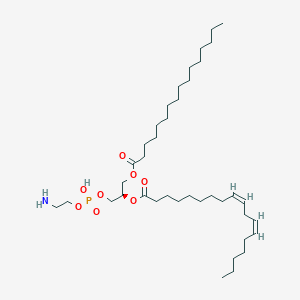
1-Palmitoyl-2-linoleoyl PE
概要
説明
1-Palmitoyl-2-linoleoyl phosphatidylethanolamine (PE) is a glycerophospholipid characterized by a palmitic acid (16:0, saturated) esterified at the sn-1 position and a linoleic acid (18:2, polyunsaturated) at the sn-2 position of the glycerol backbone, with an ethanolamine head group . This unique fatty acid composition confers distinct biophysical properties, such as increased membrane fluidity and curvature, due to the unsaturated linoleoyl chain introducing disorder in lipid bilayers .
Structurally, the ethanolamine head group imparts a neutral charge at physiological pH, distinguishing it from anionic phosphatidylserine (PS) or zwitterionic phosphatidylcholine (PC). This lipid is naturally abundant in biological membranes and has been identified as a major molecular species in rice bran phospholipids, alongside phosphatidylcholine (PC) and phosphatidylinositol (PI) . Its role extends beyond structural membrane integrity; it serves as a substrate for phospholipase A1 (PLA1) in metabolic studies and participates in signaling pathways, such as oxidized phospholipid-mediated activation of G protein-coupled receptors (e.g., G2A) when hydrolyzed .
準備方法
Biochemical Pathways for Phosphatidylethanolamine Synthesis
Phosphatidylethanolamines (PEs) are synthesized through two primary pathways in biological systems: the CDP-ethanolamine pathway and phosphatidylserine (PS) decarboxylation . These pathways have been adapted for in vitro synthesis of PLPE with modifications to incorporate specific acyl chains .
CDP-Ethanolamine Pathway
The CDP-ethanolamine pathway involves three enzymatic steps:
-
Ethanolamine phosphorylation : Ethanolamine is phosphorylated by ethanolamine kinase to form phosphoethanolamine.
-
CTP coupling : Phosphoethanolamine reacts with cytidine triphosphate (CTP) via phosphoethanolamine cytidylyltransferase to produce CDP-ethanolamine.
-
Diacylglycerol (DAG) transfer : CDP-ethanolamine transfers its phosphoethanolamine group to DAG, catalyzed by ethanolamine phosphotransferase, yielding PE .
For PLPE synthesis, DAG must be pre-acylated with palmitic and linoleic acids. This requires:
-
sn-1 acylation : Palmitoyl-CoA is esterified to sn-1 of glycerol-3-phosphate via glycerol-3-phosphate acyltransferase.
-
sn-2 acylation : Linoleoyl-CoA is introduced at the sn-2 position by 1-acylglycerol-3-phosphate acyltransferase .
Phosphatidylserine Decarboxylation
PS decarboxylation is a mitochondrial pathway where PS is converted to PE by phosphatidylserine decarboxylase. While this pathway is less direct for PLPE synthesis, it can be exploited by first synthesizing PS with the desired acyl chains and then decarboxylating it .
Chemical Synthesis Strategies
Chemical synthesis of PLPE emphasizes regioselective acylation and head-group modification. Key methods include:
Stepwise Acylation of Glycerol Backbone
A common strategy involves protecting the sn-3 hydroxyl group of glycerol, followed by sequential acylation:
-
sn-1 acylation : Palmitic acid is activated as an acyl chloride or using carbodiimide coupling reagents (e.g., DCC) and reacted with the protected glycerol.
-
sn-2 acylation : Linoleic acid is introduced under controlled conditions to avoid acyl migration.
-
Phosphoethanolamine attachment : The sn-3 hydroxyl is deprotected and coupled to phosphoethanolamine using phosphorylating agents .
A critical challenge is preventing acyl migration during synthesis. Studies recommend low temperatures (0–5°C) and non-polar solvents (e.g., dichloromethane) to stabilize the intermediate .
Enzymatic Synthesis
Lipases and phospholipases offer regiospecific catalysis. For example:
-
Phospholipase A2 (PLA2) : Hydrolyzes the sn-2 acyl chain of native PE, enabling its replacement with linoleic acid via acyl-CoA donors.
-
Phospholipase D (PLD) : Catalyzes transphosphatidylation, exchanging the head group of phosphatidylcholine (PC) with ethanolamine to form PE .
A representative protocol involves:
-
Preparing 1-palmitoyl-2-lysophosphatidylethanolamine via PLA2-mediated hydrolysis.
-
Reacting the lysolipid with linoleoyl-CoA in the presence of acyltransferase.
-
Purifying PLPE using column chromatography (silica gel, chloroform/methanol eluent) .
Industrial-Scale Production and Patent Innovations
Recent patents highlight innovations in acylating agents and purification techniques. For example, EP3808729A1 and US20210107857A1 describe methods for synthesizing structurally related compounds (e.g., 1-palmitoyl-2-linoleoyl-3-acetyl glycerol) that inform PLPE production :
Acetylation and Acylation Optimization
-
Acetylating agents : Mixtures of acetic anhydride and acetyl chloride reduce byproducts (e.g., HCl) compared to acetyl chloride alone, enhancing intermediate stability .
-
Solvent systems : Dichloromethane and hexane improve solubility of hydrophobic intermediates, facilitating crystallization .
Parameter | Conditions for High Yield |
---|---|
Reaction Temperature | 5–30°C |
Solvent | Dichloromethane/hexane (3:1) |
Catalysts | Dimethylaminopyridine (DMAP), triethylamine |
Yield | 74–86% (analogous intermediates) |
Purification Techniques
-
Crystallization : Cooling hexane solutions to -10°C precipitates intermediates with >95% purity .
-
Column chromatography : Silica gel columns with chloroform/methanol gradients resolve PLPE from lyso-PE and unreacted DAG .
Analytical Validation of PLPE
Post-synthesis analysis ensures structural integrity and purity:
化学反応の分析
Types of Reactions
1-hexadecanoyl-2-(9Z,12Z-octadecadienoyl)-sn-glycero-3-phosphoethanolamine undergoes various chemical reactions, including:
Oxidation: The linoleoyl group can undergo oxidation, leading to the formation of hydroperoxides and other oxidative products.
Hydrolysis: The ester bonds can be hydrolyzed by lipases, resulting in the release of free fatty acids and glycerophosphoethanolamine.
Substitution: The phosphoethanolamine group can participate in substitution reactions, such as the formation of phosphatidylserine through the exchange of ethanolamine with serine.
Common Reagents and Conditions
Oxidation: Catalysts like lipoxygenase or chemical oxidants such as hydrogen peroxide.
Hydrolysis: Enzymes like phospholipase A2 or chemical hydrolysis using acidic or basic conditions.
Substitution: Enzymatic transphosphatidylation using phosphatidylserine synthase.
Major Products
Oxidation: Hydroperoxides, aldehydes, and ketones.
Hydrolysis: Free fatty acids, glycerophosphoethanolamine.
Substitution: Phosphatidylserine, other substituted phospholipids.
科学的研究の応用
Biochemical Properties
PLPE is characterized by its amphiphilic nature, which allows it to form lipid bilayers similar to those found in biological membranes. This property is essential for studying membrane dynamics and cellular signaling pathways. The compound's molecular formula is C₃₉H₇₃NO₈P, with a molecular weight of approximately 703.02 g/mol. Its structure consists of two acyl chains: palmitoyl (C16:0) and linoleoyl (C18:2), contributing to its unique biological functions.
Cell Membrane Studies
PLPE is extensively used in research related to cell membranes due to its ability to mimic natural membrane structures. It facilitates the study of:
- Membrane Fluidity : PLPE influences the fluidity of lipid bilayers, which is crucial for the function of membrane proteins.
- Lipid Raft Formation : Its interactions with cholesterol help understand the stability and formation of lipid rafts, which are essential for signal transduction .
Enzyme Substrate
PLPE serves as a substrate for various enzymes, particularly phospholipases. This application allows researchers to measure enzyme activity and understand their roles in biological processes:
- Phospholipase A2 Activity : PLPE can be used to assess the activity of secretory phospholipase A2 (sPLA2-IIA), aiding in the study of inflammatory responses.
Signal Transduction
Research indicates that PLPE modulates signaling pathways involving phosphatidylinositol, impacting processes such as apoptosis and inflammation. Its fatty acid components can influence cellular metabolism and inflammatory responses .
Immunomodulation
Studies have shown that PLPE may have immunomodulatory effects, particularly in conditions characterized by excessive immune activity:
- Asthma and Allergic Responses : PLPE has been investigated for its potential to alleviate asthma phenotypes by modulating cytokine production and eosinophil migration .
Cancer Research
PLPE's role in cancer therapy has garnered attention due to its effects on tumor cells:
- Metastasis Inhibition : Research has demonstrated that PLPE can inhibit matrix metalloproteinase (MMP) expression in cancer cells stimulated by epidermal growth factor (EGF), suggesting a potential role in preventing cancer metastasis .
Case Studies and Research Findings
Several studies have highlighted the applications of PLPE:
- Immunomodulatory Effects : A clinical trial investigated PLPE's effects on immune functions, demonstrating its potential to modulate cytokine production and improve conditions related to autoimmune diseases .
- Cancer Metastasis : Research focused on PLPE's ability to inhibit EGF-induced metastasis in breast cancer cells showed promising results, indicating its potential as an anti-cancer agent .
- Inflammatory Responses : Studies have explored how PLPE can mitigate inflammation associated with chemotherapy treatments, providing insights into its therapeutic applications for cancer patients .
作用機序
The mechanism of action of 1-hexadecanoyl-2-(9Z,12Z-octadecadienoyl)-sn-glycero-3-phosphoethanolamine involves its incorporation into biological membranes, where it influences membrane fluidity and permeability. The linoleoyl group can undergo oxidation, generating bioactive lipid mediators that modulate inflammatory responses. Additionally, the phosphoethanolamine head group can interact with proteins and other biomolecules, affecting signaling pathways and cellular functions.
類似化合物との比較
Structural Analogs: Fatty Acid Variations in PE
Table 1: Comparison of PE Species with Different Fatty Acid Compositions
Key Findings :
- Unsaturation at sn-2 (e.g., linoleic or arachidonic acid) increases membrane fluidity compared to saturated analogs like 1,2-dipalmitoyl PE .
- 1-Stearoyl-2-arachidonoyl PE is enriched in signaling tissues, where arachidonic acid serves as a precursor for eicosanoids .
Head Group Variations: PE vs. PC, PS, and PA
Table 2: Comparison with Phospholipids Sharing sn-1/sn-2 Fatty Acids
Key Findings :
- The ethanolamine head group in PE reduces electrostatic interactions compared to anionic PS or PA, influencing membrane protein localization .
- PC is resistant to PLA1 but a primary substrate for secretory PLA2 (sPLA2), while PE is preferentially hydrolyzed by PLA1 in metabolic studies .
Functional and Metabolic Differences
Oxidation and Signaling
- Oxidized 1-palmitoyl-2-linoleoyl PC, when hydrolyzed by PLA2, activates the G2A receptor via liberated oxidized free fatty acids (e.g., 9-HODE) . In contrast, oxidized PE derivatives are less studied but may exhibit distinct receptor affinities due to head group interactions.
Natural Abundance and Sources
- In rice bran, this compound is a major PE species, whereas PC analogs like 1-oleoyl-2-oleoyl PC dominate in glutinous rice strains . This divergence highlights how acyl chain composition adapts to biological requirements.
Therapeutic Potential
- Its metabolites, however, are less bioactive compared to PLAG derivatives in resolving neutrophil infiltration .
生物活性
1-Palmitoyl-2-linoleoyl phosphatidylethanolamine (PE) is a phospholipid that plays a significant role in various biological processes, including cell signaling, membrane dynamics, and inflammation. This article explores its biological activity, focusing on its immunomodulatory effects, anti-inflammatory properties, and potential therapeutic applications.
Chemical Structure and Properties
1-Palmitoyl-2-linoleoyl PE is characterized by its unique acyl chain composition:
- Palmitic Acid (C16:0) : A saturated fatty acid.
- Linoleic Acid (C18:2) : An unsaturated fatty acid.
This combination contributes to the fluidity and functionality of cellular membranes, impacting various biochemical pathways.
Immunomodulatory Effects
Research indicates that this compound exhibits significant immunomodulatory properties. A study involving healthy adults showed that supplementation with a related compound, 1-palmitoyl-2-linoleoyl-3-acetyl-rac-glycerol (PLAG), led to decreased production of pro-inflammatory cytokines IL-4 and IL-6 in peripheral blood mononuclear cells (PBMCs) compared to a control group receiving soybean oil. This suggests a potential role in managing excessive immune responses associated with autoimmune conditions .
Table 1: Effects of PLAG Supplementation on Cytokine Production
Cytokine | Control Group (Soybean Oil) | PLAG Group | p-value |
---|---|---|---|
IL-4 | Higher | Lower | <0.001 |
IL-6 | Higher | Lower | <0.001 |
IL-2 | Unchanged | Unchanged | N.S. |
IFN-γ | Unchanged | Unchanged | N.S. |
Anti-inflammatory Properties
This compound has been shown to mitigate inflammation in various models. In vitro studies indicate that it can reduce matrix metalloproteinase (MMP) expression in EGF-stimulated breast cancer cells, suggesting its potential as an anti-metastatic agent. The compound enhances the degradation of epidermal growth factor receptors (EGFR), thereby attenuating signaling pathways associated with cancer progression .
Case Study: Chemotherapy-Induced Inflammation
A clinical trial assessed the impact of PLAG on patients undergoing chemotherapy. Results indicated that PLAG supplementation helped alleviate symptoms such as oral mucositis and neutropenia, common complications of chemotherapy treatments .
The biological activity of this compound is mediated through several mechanisms:
- Cytokine Modulation : The compound influences cytokine production, reducing pro-inflammatory markers.
- EGFR Trafficking : It promotes the internalization and degradation of EGFRs, leading to decreased MMP expression and reduced metastatic potential in cancer cells .
- Lipid Metabolism : It plays a role in the biosynthesis of bioactive lipids such as anandamide through the phospholipase A2 pathway, which is crucial for various physiological processes .
Q & A
Q. How can researchers determine the role of 1-palmitoyl-2-linoleoyl PE in membrane bilayer dynamics?
Level: Basic
Methodological Answer:
To investigate its role in membrane dynamics, researchers can employ biophysical techniques such as differential scanning calorimetry (DSC) to study phase transition behavior or fluorescence anisotropy to assess lipid packing. Additionally, small-angle X-ray scattering (SAXS) can reveal structural changes in lipid bilayers when sterols or other phospholipids are introduced. For example, studies have utilized 16:0/18:2 PE to evaluate sterol-phospholipid affinity and lateral phase separation in model membranes . Ensure lipid vesicles are prepared using solvent evaporation and extrusion methods to achieve homogeneous unilamellar vesicles.
Q. What analytical methods are recommended for assessing the purity and structural integrity of this compound?
Level: Basic
Methodological Answer:
High-performance liquid chromatography (HPLC) coupled with evaporative light scattering detection (ELSD) or mass spectrometry (LC-MS/MS) is critical for purity analysis. Nuclear magnetic resonance (NMR) spectroscopy (¹H, ¹³C, and ³¹P) can confirm structural integrity, particularly the sn-1 and sn-2 acyl chain positions. Batch-specific certificates of analysis (CoA) from suppliers should be reviewed to verify fatty acid composition and absence of oxidation products . For reproducible results, store the compound at -20°C under inert gas to prevent hydrolysis or oxidation.
Q. How should experiments be designed to study the hydrolysis of this compound by secretory phospholipase A₂ (sPLA₂)?
Level: Advanced
Methodological Answer:
Design assays using purified sPLA₂ isoforms (e.g., group IIA) in buffer systems with optimized Ca²⁺ concentrations (1–5 mM), as Ca²⁺ is required for enzymatic activity. Monitor hydrolysis kinetics via thin-layer chromatography (TLC) or mass spectrometry to detect linoleic acid release. Note that sPLA₂ lacks specificity for arachidonate but hydrolyzes 16:0/18:2 PE efficiently, making it a suitable substrate for studying broad-spectrum phospholipase activity . Include controls with inhibitors like LY311727 to validate enzyme specificity.
Q. What experimental approaches are used to analyze the upregulation of this compound in cancer metabolomic studies?
Level: Advanced
Methodological Answer:
Liquid chromatography-mass spectrometry (LC-MS/MS)-based lipidomics is the gold standard. Extract lipids from tumor tissues using chloroform-methanol (2:1 v/v), and employ targeted MRM (multiple reaction monitoring) for quantification. Normalize data to protein content or internal standards (e.g., deuterated PE species). In endometrial cancer, 16:0/18:2 PE was found upregulated, suggesting its role in membrane remodeling during carcinogenesis. Pair lipidomic data with transcriptomic analysis to link elevated levels to pathways like phospholipase A₂ or de novo lipid synthesis .
Q. How can contradictions in reported oxidation pathways of this compound be resolved?
Level: Advanced
Methodological Answer:
Contradictions often arise from varying experimental conditions (e.g., oxidant types, pH). Use controlled oxidation assays with defined oxidants (e.g., Fe²⁺/H₂O₂ for Fenton reactions) and monitor products via LC-MS. Compare results with phospholipase-driven hydrolysis to differentiate oxidation from enzymatic degradation. For example, linoleoyl oxidation generates hydroperoxides, which can be quantified using thiobarbituric acid-reactive substances (TBARS) assays. Cross-validate findings with isotopic labeling to track carbon sources in degradation products .
Q. What protocols are recommended for preparing lipid vesicles containing this compound?
Level: Basic
Methodological Answer:
Use the film hydration method : Dissolve 16:0/18:2 PE in chloroform, evaporate solvent under nitrogen to form a thin film, and hydrate with buffer (e.g., Tris-HCl, pH 7.4). Sonicate or extrude through polycarbonate membranes (100 nm pores) to create unilamellar vesicles. Characterize size distribution via dynamic light scattering (DLS). For mixed membranes, combine with cholesterol or other phospholipids at defined molar ratios and validate homogeneity using fluorescence quenching assays .
Q. How can researchers quantify trace levels of this compound in complex biological matrices?
Level: Advanced
Methodological Answer:
Implement a solid-phase extraction (SPE) step using C18 cartridges to enrich phospholipids prior to LC-MS/MS analysis. Use a reverse-phase C18 column with gradient elution (mobile phase: acetonitrile/water with 0.1% formic acid). Quantify using external calibration curves with synthetic 16:0/18:2 PE standards. Address matrix effects by spiking isotopically labeled internal standards (e.g., ¹³C-labeled PE). Document recovery rates and limit of detection (LOD) to ensure reproducibility .
Q. What strategies differentiate the metabolic pathways of this compound from other phosphatidylethanolamines?
Level: Advanced
Methodological Answer:
Use stable isotope tracing (e.g., ¹³C-labeled linoleate) to track incorporation into 16:0/18:2 PE. Combine with siRNA knockdown of enzymes like PLA₂ or lysoPLD to identify pathway dependencies. For example, inhibition of PLA₂ reduces 16:0/18:2 PE hydrolysis, linking it to anandamide biosynthesis. Compare kinetic parameters (Km, Vmax) of phospholipases using substrate-specific assays. Cross-reference lipidomic datasets with enzyme activity profiles to establish metabolic flux .
特性
IUPAC Name |
[(2R)-1-[2-aminoethoxy(hydroxy)phosphoryl]oxy-3-hexadecanoyloxypropan-2-yl] (9Z,12Z)-octadeca-9,12-dienoate | |
---|---|---|
Source | PubChem | |
URL | https://pubchem.ncbi.nlm.nih.gov | |
Description | Data deposited in or computed by PubChem | |
InChI |
InChI=1S/C39H74NO8P/c1-3-5-7-9-11-13-15-17-18-20-22-24-26-28-30-32-39(42)48-37(36-47-49(43,44)46-34-33-40)35-45-38(41)31-29-27-25-23-21-19-16-14-12-10-8-6-4-2/h11,13,17-18,37H,3-10,12,14-16,19-36,40H2,1-2H3,(H,43,44)/b13-11-,18-17-/t37-/m1/s1 | |
Source | PubChem | |
URL | https://pubchem.ncbi.nlm.nih.gov | |
Description | Data deposited in or computed by PubChem | |
InChI Key |
HBZNVZIRJWODIB-NHCUFCNUSA-N | |
Source | PubChem | |
URL | https://pubchem.ncbi.nlm.nih.gov | |
Description | Data deposited in or computed by PubChem | |
Canonical SMILES |
CCCCCCCCCCCCCCCC(=O)OCC(COP(=O)(O)OCCN)OC(=O)CCCCCCCC=CCC=CCCCCC | |
Source | PubChem | |
URL | https://pubchem.ncbi.nlm.nih.gov | |
Description | Data deposited in or computed by PubChem | |
Isomeric SMILES |
CCCCCCCCCCCCCCCC(=O)OC[C@H](COP(=O)(O)OCCN)OC(=O)CCCCCCC/C=C\C/C=C\CCCCC | |
Source | PubChem | |
URL | https://pubchem.ncbi.nlm.nih.gov | |
Description | Data deposited in or computed by PubChem | |
Molecular Formula |
C39H74NO8P | |
Source | PubChem | |
URL | https://pubchem.ncbi.nlm.nih.gov | |
Description | Data deposited in or computed by PubChem | |
DSSTOX Substance ID |
DTXSID30709973 | |
Record name | 1-Palmitoyl-2-linoleoyl-sn-glycero-3-phosphoethanolamine | |
Source | EPA DSSTox | |
URL | https://comptox.epa.gov/dashboard/DTXSID30709973 | |
Description | DSSTox provides a high quality public chemistry resource for supporting improved predictive toxicology. | |
Molecular Weight |
716.0 g/mol | |
Source | PubChem | |
URL | https://pubchem.ncbi.nlm.nih.gov | |
Description | Data deposited in or computed by PubChem | |
Physical Description |
Solid | |
Record name | PE(16:0/18:2(9Z,12Z)) | |
Source | Human Metabolome Database (HMDB) | |
URL | http://www.hmdb.ca/metabolites/HMDB0008928 | |
Description | The Human Metabolome Database (HMDB) is a freely available electronic database containing detailed information about small molecule metabolites found in the human body. | |
Explanation | HMDB is offered to the public as a freely available resource. Use and re-distribution of the data, in whole or in part, for commercial purposes requires explicit permission of the authors and explicit acknowledgment of the source material (HMDB) and the original publication (see the HMDB citing page). We ask that users who download significant portions of the database cite the HMDB paper in any resulting publications. | |
CAS No. |
26662-95-3 | |
Record name | 1-Palmitoyl-2-linoleoyl-sn-glycero-3-phosphoethanolamine | |
Source | CAS Common Chemistry | |
URL | https://commonchemistry.cas.org/detail?cas_rn=26662-95-3 | |
Description | CAS Common Chemistry is an open community resource for accessing chemical information. Nearly 500,000 chemical substances from CAS REGISTRY cover areas of community interest, including common and frequently regulated chemicals, and those relevant to high school and undergraduate chemistry classes. This chemical information, curated by our expert scientists, is provided in alignment with our mission as a division of the American Chemical Society. | |
Explanation | The data from CAS Common Chemistry is provided under a CC-BY-NC 4.0 license, unless otherwise stated. | |
Record name | 1-Palmitoyl-2-linoleoyl-sn-glycero-3-phosphoethanolamine | |
Source | ChemIDplus | |
URL | https://pubchem.ncbi.nlm.nih.gov/substance/?source=chemidplus&sourceid=0026662953 | |
Description | ChemIDplus is a free, web search system that provides access to the structure and nomenclature authority files used for the identification of chemical substances cited in National Library of Medicine (NLM) databases, including the TOXNET system. | |
Record name | 1-Palmitoyl-2-linoleoyl-sn-glycero-3-phosphoethanolamine | |
Source | EPA DSSTox | |
URL | https://comptox.epa.gov/dashboard/DTXSID30709973 | |
Description | DSSTox provides a high quality public chemistry resource for supporting improved predictive toxicology. | |
Record name | 1-PALMITOYL-2-LINOLEOYL-SN-GLYCERO-3-PHOSPHOETHANOLAMINE | |
Source | FDA Global Substance Registration System (GSRS) | |
URL | https://gsrs.ncats.nih.gov/ginas/app/beta/substances/II22JAF28M | |
Description | The FDA Global Substance Registration System (GSRS) enables the efficient and accurate exchange of information on what substances are in regulated products. Instead of relying on names, which vary across regulatory domains, countries, and regions, the GSRS knowledge base makes it possible for substances to be defined by standardized, scientific descriptions. | |
Explanation | Unless otherwise noted, the contents of the FDA website (www.fda.gov), both text and graphics, are not copyrighted. They are in the public domain and may be republished, reprinted and otherwise used freely by anyone without the need to obtain permission from FDA. Credit to the U.S. Food and Drug Administration as the source is appreciated but not required. | |
Record name | PE(16:0/18:2(9Z,12Z)) | |
Source | Human Metabolome Database (HMDB) | |
URL | http://www.hmdb.ca/metabolites/HMDB0008928 | |
Description | The Human Metabolome Database (HMDB) is a freely available electronic database containing detailed information about small molecule metabolites found in the human body. | |
Explanation | HMDB is offered to the public as a freely available resource. Use and re-distribution of the data, in whole or in part, for commercial purposes requires explicit permission of the authors and explicit acknowledgment of the source material (HMDB) and the original publication (see the HMDB citing page). We ask that users who download significant portions of the database cite the HMDB paper in any resulting publications. | |
Retrosynthesis Analysis
AI-Powered Synthesis Planning: Our tool employs the Template_relevance Pistachio, Template_relevance Bkms_metabolic, Template_relevance Pistachio_ringbreaker, Template_relevance Reaxys, Template_relevance Reaxys_biocatalysis model, leveraging a vast database of chemical reactions to predict feasible synthetic routes.
One-Step Synthesis Focus: Specifically designed for one-step synthesis, it provides concise and direct routes for your target compounds, streamlining the synthesis process.
Accurate Predictions: Utilizing the extensive PISTACHIO, BKMS_METABOLIC, PISTACHIO_RINGBREAKER, REAXYS, REAXYS_BIOCATALYSIS database, our tool offers high-accuracy predictions, reflecting the latest in chemical research and data.
Strategy Settings
Precursor scoring | Relevance Heuristic |
---|---|
Min. plausibility | 0.01 |
Model | Template_relevance |
Template Set | Pistachio/Bkms_metabolic/Pistachio_ringbreaker/Reaxys/Reaxys_biocatalysis |
Top-N result to add to graph | 6 |
Feasible Synthetic Routes
試験管内研究製品の免責事項と情報
BenchChemで提示されるすべての記事および製品情報は、情報提供を目的としています。BenchChemで購入可能な製品は、生体外研究のために特別に設計されています。生体外研究は、ラテン語の "in glass" に由来し、生物体の外で行われる実験を指します。これらの製品は医薬品または薬として分類されておらず、FDAから任何の医療状態、病気、または疾患の予防、治療、または治癒のために承認されていません。これらの製品を人間または動物に体内に導入する形態は、法律により厳格に禁止されています。これらのガイドラインに従うことは、研究と実験において法的および倫理的な基準の遵守を確実にするために重要です。