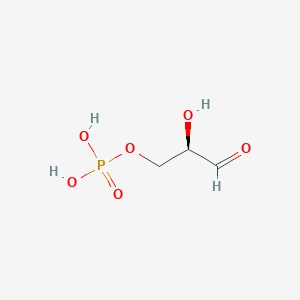
Glyceraldehyde-3-phosphate
概要
説明
Glyceraldehyde-3-phosphate, also known as triose phosphate or 3-phosphoglyceraldehyde, is a metabolite that occurs as an intermediate in several central pathways of all organisms . It is a monophosphate ester of glyceraldehyde .
Synthesis Analysis
D-glyceraldehyde 3-phosphate is formed from three compounds in reversible reactions: Fructose-1,6-bisphosphate, Dihydroxyacetone phosphate, and 1,3-bisphosphoglycerate . It is also of some importance since this is how glycerol enters the glycolytic and gluconeogenic pathways .Molecular Structure Analysis
The molecular formula of Glyceraldehyde-3-phosphate is C3H7O6P . The structure of the fully occupied monomers was compared to those with partial cofactor occupancy, identifying regions of difference that may account for the increased thermal stability .Chemical Reactions Analysis
Glyceraldehyde-3-phosphate is a key intermediate in several central metabolic pathways of all organisms . It is during this step that the energy liberated during oxidation of the aldehyde group is conserved in the form of a high-energy phosphate compound .Physical And Chemical Properties Analysis
The physical and chemical properties of Glyceraldehyde-3-phosphate include a density of 1.7±0.1 g/cm3, boiling point of 399.2±52.0 °C at 760 mmHg, vapour pressure of 0.0±2.1 mmHg at 25°C, enthalpy of vaporization of 75.1±6.0 kJ/mol, and flash point of 195.2±30.7 °C .科学的研究の応用
Role in Cellular Metabolism and Disease
Glyceraldehyde-3-phosphate plays a critical role in central metabolic pathways across various organisms. Its involvement extends to processes like glycolysis and gluconeogenesis. Studies have revealed its differential expression under physiological conditions, including cancer, hypoxia, and apoptosis. For instance, Jang et al. (2009) discovered that glyceraldehyde-3-phosphate directly suppresses caspase-3 activity, influencing the balance between cell survival and apoptosis (Jang et al., 2009).
Enzymatic Interactions
Glyceraldehyde-3-phosphate dehydrogenase, an enzyme interacting with glyceraldehyde-3-phosphate, has been extensively studied. For example, Yonuschot et al. (1970) examined its properties in spinach leaves, highlighting its role in the oxidation of glyceraldehyde-3-phosphate (Yonuschot et al., 1970).
Synthesis and Stability
Improved methods for synthesizing enantiomerically pure glyceraldehyde-3-phosphates have been reported, underlining their stability and degradation under various pH conditions, as investigated by Gauss et al. (2014) (Gauss et al., 2014).
Molecular Structure Studies
Understanding the molecular structure of glyceraldehyde-3-phosphate dehydrogenase has been vital. Harrington and Karr (1965) determined its molecular weight and subunit structure, contributing to our knowledge of enzyme mechanisms (Harrington & Karr, 1965).
Involvement in Apoptosis and Cell Survival
Further research by Lee et al. (2012) showed that glyceraldehyde-3-phosphate can prevent apoptosis by influencing the S-nitrosylation of glyceraldehyde-3-phosphate dehydrogenase, adding to our understanding of its role in cell fate decisions (Lee et al., 2012).
GAPDH in Cancer Research
The enzyme GAPDH, associated with glyceraldehyde-3-phosphate, has been identified as a therapeutic target in cancer research, as it plays a non-glycolytic role in cell death and survival mechanisms. Krasnov et al. (2013) discussed its potential in anticancer therapy (Krasnov et al., 2013).
Multifaceted Therapeutic Potential
GAPDH's involvement in various pathological processes and potential as a therapeutic target in different diseases has been explored, highlighting its versatile role beyond glycolysis (Lazarev et al., 2020).
作用機序
Glyceraldehyde-3-phosphate dehydrogenase (GAPDH) is essential for most organisms’ energy and carbon metabolism, which plays a critical role in some industrial bacteria . After perception of bacterial flagellin, GAPC1 dynamically responded with a significant increase in size of fluorescent puncta and enhanced nuclear accumulation .
Safety and Hazards
将来の方向性
Research has focused on the thermal stability of both coral and algal metabolic enzymes, since metabolic throughput is important to the calculus of mutual benefit . The thermal stability of a critical metabolic enzyme, glyceraldehyde-3-phosphate dehydrogenase, from the stony coral Acropora millepora was found to increase significantly in the presence of its cofactor NAD+ .
特性
IUPAC Name |
[(2R)-2-hydroxy-3-oxopropyl] dihydrogen phosphate | |
---|---|---|
Source | PubChem | |
URL | https://pubchem.ncbi.nlm.nih.gov | |
Description | Data deposited in or computed by PubChem | |
InChI |
InChI=1S/C3H7O6P/c4-1-3(5)2-9-10(6,7)8/h1,3,5H,2H2,(H2,6,7,8)/t3-/m0/s1 | |
Source | PubChem | |
URL | https://pubchem.ncbi.nlm.nih.gov | |
Description | Data deposited in or computed by PubChem | |
InChI Key |
LXJXRIRHZLFYRP-VKHMYHEASA-N | |
Source | PubChem | |
URL | https://pubchem.ncbi.nlm.nih.gov | |
Description | Data deposited in or computed by PubChem | |
Canonical SMILES |
C(C(C=O)O)OP(=O)(O)O | |
Source | PubChem | |
URL | https://pubchem.ncbi.nlm.nih.gov | |
Description | Data deposited in or computed by PubChem | |
Isomeric SMILES |
C([C@H](C=O)O)OP(=O)(O)O | |
Source | PubChem | |
URL | https://pubchem.ncbi.nlm.nih.gov | |
Description | Data deposited in or computed by PubChem | |
Molecular Formula |
C3H7O6P | |
Source | PubChem | |
URL | https://pubchem.ncbi.nlm.nih.gov | |
Description | Data deposited in or computed by PubChem | |
DSSTOX Substance ID |
DTXSID70331398 | |
Record name | glyceraldehyde-3-phosphate | |
Source | EPA DSSTox | |
URL | https://comptox.epa.gov/dashboard/DTXSID70331398 | |
Description | DSSTox provides a high quality public chemistry resource for supporting improved predictive toxicology. | |
Molecular Weight |
170.06 g/mol | |
Source | PubChem | |
URL | https://pubchem.ncbi.nlm.nih.gov | |
Description | Data deposited in or computed by PubChem | |
Physical Description |
Solid | |
Record name | Glyceraldehyde 3-phosphate | |
Source | Human Metabolome Database (HMDB) | |
URL | http://www.hmdb.ca/metabolites/HMDB0001112 | |
Description | The Human Metabolome Database (HMDB) is a freely available electronic database containing detailed information about small molecule metabolites found in the human body. | |
Explanation | HMDB is offered to the public as a freely available resource. Use and re-distribution of the data, in whole or in part, for commercial purposes requires explicit permission of the authors and explicit acknowledgment of the source material (HMDB) and the original publication (see the HMDB citing page). We ask that users who download significant portions of the database cite the HMDB paper in any resulting publications. | |
Product Name |
Glyceraldehyde-3-phosphate | |
CAS RN |
591-57-1 | |
Record name | Triose phosphate | |
Source | CAS Common Chemistry | |
URL | https://commonchemistry.cas.org/detail?cas_rn=591-57-1 | |
Description | CAS Common Chemistry is an open community resource for accessing chemical information. Nearly 500,000 chemical substances from CAS REGISTRY cover areas of community interest, including common and frequently regulated chemicals, and those relevant to high school and undergraduate chemistry classes. This chemical information, curated by our expert scientists, is provided in alignment with our mission as a division of the American Chemical Society. | |
Explanation | The data from CAS Common Chemistry is provided under a CC-BY-NC 4.0 license, unless otherwise stated. | |
Record name | 3-Phosphoglyceraldehyde, D- | |
Source | ChemIDplus | |
URL | https://pubchem.ncbi.nlm.nih.gov/substance/?source=chemidplus&sourceid=0000591571 | |
Description | ChemIDplus is a free, web search system that provides access to the structure and nomenclature authority files used for the identification of chemical substances cited in National Library of Medicine (NLM) databases, including the TOXNET system. | |
Record name | D-glyceraldehyde 3-phosphate | |
Source | DrugBank | |
URL | https://www.drugbank.ca/drugs/DB02263 | |
Description | The DrugBank database is a unique bioinformatics and cheminformatics resource that combines detailed drug (i.e. chemical, pharmacological and pharmaceutical) data with comprehensive drug target (i.e. sequence, structure, and pathway) information. | |
Explanation | Creative Common's Attribution-NonCommercial 4.0 International License (http://creativecommons.org/licenses/by-nc/4.0/legalcode) | |
Record name | glyceraldehyde-3-phosphate | |
Source | EPA DSSTox | |
URL | https://comptox.epa.gov/dashboard/DTXSID70331398 | |
Description | DSSTox provides a high quality public chemistry resource for supporting improved predictive toxicology. | |
Record name | 3-PHOSPHOGLYCERALDEHYDE, D- | |
Source | FDA Global Substance Registration System (GSRS) | |
URL | https://gsrs.ncats.nih.gov/ginas/app/beta/substances/TD9ZNF8JPW | |
Description | The FDA Global Substance Registration System (GSRS) enables the efficient and accurate exchange of information on what substances are in regulated products. Instead of relying on names, which vary across regulatory domains, countries, and regions, the GSRS knowledge base makes it possible for substances to be defined by standardized, scientific descriptions. | |
Explanation | Unless otherwise noted, the contents of the FDA website (www.fda.gov), both text and graphics, are not copyrighted. They are in the public domain and may be republished, reprinted and otherwise used freely by anyone without the need to obtain permission from FDA. Credit to the U.S. Food and Drug Administration as the source is appreciated but not required. | |
Record name | Glyceraldehyde 3-phosphate | |
Source | Human Metabolome Database (HMDB) | |
URL | http://www.hmdb.ca/metabolites/HMDB0001112 | |
Description | The Human Metabolome Database (HMDB) is a freely available electronic database containing detailed information about small molecule metabolites found in the human body. | |
Explanation | HMDB is offered to the public as a freely available resource. Use and re-distribution of the data, in whole or in part, for commercial purposes requires explicit permission of the authors and explicit acknowledgment of the source material (HMDB) and the original publication (see the HMDB citing page). We ask that users who download significant portions of the database cite the HMDB paper in any resulting publications. | |
Retrosynthesis Analysis
AI-Powered Synthesis Planning: Our tool employs the Template_relevance Pistachio, Template_relevance Bkms_metabolic, Template_relevance Pistachio_ringbreaker, Template_relevance Reaxys, Template_relevance Reaxys_biocatalysis model, leveraging a vast database of chemical reactions to predict feasible synthetic routes.
One-Step Synthesis Focus: Specifically designed for one-step synthesis, it provides concise and direct routes for your target compounds, streamlining the synthesis process.
Accurate Predictions: Utilizing the extensive PISTACHIO, BKMS_METABOLIC, PISTACHIO_RINGBREAKER, REAXYS, REAXYS_BIOCATALYSIS database, our tool offers high-accuracy predictions, reflecting the latest in chemical research and data.
Strategy Settings
Precursor scoring | Relevance Heuristic |
---|---|
Min. plausibility | 0.01 |
Model | Template_relevance |
Template Set | Pistachio/Bkms_metabolic/Pistachio_ringbreaker/Reaxys/Reaxys_biocatalysis |
Top-N result to add to graph | 6 |
Feasible Synthetic Routes
Q & A
A: Glyceraldehyde-3-phosphate (G3P) is a key intermediate molecule in several metabolic pathways, including glycolysis and gluconeogenesis. [] In glycolysis, G3P is generated from the breakdown of glucose and is further metabolized to produce energy in the form of ATP. It can also be used as a precursor for other molecules, such as lipids and amino acids.
A: G3P binds to the active site of GAPDH, a key enzyme in glycolysis. [, ] This binding is essential for the enzyme's catalytic activity, which involves the oxidation of G3P to 1,3-diphosphoglycerate. [] This reaction is coupled with the reduction of NAD+ to NADH, a crucial electron carrier in cellular respiration.
ANone: Glyceraldehyde 3-phosphate, also known as triose phosphate or 3-phosphoglyceraldehyde, is a 3-carbon sugar phosphate. It is the phosphate ester of the triose sugar, glyceraldehyde. While we don't have specific spectroscopic data from the provided research, its molecular formula is C3H7O6P and its molecular weight is 170.06 g/mol.
A: A coupled reaction is a process where an energetically unfavorable reaction (one that requires energy) is driven by an energetically favorable reaction (one that releases energy). In the case of G3P and GAPDH, the oxidation of G3P to 1,3-diphosphoglycerate is energetically favorable. This energy release is coupled to the phosphorylation of ADP to ATP, which is an energetically unfavorable reaction. This coupling allows for the efficient production of ATP, the primary energy currency of cells. []
A: Oxidative stress can lead to the oxidation of cysteine residues in GAPDH, particularly the catalytic cysteine residue Cys-152. [, , ] This oxidation can inhibit GAPDH activity, affecting its ability to bind and metabolize G3P. [] The resulting disruption in glycolysis can have significant downstream effects on cellular function.
A: Research suggests a link between GAPDH, G3P, and apoptosis, a programmed cell death process. Studies show that under oxidative stress, GAPDH can translocate to the nucleus and form aggregates. [, ] These aggregates have been implicated in neuronal cell death. [] While the exact mechanism linking G3P to this process is unclear, the disruption of GAPDH function due to oxidative stress likely plays a role.
A: Several research papers delve into the specific interactions of G3P and GAPDH. For example, one study used immobilized GAPDH to investigate its complex formation with phosphoglycerate kinase, another enzyme involved in glycolysis. [] This study demonstrated the formation of stable bienzyme complexes, highlighting the potential for metabolic channeling within the pathway.
A: "Channeling" refers to the direct transfer of a metabolite (like G3P) between two sequentially acting enzymes (like GAPDH and phosphoglycerate kinase) without it being released into the bulk solution. [] This phenomenon is thought to increase the efficiency of metabolic pathways by reducing diffusion distances and minimizing the loss of intermediates. []
A: Isoenzymes are enzymes that catalyze the same chemical reaction but have different amino acid sequences. [, ] This difference in sequence can lead to variations in their kinetic properties, such as their affinity for substrates like G3P. For example, the study on yeast GAPDH isoenzymes (Tdh2 and Tdh3) showed that they have different sensitivities to oxidative stress, suggesting potential differences in their interaction with G3P under these conditions. []
試験管内研究製品の免責事項と情報
BenchChemで提示されるすべての記事および製品情報は、情報提供を目的としています。BenchChemで購入可能な製品は、生体外研究のために特別に設計されています。生体外研究は、ラテン語の "in glass" に由来し、生物体の外で行われる実験を指します。これらの製品は医薬品または薬として分類されておらず、FDAから任何の医療状態、病気、または疾患の予防、治療、または治癒のために承認されていません。これらの製品を人間または動物に体内に導入する形態は、法律により厳格に禁止されています。これらのガイドラインに従うことは、研究と実験において法的および倫理的な基準の遵守を確実にするために重要です。