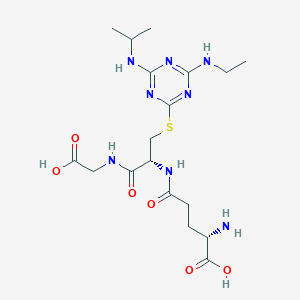
Atrazine glutathione conjugate
説明
Atrazine glutathione conjugate (GS-atrazine) is a product of the detoxification of the herbicide atrazine, which is achieved through a process known as glutathione conjugation . This process occurs in the leaf tissue of corn (Zea mays, L.) and represents a major detoxification mechanism . The identification of GS-atrazine is the first example of glutathione conjugation as a biotransformation mechanism of a pesticide in plants .
Synthesis Analysis
The synthesis of Atrazine glutathione conjugate is facilitated by the enzyme glutathione S-transferase . This enzyme detoxifies atrazine by catalyzing the formation of GS-atrazine . In human and murine models, only hGSTP1-1 and mGSTP1-1 respectively, display significant activity towards atrazine .Molecular Structure Analysis
The molecular structure of Atrazine glutathione conjugate is complex. Docking studies of the atrazine-GST conjugate in the hGSTP1-1 substrate-binding site were used to elucidate a basis for the dramatic difference in activity between mouse GSTP1-1 and GSTP2-2 .Chemical Reactions Analysis
The chemical reaction involved in the formation of Atrazine glutathione conjugate is a detoxification process facilitated by the enzyme glutathione S-transferase . This enzyme catalyzes the formation of GS-atrazine, thereby detoxifying atrazine .科学的研究の応用
Herbicide Resistance in Plants
Atrazine glutathione conjugate plays a significant role in conferring resistance to the herbicide atrazine in plants . For instance, in Palmer amaranth, a troublesome weed, the plants rapidly conjugate atrazine via glutathione S-transferase (GST) activity . This rapid detoxification confers a high level of resistance to atrazine .
Selectivity in Corn
The primary factor for atrazine selectivity in corn (Zea mays) is the activity of a soluble enzyme, glutathione S-transferase, which detoxifies atrazine by catalyzing the formation of an atrazine-glutathione conjugate . This detoxification pathway contributes to the total resistance of corn to atrazine .
Detoxification of Anticancer Drugs
Glutathione usually combines with anticancer drugs and/or their metabolites to form more polar and water-soluble glutathione S-conjugates . These conjugates are readily excreted outside the body, decreasing the likelihood that a xenobiotic will react with cellular targets .
Protection Against Toxic Organic Halide Compounds
Some plants protect themselves against toxic organic halide compounds with a mechanism similar to that known to exist in animals . This mechanism involves the activity of glutathione S-transferase, which detoxifies these compounds by catalyzing the formation of glutathione conjugates .
Non-enzymatic Detoxification Pathway
In addition to the enzymatic detoxification pathway mediated by glutathione S-transferase, there is also a non-enzymatic detoxification pathway that contributes to the total resistance of corn to atrazine . This pathway involves the benzoxazinone-catalyzed hydrolysis of atrazine to hydroxyatrazine .
Potential Role in Human Health
While not directly related to “Atrazine glutathione conjugate”, it’s worth noting that glutathione and glutathione conjugates play crucial roles in human health . They are involved in protecting against various diseases and conditions, including lung inflammation, asthma, virally induced diseases, cancers, alcoholic liver diseases, cystic fibrosis, and diabetes .
将来の方向性
While specific future directions are not mentioned in the search results, the study of Atrazine glutathione conjugate contributes to our understanding of how plants detoxify pesticides. This could have implications for improving crop resistance to pesticides and reducing environmental contamination .
特性
IUPAC Name |
(2S)-2-amino-5-[[(2R)-1-(carboxymethylamino)-3-[[4-(ethylamino)-6-(propan-2-ylamino)-1,3,5-triazin-2-yl]sulfanyl]-1-oxopropan-2-yl]amino]-5-oxopentanoic acid | |
---|---|---|
Source | PubChem | |
URL | https://pubchem.ncbi.nlm.nih.gov | |
Description | Data deposited in or computed by PubChem | |
InChI |
InChI=1S/C18H30N8O6S/c1-4-20-16-24-17(22-9(2)3)26-18(25-16)33-8-11(14(30)21-7-13(28)29)23-12(27)6-5-10(19)15(31)32/h9-11H,4-8,19H2,1-3H3,(H,21,30)(H,23,27)(H,28,29)(H,31,32)(H2,20,22,24,25,26)/t10-,11-/m0/s1 | |
Source | PubChem | |
URL | https://pubchem.ncbi.nlm.nih.gov | |
Description | Data deposited in or computed by PubChem | |
InChI Key |
WDLCRTLULMGVMR-QWRGUYRKSA-N | |
Source | PubChem | |
URL | https://pubchem.ncbi.nlm.nih.gov | |
Description | Data deposited in or computed by PubChem | |
Canonical SMILES |
CCNC1=NC(=NC(=N1)SCC(C(=O)NCC(=O)O)NC(=O)CCC(C(=O)O)N)NC(C)C | |
Source | PubChem | |
URL | https://pubchem.ncbi.nlm.nih.gov | |
Description | Data deposited in or computed by PubChem | |
Isomeric SMILES |
CCNC1=NC(=NC(=N1)SC[C@@H](C(=O)NCC(=O)O)NC(=O)CC[C@@H](C(=O)O)N)NC(C)C | |
Source | PubChem | |
URL | https://pubchem.ncbi.nlm.nih.gov | |
Description | Data deposited in or computed by PubChem | |
Molecular Formula |
C18H30N8O6S | |
Source | PubChem | |
URL | https://pubchem.ncbi.nlm.nih.gov | |
Description | Data deposited in or computed by PubChem | |
DSSTOX Substance ID |
DTXSID60947301 | |
Record name | 5-({1-[(Carboxymethyl)imino]-3-({4-(ethylimino)-6-[(propan-2-yl)imino]-1,4,5,6-tetrahydro-1,3,5-triazin-2-yl}sulfanyl)-1-hydroxypropan-2-yl}imino)-5-hydroxynorvaline | |
Source | EPA DSSTox | |
URL | https://comptox.epa.gov/dashboard/DTXSID60947301 | |
Description | DSSTox provides a high quality public chemistry resource for supporting improved predictive toxicology. | |
Molecular Weight |
486.5 g/mol | |
Source | PubChem | |
URL | https://pubchem.ncbi.nlm.nih.gov | |
Description | Data deposited in or computed by PubChem | |
Product Name |
Atrazine glutathione conjugate | |
CAS RN |
24429-05-8 | |
Record name | Glutathione S-atrazine | |
Source | ChemIDplus | |
URL | https://pubchem.ncbi.nlm.nih.gov/substance/?source=chemidplus&sourceid=0024429058 | |
Description | ChemIDplus is a free, web search system that provides access to the structure and nomenclature authority files used for the identification of chemical substances cited in National Library of Medicine (NLM) databases, including the TOXNET system. | |
Record name | Glutathione S-atrazine | |
Source | DrugBank | |
URL | https://www.drugbank.ca/drugs/DB02146 | |
Description | The DrugBank database is a unique bioinformatics and cheminformatics resource that combines detailed drug (i.e. chemical, pharmacological and pharmaceutical) data with comprehensive drug target (i.e. sequence, structure, and pathway) information. | |
Explanation | Creative Common's Attribution-NonCommercial 4.0 International License (http://creativecommons.org/licenses/by-nc/4.0/legalcode) | |
Record name | 5-({1-[(Carboxymethyl)imino]-3-({4-(ethylimino)-6-[(propan-2-yl)imino]-1,4,5,6-tetrahydro-1,3,5-triazin-2-yl}sulfanyl)-1-hydroxypropan-2-yl}imino)-5-hydroxynorvaline | |
Source | EPA DSSTox | |
URL | https://comptox.epa.gov/dashboard/DTXSID60947301 | |
Description | DSSTox provides a high quality public chemistry resource for supporting improved predictive toxicology. | |
Retrosynthesis Analysis
AI-Powered Synthesis Planning: Our tool employs the Template_relevance Pistachio, Template_relevance Bkms_metabolic, Template_relevance Pistachio_ringbreaker, Template_relevance Reaxys, Template_relevance Reaxys_biocatalysis model, leveraging a vast database of chemical reactions to predict feasible synthetic routes.
One-Step Synthesis Focus: Specifically designed for one-step synthesis, it provides concise and direct routes for your target compounds, streamlining the synthesis process.
Accurate Predictions: Utilizing the extensive PISTACHIO, BKMS_METABOLIC, PISTACHIO_RINGBREAKER, REAXYS, REAXYS_BIOCATALYSIS database, our tool offers high-accuracy predictions, reflecting the latest in chemical research and data.
Strategy Settings
Precursor scoring | Relevance Heuristic |
---|---|
Min. plausibility | 0.01 |
Model | Template_relevance |
Template Set | Pistachio/Bkms_metabolic/Pistachio_ringbreaker/Reaxys/Reaxys_biocatalysis |
Top-N result to add to graph | 6 |
Feasible Synthetic Routes
Q & A
Q1: Are there differences in atrazine detoxification rates among plant species?
A: Yes, research indicates significant variations in atrazine degradation rates across different plant species. Studies have shown that atrazine-tolerant species, including corn, metabolize atrazine primarily through glutathione conjugation. [, ] Conversely, susceptible species exhibit lower GST activity, leading to slower detoxification and increased sensitivity to the herbicide. [] This difference in metabolic rates directly influences the level of atrazine tolerance observed in various plant species.
Q2: Beyond glutathione conjugation, are there other mechanisms of atrazine detoxification in plants?
A: While glutathione conjugation plays a primary role in atrazine detoxification, other metabolic pathways contribute as well. Research has identified N-dealkylation and 2-hydroxylation as additional detoxification mechanisms in various grass species. [] These pathways involve modifying the atrazine molecule, ultimately reducing its toxicity. The relative importance of each pathway can vary depending on the plant species.
試験管内研究製品の免責事項と情報
BenchChemで提示されるすべての記事および製品情報は、情報提供を目的としています。BenchChemで購入可能な製品は、生体外研究のために特別に設計されています。生体外研究は、ラテン語の "in glass" に由来し、生物体の外で行われる実験を指します。これらの製品は医薬品または薬として分類されておらず、FDAから任何の医療状態、病気、または疾患の予防、治療、または治癒のために承認されていません。これらの製品を人間または動物に体内に導入する形態は、法律により厳格に禁止されています。これらのガイドラインに従うことは、研究と実験において法的および倫理的な基準の遵守を確実にするために重要です。