropionic acid has been found to interact with certain enzymes and proteins. For instance, it has been reported that the esterification of 2-Phenoxypropionic acid is catalyzed by Candida cylindracea lipase This interaction suggests that 2-Phenoxypropionic acid may play a role in lipid metabolism
Cellular Effects
It is known that derivatives of 2-Phenoxypropionic acid have potential as herbicides This suggests that 2-Phenoxypropionic acid may have an impact on cellular processes in certain types of cells, particularly plant cells
Molecular Mechanism
It is known that the compound interacts with certain enzymes, such as Candida cylindracea lipase
Metabolic Pathways
It is known that the compound interacts with certain enzymes , suggesting that it may be involved in specific metabolic pathways
準備方法
Synthetic Routes and Reaction Conditions: 2-Phenoxypropionic acid can be synthesized through several methods. One common method involves the reaction of phenol with 2-chloropropionic acid in the presence of a base, such as sodium hydroxide, to form the desired product . Another method includes the etherification of S-2-chloropropionic acid with phenol, followed by purification using anionic exchange resin .
Industrial Production Methods: In industrial settings, the production of 2-Phenoxypropionic acid often involves the use of large-scale reactors and optimized reaction conditions to ensure high yield and purity. The process typically includes steps such as diazotization, chlorination, and etherification, followed by purification and crystallization .
化学反応の分析
Types of Reactions: 2-Phenoxypropionic acid undergoes various chemical reactions, including:
Oxidation: It can be oxidized to form quinone-type compounds.
Reduction: It can be reduced to form alcohol derivatives.
Substitution: It can undergo nucleophilic substitution reactions to form various derivatives.
Common Reagents and Conditions:
Oxidation: Potassium dichromate in acidic conditions.
Reduction: Sodium borohydride or lithium aluminum hydride.
Substitution: Alkyl halides or acyl chlorides in the presence of a base.
Major Products:
Oxidation: Quinone-type compounds.
Reduction: Alcohol derivatives.
Substitution: Various substituted phenoxypropionic acids.
類似化合物との比較
- 2-Phenylpropionic acid
- 3-Phenoxypropionic acid
- 2-(4-Hydroxyphenoxy)propionic acid
Comparison: 2-Phenoxypropionic acid is unique due to its specific interactions with biological targets and its diverse applications. Compared to 2-Phenylpropionic acid and 3-Phenoxypropionic acid, it has a distinct phenoxy group that contributes to its unique chemical properties and reactivity. Additionally, 2-(4-Hydroxyphenoxy)propionic acid is a hydroxylated derivative that is often used as an intermediate in the synthesis of herbicides .
生物活性
2-Phenoxypropionic acid (PPA) is an organic compound with significant biological activity, particularly in agricultural and pharmaceutical applications. This article explores its biological properties, mechanisms of action, and implications in various fields, supported by data tables and research findings.
Chemical Structure and Properties
2-Phenoxypropionic acid is characterized by its phenoxy group attached to a propionic acid moiety. Its chemical formula is , with a melting point of 112-115 °C and a boiling point of 265 °C . The compound exhibits different conformations based on hydrogen-bonding motifs, which influence its crystallization behavior and biological interactions .
Biological Activity Overview
The biological activities of 2-phenoxypropionic acid can be categorized into several key areas:
1. Herbicidal Activity
- PPA derivatives are recognized as potential herbicides due to their selectivity against grassy weeds. The synthesis of R-2-(4-hydroxyphenoxy)propionic acid (R-HPPA), an important intermediate for herbicides, has been optimized for better efficacy .
2. Metabolic Effects in Livestock
- A study investigated the impact of 2-methyl-2-phenoxypropionic acid (MFPA), a derivative of PPA, on dairy cows. The administration of MFPA was evaluated for its effects on serum lipid profiles and ovarian activity. Results indicated no significant changes in serum triglycerides or cholesterol levels post-administration, suggesting that while MFPA activates energy metabolism, it does not adversely affect lipid profiles or reproductive performance in dairy cows .
The mechanisms through which PPA exerts its biological effects include:
- Peroxisome Proliferator-Activated Receptors (PPARs) Activation : PPA acts as an agonist for PPARα and PPARγ, influencing lipid metabolism by enhancing fatty acid oxidation and reducing triglyceride levels in the liver .
- Antioxidant Properties : Research indicates that PPA may possess antioxidant properties, contributing to cellular protection against oxidative stress .
Case Study 1: Effect on Dairy Cow Metabolism
A study involving 57 multiparous Holstein cows assessed the effects of MFPA on metabolic disorders during the transition period. Blood samples were collected at various intervals to monitor serum lipid profiles and progesterone levels. The findings revealed that while MFPA administration did not significantly alter lipid concentrations, it did correlate with increased postpartum diseases in certain groups, highlighting the need for careful consideration in metabolic management strategies .
Group | Treatment | Serum BHB Levels | Postpartum Diseases |
---|---|---|---|
1 | MFPA | Increased | 8 cases |
2 | Placebo | Stable | 3 cases |
Case Study 2: Herbicide Development
Research focused on the synthesis of R-HPPA demonstrated an efficient biotransformative route for producing this herbicide intermediate. The study highlighted the importance of enzymatic processes in achieving high selectivity and yield, showcasing the potential agricultural applications of PPA derivatives .
特性
IUPAC Name |
2-phenoxypropanoic acid | |
---|---|---|
Source | PubChem | |
URL | https://pubchem.ncbi.nlm.nih.gov | |
Description | Data deposited in or computed by PubChem | |
InChI |
InChI=1S/C9H10O3/c1-7(9(10)11)12-8-5-3-2-4-6-8/h2-7H,1H3,(H,10,11) | |
Source | PubChem | |
URL | https://pubchem.ncbi.nlm.nih.gov | |
Description | Data deposited in or computed by PubChem | |
InChI Key |
SXERGJJQSKIUIC-UHFFFAOYSA-N | |
Source | PubChem | |
URL | https://pubchem.ncbi.nlm.nih.gov | |
Description | Data deposited in or computed by PubChem | |
Canonical SMILES |
CC(C(=O)O)OC1=CC=CC=C1 | |
Source | PubChem | |
URL | https://pubchem.ncbi.nlm.nih.gov | |
Description | Data deposited in or computed by PubChem | |
Molecular Formula |
C9H10O3 | |
Source | PubChem | |
URL | https://pubchem.ncbi.nlm.nih.gov | |
Description | Data deposited in or computed by PubChem | |
DSSTOX Substance ID |
DTXSID10870812 | |
Record name | Propanoic acid, 2-phenoxy- | |
Source | EPA DSSTox | |
URL | https://comptox.epa.gov/dashboard/DTXSID10870812 | |
Description | DSSTox provides a high quality public chemistry resource for supporting improved predictive toxicology. | |
Molecular Weight |
166.17 g/mol | |
Source | PubChem | |
URL | https://pubchem.ncbi.nlm.nih.gov | |
Description | Data deposited in or computed by PubChem | |
CAS No. |
940-31-8 | |
Record name | DL-2-Phenoxypropionic acid | |
Source | CAS Common Chemistry | |
URL | https://commonchemistry.cas.org/detail?cas_rn=940-31-8 | |
Description | CAS Common Chemistry is an open community resource for accessing chemical information. Nearly 500,000 chemical substances from CAS REGISTRY cover areas of community interest, including common and frequently regulated chemicals, and those relevant to high school and undergraduate chemistry classes. This chemical information, curated by our expert scientists, is provided in alignment with our mission as a division of the American Chemical Society. | |
Explanation | The data from CAS Common Chemistry is provided under a CC-BY-NC 4.0 license, unless otherwise stated. | |
Record name | 2-Phenoxypropionic acid | |
Source | ChemIDplus | |
URL | https://pubchem.ncbi.nlm.nih.gov/substance/?source=chemidplus&sourceid=0000940318 | |
Description | ChemIDplus is a free, web search system that provides access to the structure and nomenclature authority files used for the identification of chemical substances cited in National Library of Medicine (NLM) databases, including the TOXNET system. | |
Record name | 2-Phenoxypropionic acid | |
Source | DTP/NCI | |
URL | https://dtp.cancer.gov/dtpstandard/servlet/dwindex?searchtype=NSC&outputformat=html&searchlist=404102 | |
Description | The NCI Development Therapeutics Program (DTP) provides services and resources to the academic and private-sector research communities worldwide to facilitate the discovery and development of new cancer therapeutic agents. | |
Explanation | Unless otherwise indicated, all text within NCI products is free of copyright and may be reused without our permission. Credit the National Cancer Institute as the source. | |
Record name | 2-Phenoxypropionic acid | |
Source | DTP/NCI | |
URL | https://dtp.cancer.gov/dtpstandard/servlet/dwindex?searchtype=NSC&outputformat=html&searchlist=1866 | |
Description | The NCI Development Therapeutics Program (DTP) provides services and resources to the academic and private-sector research communities worldwide to facilitate the discovery and development of new cancer therapeutic agents. | |
Explanation | Unless otherwise indicated, all text within NCI products is free of copyright and may be reused without our permission. Credit the National Cancer Institute as the source. | |
Record name | Propanoic acid, 2-phenoxy- | |
Source | EPA Chemicals under the TSCA | |
URL | https://www.epa.gov/chemicals-under-tsca | |
Description | EPA Chemicals under the Toxic Substances Control Act (TSCA) collection contains information on chemicals and their regulations under TSCA, including non-confidential content from the TSCA Chemical Substance Inventory and Chemical Data Reporting. | |
Record name | Propanoic acid, 2-phenoxy- | |
Source | EPA DSSTox | |
URL | https://comptox.epa.gov/dashboard/DTXSID10870812 | |
Description | DSSTox provides a high quality public chemistry resource for supporting improved predictive toxicology. | |
Record name | 2-phenoxypropionic acid | |
Source | European Chemicals Agency (ECHA) | |
URL | https://echa.europa.eu/substance-information/-/substanceinfo/100.012.154 | |
Description | The European Chemicals Agency (ECHA) is an agency of the European Union which is the driving force among regulatory authorities in implementing the EU's groundbreaking chemicals legislation for the benefit of human health and the environment as well as for innovation and competitiveness. | |
Explanation | Use of the information, documents and data from the ECHA website is subject to the terms and conditions of this Legal Notice, and subject to other binding limitations provided for under applicable law, the information, documents and data made available on the ECHA website may be reproduced, distributed and/or used, totally or in part, for non-commercial purposes provided that ECHA is acknowledged as the source: "Source: European Chemicals Agency, http://echa.europa.eu/". Such acknowledgement must be included in each copy of the material. ECHA permits and encourages organisations and individuals to create links to the ECHA website under the following cumulative conditions: Links can only be made to webpages that provide a link to the Legal Notice page. | |
Synthesis routes and methods
Procedure details
Retrosynthesis Analysis
AI-Powered Synthesis Planning: Our tool employs the Template_relevance Pistachio, Template_relevance Bkms_metabolic, Template_relevance Pistachio_ringbreaker, Template_relevance Reaxys, Template_relevance Reaxys_biocatalysis model, leveraging a vast database of chemical reactions to predict feasible synthetic routes.
One-Step Synthesis Focus: Specifically designed for one-step synthesis, it provides concise and direct routes for your target compounds, streamlining the synthesis process.
Accurate Predictions: Utilizing the extensive PISTACHIO, BKMS_METABOLIC, PISTACHIO_RINGBREAKER, REAXYS, REAXYS_BIOCATALYSIS database, our tool offers high-accuracy predictions, reflecting the latest in chemical research and data.
Strategy Settings
Precursor scoring | Relevance Heuristic |
---|---|
Min. plausibility | 0.01 |
Model | Template_relevance |
Template Set | Pistachio/Bkms_metabolic/Pistachio_ringbreaker/Reaxys/Reaxys_biocatalysis |
Top-N result to add to graph | 6 |
Feasible Synthetic Routes
試験管内研究製品の免責事項と情報
BenchChemで提示されるすべての記事および製品情報は、情報提供を目的としています。BenchChemで購入可能な製品は、生体外研究のために特別に設計されています。生体外研究は、ラテン語の "in glass" に由来し、生物体の外で行われる実験を指します。これらの製品は医薬品または薬として分類されておらず、FDAから任何の医療状態、病気、または疾患の予防、治療、または治癒のために承認されていません。これらの製品を人間または動物に体内に導入する形態は、法律により厳格に禁止されています。これらのガイドラインに従うことは、研究と実験において法的および倫理的な基準の遵守を確実にするために重要です。