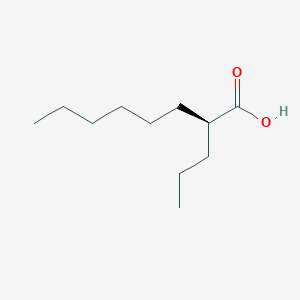
S-(+)-Arundic Acid
説明
S-(+)-Arundic Acid ((S)ONO-2506) is a chiral carboxylic acid and the S-enantiomer of Arundic Acid. It acts as an astrocyte modulator, primarily inhibiting the synthesis of S100B, a calcium-binding protein critical for glial cell function . S100B is expressed in astrocytes and enteric glial cells (EGCs) and plays roles in cell proliferation, differentiation, and neuroprotection. In the enteric nervous system (ENS), this compound has been shown to disrupt S100B-mediated pathways, reducing Sox10 expression (a transcription factor essential for glial lineage maintenance) and impairing enteric neural crest cell (ENCC) proliferation during development .
準備方法
Chiral Auxiliary-Mediated Asymmetric Synthesis
NCPS-Supported Oxazolidine Auxiliaries
The most efficient route to S-(+)-arundic acid involves a stereoselective alkylation using non-cross-linked polystyrene (NCPS)-supported (4S)-2-phenylimino-2-oxazolidine as a chiral auxiliary . This method, developed by Lu et al., proceeds via three steps:
-
Acylation : NCPS-bound (4S)-2-phenylimino-2-oxazolidine is acylated with octanoyl chloride in dichloromethane, mediated by triethylamine and DMAP, yielding the polymer-supported intermediate in 90% yield .
-
Alkylation : The enolate generated with lithium hexamethyldisilazide (LiHMDS) undergoes stereoselective alkylation with propargyl bromide at −78°C, establishing the (S)-configuration with 99% ee .
-
Hydrolysis and Recovery : Oxidative cleavage with lithium hydroxide and hydrogen peroxide releases this compound while regenerating the NCPS auxiliary for reuse .
Key Advantages :
-
Enantiomeric Excess : 99.0% ee confirmed via HPLC (Chiralcel OJ-RH column) .
-
Sustainability : NCPS auxiliary recovery exceeds 90%, reducing costs and waste .
Table 1: Reaction Conditions and Outcomes for NCPS-Mediated Synthesis
Step | Reagents/Conditions | Yield (%) | ee (%) |
---|---|---|---|
Acylation | Octanoyl chloride, Et₃N, DMAP, CH₂Cl₂ | 90 | – |
Alkylation | LiHMDS, propargyl bromide, −78°C | 85 | 99 |
Hydrolysis | LiOH, H₂O₂, THF/H₂O | 62 | 99 |
Camphorsultam-Based Diastereoselective Alkylation
Traditional Synthesis via Oppolzer’s Sultam
An alternative approach employs (−)-2,10-camphorsultam as a chiral auxiliary, as outlined in DrugFuture’s synthesis pathway :
-
Acylation : Camphorsultam reacts with octanoyl chloride to form an N-octanoyl sultam.
-
Alkylation : The lithium enolate is alkylated with allyl bromide, yielding a diastereomerically pure product.
-
Hydrogenation and Hydrolysis : Catalytic hydrogenation (Pd/C or Pt/C) saturates the allyl group, followed by oxidative cleavage to release the carboxylic acid .
Challenges :
-
Cost : Camphorsultam is expensive and difficult to recover .
-
Yield Limitations : Multi-step sequences reduce overall efficiency compared to NCPS methods.
Table 2: Comparison of NCPS vs. Camphorsultam Methods
Parameter | NCPS Method | Camphorsultam Method |
---|---|---|
Overall Yield | 60% | Not reported |
ee | 99% | >98% (assumed) |
Auxiliary Recovery | >90% | Low |
Cost Efficiency | High | Low |
Mechanistic Insights into Stereoselective Alkylation
Enolate Formation and Steric Control
The high enantioselectivity in the NCPS method arises from the chiral oxazolidine auxiliary directing the alkylation via a rigid transition state. The (4S)-configuration induces a steric bias, favoring propargyl bromide attack from the re face . Computational studies suggest that the NCPS support stabilizes the enolate through π-π interactions, further enhancing selectivity .
Solvent and Temperature Effects
Optimal results are achieved in tetrahydrofuran (THF) at −78°C, which suppresses side reactions and stabilizes the enolate . Polar aprotic solvents like DMF reduce selectivity due to competitive solvation.
Recent Advances and Alternative Approaches
Organocatalytic Strategies
Emerging methodologies employ carbenium ion organocatalysts for asymmetric alkylation of aldehydes, though yields remain suboptimal (<40%) .
Continuous Flow Systems
Pilot-scale studies demonstrate NCPS auxiliary reuse over 10 cycles without loss of activity, highlighting potential for industrial adoption .
化学反応の分析
Types of Reactions: (2S)-2-Propyloctanoic Acid undergoes various chemical reactions, including:
Oxidation: It can be oxidized to form corresponding ketones or aldehydes.
Reduction: Reduction reactions can convert it into alcohols.
Substitution: The carboxyl group can be substituted with other functional groups through nucleophilic substitution reactions.
Common Reagents and Conditions:
Oxidation: Common oxidizing agents include potassium permanganate (KMnO4) and chromium trioxide (CrO3).
Reduction: Reducing agents such as lithium aluminum hydride (LiAlH4) or sodium borohydride (NaBH4) are frequently used.
Substitution: Reagents like thionyl chloride (SOCl2) or phosphorus tribromide (PBr3) facilitate substitution reactions.
Major Products:
Oxidation: Produces ketones or aldehydes.
Reduction: Yields alcohols.
Substitution: Results in various substituted carboxylic acids.
科学的研究の応用
Neuroprotective Agent
S-(+)-Arundic Acid has been extensively studied for its neuroprotective effects, particularly in the context of neurodegenerative diseases and acute neurological events.
Clinical Trials
Phase II clinical trials have been conducted to evaluate the efficacy of arundic acid in treating acute ischemic stroke and other neurodegenerative diseases. These trials have shown promising results, indicating a potential role for arundic acid in clinical settings .
Ischemic Stroke
Research indicates that arundic acid can ameliorate delayed ischemic brain damage by enhancing glutamate uptake through upregulation of GLAST (glutamate aspartate transporter) expression. This mechanism helps prevent excitotoxicity associated with excessive glutamate levels during ischemia .
Glaucoma
In studies involving retinal ganglion cells (RGCs), arundic acid has been shown to prevent RGC death by increasing GLAST expression. This suggests its potential application in treating glaucoma, a condition affecting millions worldwide and leading to blindness .
Multiple Sclerosis
Recent investigations have highlighted arundic acid's immunomodulatory effects in experimental autoimmune encephalomyelitis (EAE), a model for multiple sclerosis. The compound reduced immune cell infiltration and pro-inflammatory cytokine levels, indicating its potential as a therapeutic agent for MS .
Summary of Case Studies
作用機序
The mechanism of action of (2S)-2-Propyloctanoic Acid involves its interaction with specific molecular targets, such as enzymes or receptors. Its chiral nature allows it to fit into enzyme active sites or receptor binding pockets with high specificity, influencing biochemical pathways. The exact pathways and targets depend on the context of its application, whether in metabolic studies or therapeutic research.
類似化合物との比較
Mechanism of Action: S100B Inhibition
- S-(+)-Arundic Acid: Selectively inhibits S100B synthesis, reducing intracellular S100B levels. This leads to decreased Sox10 expression and ENCC proliferation in embryonic ENS cultures, without affecting mature neurons .
- For example, extracellular S100B at high concentrations exacerbates neuronal damage during inflammation , but exogenous S100B addition fails to rescue Sox10 expression in Arundic Acid-treated cultures, highlighting the compound’s cell-intrinsic mechanism .
Neuroprotective Agents in Stroke
Effects on Glial vs. Neuronal Lineages
- This compound : Reduces Sox10+ glial progenitors and ENCC proliferation but spares Hu+ neurons . This specificity contrasts with compounds like Geminin, which induces p53-dependent apoptosis in neural crest cells but affects both neurons and glia .
Enantiomeric Specificity
- This enantioselectivity is critical for its efficacy, as seen in its ability to inhibit S100B at 300 µM in vitro .
Table 1: Key Comparative Data
Research Findings and Clinical Relevance
生物活性
S-(+)-Arundic Acid, also known as ONO-2506, is a compound that has garnered attention for its neuroprotective properties and its ability to modulate astrocytic functions. This article explores the biological activity of this compound, focusing on its mechanisms of action, therapeutic potential in neurodegenerative diseases, and relevant research findings.
This compound primarily exerts its effects through the modulation of astrocytic functions and the inhibition of S100B protein synthesis. S100B is a calcium-binding protein secreted by astrocytes, which can have neurotrophic effects at low concentrations but becomes neurotoxic at higher levels. The compound's ability to inhibit S100B synthesis is crucial in mitigating neuroinflammation and protecting against neuronal damage.
Key Mechanisms:
- Inhibition of S100B: Arundic acid reduces the synthesis and secretion of S100B in astrocytes, leading to decreased neuroinflammation and protection against excitotoxicity.
- Activation of Signaling Pathways: It enhances the expression of excitatory amino acid transporter 1 (EAAT1) through the activation of the NF-κB, Akt, and ERK signaling pathways. This upregulation is significant for glutamate uptake, which is critical in preventing excitotoxic damage in various neurological conditions .
- Reduction of Reactive Species: Arundic acid has been shown to decrease levels of reactive nitrogen and oxygen species, contributing further to its neuroprotective effects .
Therapeutic Potential
This compound has demonstrated efficacy in various preclinical models related to neurodegenerative diseases:
- Glaucoma Models: In studies involving glaucoma mouse models, this compound prevented retinal ganglion cell death by increasing EAAT1 expression .
- Parkinson’s Disease Models: The compound has shown protective effects against dopaminergic neuronal loss in MPTP-induced models by suppressing S100B expression and reducing oxidative stress .
- Multiple Sclerosis Models: Research indicates that it modulates astrocytosis and demyelination during experimental autoimmune encephalomyelitis (EAE), suggesting potential applications in treating multiple sclerosis .
Research Findings
A summary of significant studies on this compound is presented below:
Study | Findings | Model |
---|---|---|
Arundic acid increases EAAT1 expression | Enhanced glutamate uptake and reduced excitotoxicity | Human astrocyte cultures |
Neuroprotection against MPTP-induced damage | Reduced dopaminergic neuron loss via S100B suppression | Mouse model of Parkinson’s disease |
Modulation of immune response in EAE | Decreased inflammatory markers and improved histological outcomes | Mouse model of multiple sclerosis |
Prevention of retinal ganglion cell death | Increased EAAT1 expression leading to reduced glutamate toxicity | Glaucoma mouse model |
Case Studies
Several case studies have highlighted the effectiveness of this compound in various contexts:
- Case Study 1: In a study on status epilepticus induced by lithium-pilocarpine in young rats, treatment with Arundic acid resulted in significant downregulation of neuroinflammation markers and improved hippocampal tissue integrity .
- Case Study 2: In chronic EAE models, administration of Arundic acid led to a notable reduction in CD68-positive reactive microglia and improved clinical scores, indicating its potential as an immunomodulatory agent .
Q & A
Basic Research Questions
Q. What experimental models are most suitable for studying the mechanistic role of S-(+)-Arundic Acid in neural crest cell (ENCC) development?
- Methodological Answer : In vitro models (e.g., primary enteric glial cell cultures) and in vivo rodent models are commonly used. For example, GFP+ ENCC quantification in murine duodenal and jejunal tissues under DMSO vs. This compound treatment provides insights into dose-dependent effects on cell proliferation . Immunofluorescence and statistical analysis (error bars, significance markers) are critical for validating results .
Q. How should researchers address ethical considerations in animal studies involving this compound?
- Methodological Answer : Submit protocols to institutional animal care committees (IACUC) for approval. Report animal welfare metrics (e.g., weight loss, distress signs) and euthanasia methods. Follow ARRIVE guidelines for transparent reporting .
特性
IUPAC Name |
(2S)-2-propyloctanoic acid | |
---|---|---|
Source | PubChem | |
URL | https://pubchem.ncbi.nlm.nih.gov | |
Description | Data deposited in or computed by PubChem | |
InChI |
InChI=1S/C11H22O2/c1-3-5-6-7-9-10(8-4-2)11(12)13/h10H,3-9H2,1-2H3,(H,12,13)/t10-/m0/s1 | |
Source | PubChem | |
URL | https://pubchem.ncbi.nlm.nih.gov | |
Description | Data deposited in or computed by PubChem | |
InChI Key |
YCYMCMYLORLIJX-JTQLQIEISA-N | |
Source | PubChem | |
URL | https://pubchem.ncbi.nlm.nih.gov | |
Description | Data deposited in or computed by PubChem | |
Canonical SMILES |
CCCCCCC(CCC)C(=O)O | |
Source | PubChem | |
URL | https://pubchem.ncbi.nlm.nih.gov | |
Description | Data deposited in or computed by PubChem | |
Isomeric SMILES |
CCCCCC[C@H](CCC)C(=O)O | |
Source | PubChem | |
URL | https://pubchem.ncbi.nlm.nih.gov | |
Description | Data deposited in or computed by PubChem | |
Molecular Formula |
C11H22O2 | |
Source | PubChem | |
URL | https://pubchem.ncbi.nlm.nih.gov | |
Description | Data deposited in or computed by PubChem | |
DSSTOX Substance ID |
DTXSID90431147 | |
Record name | (2S)-2-Propyloctanoic acid | |
Source | EPA DSSTox | |
URL | https://comptox.epa.gov/dashboard/DTXSID90431147 | |
Description | DSSTox provides a high quality public chemistry resource for supporting improved predictive toxicology. | |
Molecular Weight |
186.29 g/mol | |
Source | PubChem | |
URL | https://pubchem.ncbi.nlm.nih.gov | |
Description | Data deposited in or computed by PubChem | |
CAS No. |
807363-10-6 | |
Record name | (2S)-2-Propyloctanoic acid | |
Source | EPA DSSTox | |
URL | https://comptox.epa.gov/dashboard/DTXSID90431147 | |
Description | DSSTox provides a high quality public chemistry resource for supporting improved predictive toxicology. | |
試験管内研究製品の免責事項と情報
BenchChemで提示されるすべての記事および製品情報は、情報提供を目的としています。BenchChemで購入可能な製品は、生体外研究のために特別に設計されています。生体外研究は、ラテン語の "in glass" に由来し、生物体の外で行われる実験を指します。これらの製品は医薬品または薬として分類されておらず、FDAから任何の医療状態、病気、または疾患の予防、治療、または治癒のために承認されていません。これらの製品を人間または動物に体内に導入する形態は、法律により厳格に禁止されています。これらのガイドラインに従うことは、研究と実験において法的および倫理的な基準の遵守を確実にするために重要です。