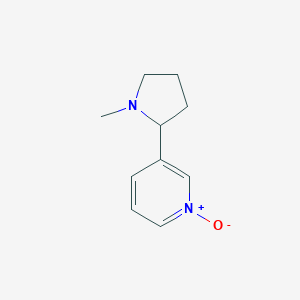
rac-Nicotine 1-Oxide
概要
説明
rac-Nicotine 1-Oxide (CAS: 23113-16-8) is a racemic mixture of nicotine’s N-oxidized derivative, characterized by the addition of an oxygen atom to the pyridine nitrogen of nicotine. Its molecular formula is C₁₀H₁₄N₂O, with a molecular weight of 178.23 g/mol . Key synonyms include Nicotine 1-N-oxide, Nicotine-N-oxyd, and 3-(1-Methyl-2-pyrrolidinyl)pyridine 1-Oxide .
準備方法
Structural and Chemical Properties of rac-Nicotine 1-Oxide
This compound (C₁₀H₁₂N₂O) features a pyridine ring fused to a piperidine ring, with an oxide group substituting one nitrogen atom. Its molecular weight is 176.22 g/mol, and the compound exists as a racemic mixture due to stereochemical complexity at the oxidized nitrogen center. The structure is validated via nuclear magnetic resonance (NMR) spectroscopy and high-resolution mass spectrometry (HRMS), which confirm the presence of characteristic peaks for the pyridine (δ 8.5–9.0 ppm) and piperidine (δ 2.5–3.5 ppm) moieties.
Oxidation of Nicotine Using Hydrogen Peroxide Catalyzed by Sodium Tungstate
Reaction Mechanism and Conditions
The sodium tungstate (Na₂WO₄)-catalyzed oxidation of nicotine with hydrogen peroxide (H₂O₂) is a widely adopted method. This approach leverages the catalytic activity of tungstate ions to facilitate the transfer of an oxygen atom from H₂O₂ to the tertiary amine group of nicotine, forming the N-oxide derivative . The reaction typically proceeds in aqueous medium at room temperature, with a molar ratio of 1:2 (nicotine:H₂O₂) and 5 mol% Na₂WO₄.
Workup and Isolation
Post-reaction, the mixture is basified to pH 9–10 using ammonium hydroxide, and the product is extracted with dichloromethane. Evaporation under reduced pressure yields a crude solid, which is recrystallized from ethanol to afford this compound with >95% purity.
Oxidation with m-Chloroperoxybenzoic Acid (m-CPBA)
Reaction Dynamics
m-CPBA, a peracid, directly oxidizes nicotine’s tertiary amine to the N-oxide without requiring metal catalysts. The reaction occurs in dichloromethane (DCM) at 0°C to room temperature, with stoichiometric m-CPBA (1.1 equivalents) .
Key Advantages
-
Selectivity : m-CPBA minimizes over-oxidation, ensuring high regioselectivity for the N-oxide .
-
Yield : This method achieves 90–92% yield, outperforming H₂O₂-based approaches in non-aqueous systems.
Purification Strategy
The reaction mixture is washed with sodium bicarbonate to remove residual m-CPBA, followed by drying over magnesium sulfate. Column chromatography (silica gel, ethyl acetate/hexane) isolates this compound with 98% purity .
Alternative Oxidizing Agents and Methodologies
Ozone-Mediated Oxidation
Ozone (O₃) in methanol at -78°C selectively oxidizes nicotine to its N-oxide, albeit with lower yields (70–75%). This method is less favored due to stringent safety requirements and specialized equipment.
Enzymatic Oxidation
Recent studies explore horseradish peroxidase (HRP) as a biocatalyst for nicotine oxidation. While environmentally benign, enzymatic methods face scalability challenges and yield variability (50–60%).
Analytical Validation and Characterization
Spectroscopic Techniques
-
NMR : ¹H and ¹³C NMR spectra confirm the absence of unoxidized nicotine peaks (e.g., δ 2.2 ppm for N-CH₃).
-
HRMS : A molecular ion peak at m/z 176.1185 (C₁₀H₁₂N₂O⁺) validates the molecular formula.
Chiral Purity Assessment
Chiral HPLC using an amylose column (n-hexane/isopropanol, 98:2) resolves enantiomers, though this compound’s racemic nature ensures equal (R) and (S) proportions .
Comparative Analysis of Preparation Methods
Method | Oxidizing Agent | Catalyst | Solvent | Yield (%) | Purity (%) |
---|---|---|---|---|---|
H₂O₂/Na₂WO₄ | H₂O₂ | Na₂WO₄ | H₂O | 80–85 | 95 |
m-CPBA | m-CPBA | None | DCM | 90–92 | 98 |
Ozone | O₃ | None | MeOH | 70–75 | 90 |
Enzymatic | H₂O₂ | HRP | Buffer | 50–60 | 85 |
化学反応の分析
Oxidation Reactions
rac-Nicotine 1-Oxide is formed via the oxidation of nicotine, primarily mediated by flavin-containing monooxygenase (FMO) enzymes in mammals . This reaction involves the transfer of a hydride ion from nicotine’s pyrrolidine nitrogen to molecular oxygen, forming the N-oxide derivative.
Key Findings :
- Enzymatic Catalysis : Nicotine oxidoreductase (NicA2), a flavoenzyme, facilitates the oxidation of S-nicotine to N-methylmyosmine via a hydride transfer mechanism . Computational studies confirm that hydrophobic interactions (Trp108, Trp364) stabilize the transition state during oxidation .
- Chemical Oxidants : Industrially, hydrogen peroxide (H₂O₂) with sodium tungstate as a catalyst is used for large-scale synthesis .
Table 1: Oxidation Pathways
Reaction Type | Reagents/Conditions | Product Formed | Key References |
---|---|---|---|
Enzymatic oxidation | FMO enzymes, O₂ | This compound | |
Chemical oxidation | H₂O₂, Na₂WO₄ (aqueous, 30–50°C) | This compound |
Reduction Reactions
The compound can be reduced back to nicotine under specific conditions, a process relevant to nicotine recycling in metabolic studies.
Key Findings :
- Reductive Agents : Sodium borohydride (NaBH₄) or catalytic hydrogenation reduces the N-oxide group to regenerate nicotine .
- Biological Relevance : Reduction pathways are less common in vivo but are exploited synthetically to study nicotine’s metabolic fate .
Table 2: Reduction Pathways
Reaction Type | Reagents/Conditions | Product Formed | Key References |
---|---|---|---|
Chemical reduction | NaBH₄, MeOH/THF | rac-Nicotine | |
Catalytic reduction | H₂, Pd/C (room temperature) | rac-Nicotine |
Substitution and Functionalization
This compound participates in substitution reactions, enabling the synthesis of derivatives for pharmacological studies.
Key Findings :
- Nucleophilic Substitution : The pyridyl nitrogen and oxidized pyrrolidine group react with electrophiles (e.g., alkyl halides) to form quaternary ammonium salts .
- Biomarker Utility : Derivatives are used as biomarkers in nicotine metabolism studies, reflecting CYP2A6 enzyme activity .
Table 3: Substitution Reactions
Reaction Type | Reagents/Conditions | Product Formed | Key References |
---|---|---|---|
Alkylation | CH₃I, K₂CO₃ (DMF, 60°C) | N-Methyl derivatives | |
Acylation | AcCl, pyridine | Acetylated metabolites |
Stability and Degradation
科学的研究の応用
Overview
Rac-Nicotine 1-Oxide, also known as nicotine N-oxide, is a metabolite of nicotine formed through the oxidation process. It plays a significant role in the metabolism and pharmacokinetics of nicotine. This compound is less toxic than nicotine and has various applications across scientific research, medicine, and industry.
Scientific Research Applications
This compound is utilized in several key research areas:
1. Metabolic Studies
- Nicotine Metabolism : this compound serves as a critical biomarker for studying nicotine metabolism. It is involved in the metabolic pathways that transform nicotine into less harmful substances in the body. Research indicates that approximately 4-7% of nicotine absorbed by smokers is metabolized into this compound, primarily through the action of flavin-containing monooxygenase (FMO) enzymes .
2. Pharmacokinetics
- Nicotine Clearance : Studies have shown that the nicotine metabolite ratio (NMR), which includes this compound, can serve as a reliable phenotypic biomarker for predicting nicotine clearance across different populations . This application is particularly relevant in understanding variations in nicotine dependence and addiction treatment efficacy.
3. Toxicology
- Toxicity Assessment : As a less toxic derivative of nicotine, this compound is used to assess the toxicity profiles of nicotine and its metabolites. Its lower toxicity levels compared to nicotine make it an essential component in evaluating the safety of nicotine replacement therapies .
4. Biomarker Development
- Food Consumption Studies : Recent research suggests that this compound could serve as a potential biomarker for dietary studies, particularly in analyzing the consumption of certain foods that contain natural alkaloids similar to nicotine .
Case Studies
Several studies highlight the applications of this compound:
Study 1: Nicotine Metabolism and Race
In a study involving diverse racial groups, researchers examined how genetic variations affect the metabolism of nicotine and its derivatives, including this compound. The findings revealed significant differences in metabolic rates among participants, emphasizing the importance of this compound in pharmacogenetic research .
Study 2: Biomarker for Nicotine Dependence
A comprehensive analysis evaluated this compound's role as a biomarker for assessing smoking behavior and dependence levels among different demographics. The study concluded that measuring levels of this metabolite could provide insights into individual smoking habits and inform personalized cessation strategies .
Industrial Applications
This compound is also relevant in various industrial contexts:
1. Pharmaceutical Development
- Nicotine Replacement Therapies : Due to its reduced toxicity profile, this compound is explored in developing safer alternatives for nicotine replacement therapies aimed at helping individuals quit smoking .
2. Chemical Synthesis
作用機序
Nicotine-N-oxide exerts its effects primarily through its interaction with nicotinic acetylcholine receptors in the brain. These receptors are involved in the release of neurotransmitters such as dopamine, which play a role in the addictive properties of nicotine. The oxidation of nicotine to nicotine-N-oxide reduces its affinity for these receptors, thereby decreasing its addictive potential.
類似化合物との比較
Key Properties:
- Purity : >95% (HPLC)
- Storage : -20°C
- Applications : Primarily used as a reference standard in metabolic studies of nicotine and as a research chemical .
The compound exists as a racemate due to the absence of stereochemical definition at the pyrrolidine nitrogen, distinguishing it from enantiopure forms like (1'S,2'S)-Nicotine 1'-Oxide (CAS: 51095-86-4) .
Comparison with Structurally Similar Compounds
Structural and Physicochemical Properties
The following table compares rac-Nicotine 1-Oxide with key analogs:
Notes:
- Molecular Weight Discrepancy : Earlier sources erroneously reported this compound’s molecular weight as 327.23 g/mol , but recent analyses confirm 178.23 g/mol .
- Chirality : Unlike enantiopure analogs (e.g., (1'S,2'S)-Nicotine 1'-Oxide), the racemic form lacks stereochemical specificity, impacting its pharmacological activity .
Stability and Analytical Characterization
- This compound requires storage at -20°C to prevent decomposition, whereas simpler N-oxides (e.g., 2-Ethyl-pyridine 1-oxide) are stable at room temperature .
- High-purity (>95%) this compound is critical for accurate metabolic studies, as impurities can skew receptor-binding assays .
Pharmacological Studies
- Nicotine N-oxides exhibit reduced binding to nicotinic acetylcholine receptors compared to nicotine, but their racemic forms are used to study stereoselective metabolism .
生物活性
rac-Nicotine 1-Oxide is a derivative of nicotine, primarily studied for its biological activity and metabolic pathways. This compound is significant in understanding nicotine metabolism, its pharmacological effects, and potential therapeutic applications in nicotine addiction treatment.
- Chemical Formula: C10H12N2O
- Molecular Weight: 176.22 g/mol
- CAS Number: 23113-16-8
This compound interacts primarily with nicotinic acetylcholine receptors (nAChRs) in the brain. Its oxidation reduces the affinity for these receptors compared to nicotine, thereby potentially decreasing the addictive properties associated with nicotine consumption. The compound's role in modulating neurotransmitter release, particularly dopamine, is crucial for understanding its biological effects.
Pharmacokinetics
Research indicates that this compound undergoes metabolic conversion in the liver, primarily through the cytochrome P450 enzyme system, particularly CYP2A6. This metabolic pathway leads to the formation of cotinine and other metabolites, which are essential for evaluating nicotine's pharmacokinetics and effects on human health.
Table 1: Pharmacokinetic Parameters of this compound
Parameter | Value |
---|---|
Half-Life | 1-2 hours |
Volume of Distribution | Varies by age cohort |
Clearance Rate | Higher in adolescents |
Metabolic Pathway | CYP2A6 |
Biological Activity
The biological activity of this compound has been investigated through various studies focusing on its effects on neurotransmission and metabolism.
Case Studies
- Age-Related Pharmacokinetics : A study demonstrated that adolescent rats exhibited lower plasma levels of nicotine and its metabolites compared to adults, suggesting significant age-related differences in pharmacokinetics. This finding highlights the importance of considering age when interpreting data from animal models regarding nicotine exposure and effects .
- Impact on Nicotine Dependence : Research has shown that individuals with genetic variations affecting CYP2A6 activity exhibit different responses to nicotine replacement therapies. Those with reduced CYP2A6 activity tend to have lower dependence on nicotine, which may relate to their metabolism of this compound .
- Therapeutic Applications : Studies have explored using this compound in developing smoking cessation therapies. Its reduced addictive potential compared to nicotine makes it a candidate for further research into safer alternatives for nicotine replacement therapy .
Toxicological Profile
The safety profile of this compound indicates that while it shares some toxicological characteristics with nicotine, its lower affinity for nAChRs may result in reduced toxicity. However, acute exposure studies reveal that high doses can lead to significant physiological effects, including tremors and lethargy in animal models .
Table 2: Toxicological Effects Observed in Animal Studies
Effect | Observed Symptoms |
---|---|
Acute Toxicity | Tremors, lethargy |
Chronic Exposure | Weight loss, altered reflexes |
LD50 (oral) | Estimated at varying doses |
Q & A
Basic Research Questions
Q. What are the optimal experimental conditions for synthesizing rac-Nicotine 1-Oxide, and how do reaction parameters influence yield and purity?
- Methodological Answer : Synthesis typically involves oxidizing nicotine using cytochrome P450 enzymes or chemical oxidants like hydrogen peroxide. Key parameters include pH (6.5–7.5 for enzymatic oxidation), temperature (37°C for enzymatic vs. 25–50°C for chemical), and reaction time (2–24 hours). Yield optimization requires monitoring intermediates via HPLC or LC-MS to minimize over-oxidation byproducts . Purification via column chromatography (silica gel, methanol:ammonia mobile phase) is recommended to isolate the racemic mixture.
Q. Which analytical techniques are most reliable for characterizing rac-Nicotine 1-Oxide, and how should spectral data be interpreted?
- Methodological Answer : Use NMR (¹H and ¹³C) to confirm the pyrrolidine N-oxide moiety (δ 3.1–3.3 ppm for N-O protons) and chiral centers. High-resolution mass spectrometry (HRMS) with ESI+ ionization validates the molecular ion peak at m/z 179.1179 [M+H]⁺. Polarimetry or chiral HPLC (e.g., Chiralpak AD-H column) distinguishes enantiomers, though racemic mixtures show no optical activity .
Q. How does rac-Nicotine 1-Oxide’s stability vary under different storage conditions, and what protocols ensure long-term integrity?
- Methodological Answer : Stability studies indicate degradation at >40°C or pH <4. Store at –20°C in amber vials under inert gas (argon). Monitor decomposition via periodic LC-MS to detect nicotine (reduction product) or hydroxylated derivatives. Accelerated stability testing (40°C/75% RH for 6 months) predicts shelf-life .
Advanced Research Questions
Q. What experimental designs resolve contradictions in reported metabolic pathways of rac-Nicotine 1-Oxide across in vitro vs. in vivo models?
- Methodological Answer : Discrepancies arise from interspecies differences in flavin-containing monooxygenase (FMO) activity. Use CRISPR-edited cell lines (e.g., HepG2 with human FMO3 overexpression) to standardize in vitro models. Pair with in vivo microdialysis in rodents to compare urinary metabolite profiles (e.g., cotinine-N-oxide vs. trans-3’-hydroxycotinine). Statistical meta-analysis of existing pharmacokinetic data can identify confounding variables (e.g., CYP2A6 polymorphism) .
Q. How can computational chemistry predict rac-Nicotine 1-Oxide’s retrosynthetic pathways, and what validation experiments are required?
- Methodological Answer : AI-driven tools (e.g., Reaxys or Pistachio) propose routes via nicotine oxidation or pyrrolidine-ring functionalization. Validate predictions with DFT calculations (e.g., Gibbs free energy of transition states for oxidation steps) and experimental kinetic studies (UV-Vis monitoring of intermediate radicals). Cross-validate synthetic routes using isotopic labeling (¹⁵N-tobacco-derived nicotine) to track regioselectivity .
Q. What statistical frameworks are appropriate for analyzing dose-response relationships in rac-Nicotine 1-Oxide’s neuropharmacological studies?
- Methodological Answer : Use nonlinear mixed-effects modeling (NONMEM) for sparse in vivo data. For receptor-binding assays (e.g., α4β2 nicotinic acetylcholine receptors), apply Hill equation fits with bootstrapping to estimate EC₅₀ and cooperativity coefficients. Address batch-to-batch variability via ANOVA with post-hoc Tukey tests .
Q. How do enantiomeric differences in rac-Nicotine 1-Oxide impact its pharmacokinetic and toxicological profiles?
- Methodological Answer : Employ chiral separation (supercritical fluid chromatography) to isolate (R)- and (S)-enantiomers. Compare metabolic clearance rates using hepatocyte incubations and LC-MS/MS quantification. Toxicity assays (Ames test, zebrafish embryogenesis) paired with molecular docking (AutoDock Vina) reveal enantiomer-specific interactions with metabolic enzymes .
Q. Methodological Challenges & Contradictions
Q. Why do conflicting reports exist about rac-Nicotine 1-Oxide’s role in nicotine detoxification, and how can experimental bias be mitigated?
- Methodological Answer : Contradictions stem from variable FMO3 expression in model systems. Standardize assays using FMO3-knockout mice or humanized liver chimeric mice. Control for redox state (glutathione levels) during in vitro assays to prevent artifactual oxidation. Blind metabolite quantification to reduce analyst bias .
Q. What strategies validate the purity of rac-Nicotine 1-Oxide in pharmacological studies, given its hygroscopicity and propensity for racemization?
- Methodological Answer : Combine Karl Fischer titration for water content, chiral HPLC for enantiomeric excess, and ¹H-NMR line-shape analysis to detect degradation. Store samples as lyophilized powders and reconstitute in deuterated solvents immediately before use .
Q. Data Reporting Standards
Q. How should researchers document synthetic and analytical protocols for rac-Nicotine 1-Oxide to ensure reproducibility?
- Methodological Answer : Follow the Beilstein Journal of Organic Chemistry guidelines: report reaction conditions (solvent, catalysts, molar ratios), purification steps (Rf values, column dimensions), and spectral data (NMR shifts, MS ionization parameters). Deposit raw chromatograms and computational workflows in repositories like Zenodo .
特性
IUPAC Name |
3-(1-methylpyrrolidin-2-yl)-1-oxidopyridin-1-ium | |
---|---|---|
Source | PubChem | |
URL | https://pubchem.ncbi.nlm.nih.gov | |
Description | Data deposited in or computed by PubChem | |
InChI |
InChI=1S/C10H14N2O/c1-11-6-3-5-10(11)9-4-2-7-12(13)8-9/h2,4,7-8,10H,3,5-6H2,1H3 | |
Source | PubChem | |
URL | https://pubchem.ncbi.nlm.nih.gov | |
Description | Data deposited in or computed by PubChem | |
InChI Key |
YHXKVHQFWVYXIC-UHFFFAOYSA-N | |
Source | PubChem | |
URL | https://pubchem.ncbi.nlm.nih.gov | |
Description | Data deposited in or computed by PubChem | |
Canonical SMILES |
CN1CCCC1C2=C[N+](=CC=C2)[O-] | |
Source | PubChem | |
URL | https://pubchem.ncbi.nlm.nih.gov | |
Description | Data deposited in or computed by PubChem | |
Molecular Formula |
C10H14N2O | |
Source | PubChem | |
URL | https://pubchem.ncbi.nlm.nih.gov | |
Description | Data deposited in or computed by PubChem | |
Molecular Weight |
178.23 g/mol | |
Source | PubChem | |
URL | https://pubchem.ncbi.nlm.nih.gov | |
Description | Data deposited in or computed by PubChem | |
Retrosynthesis Analysis
AI-Powered Synthesis Planning: Our tool employs the Template_relevance Pistachio, Template_relevance Bkms_metabolic, Template_relevance Pistachio_ringbreaker, Template_relevance Reaxys, Template_relevance Reaxys_biocatalysis model, leveraging a vast database of chemical reactions to predict feasible synthetic routes.
One-Step Synthesis Focus: Specifically designed for one-step synthesis, it provides concise and direct routes for your target compounds, streamlining the synthesis process.
Accurate Predictions: Utilizing the extensive PISTACHIO, BKMS_METABOLIC, PISTACHIO_RINGBREAKER, REAXYS, REAXYS_BIOCATALYSIS database, our tool offers high-accuracy predictions, reflecting the latest in chemical research and data.
Strategy Settings
Precursor scoring | Relevance Heuristic |
---|---|
Min. plausibility | 0.01 |
Model | Template_relevance |
Template Set | Pistachio/Bkms_metabolic/Pistachio_ringbreaker/Reaxys/Reaxys_biocatalysis |
Top-N result to add to graph | 6 |
Feasible Synthetic Routes
試験管内研究製品の免責事項と情報
BenchChemで提示されるすべての記事および製品情報は、情報提供を目的としています。BenchChemで購入可能な製品は、生体外研究のために特別に設計されています。生体外研究は、ラテン語の "in glass" に由来し、生物体の外で行われる実験を指します。これらの製品は医薬品または薬として分類されておらず、FDAから任何の医療状態、病気、または疾患の予防、治療、または治癒のために承認されていません。これらの製品を人間または動物に体内に導入する形態は、法律により厳格に禁止されています。これらのガイドラインに従うことは、研究と実験において法的および倫理的な基準の遵守を確実にするために重要です。