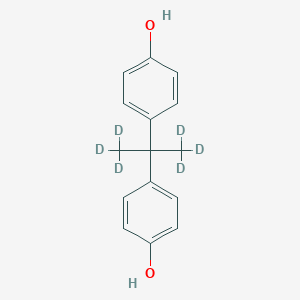
Bisphenol A-d6
概要
説明
Bisphenol A-d6 (BPA-d6; CAS 86588-58-1) is a deuterated isotopologue of Bisphenol A (BPA), where six hydrogen atoms in the methyl groups are replaced with deuterium (²H) . Its molecular formula is C₁₅H₁₆O₂, with a molecular weight of 234.32 g/mol . BPA-d6 is primarily used as an internal standard in analytical chemistry for the precise quantification of BPA and its analogs in biological and environmental matrices via liquid chromatography-mass spectrometry (LC-MS) . Its deuterated structure minimizes interference during analysis, enabling accurate detection of native BPA in complex samples .
準備方法
Synthetic Routes for Bisphenol A-d6
Acid-Catalyzed Condensation with Deuterated Acetone
The foundational method for BPA-d6 synthesis adapts the classical acid-catalyzed condensation of phenol with acetone, substituting acetone with its deuterated analog, acetone-d6. This approach ensures the incorporation of six deuterium atoms at the isopropylidene bridge (C(CH₃)₂ → C(CD₃)₂).
Reaction Conditions
-
Catalyst: Homogeneous HCl or heterogeneous ion-exchange resins (e.g., sulfonated polystyrene-divinylbenzene) .
-
Temperature: 50–65°C to optimize reaction kinetics while minimizing side products .
-
Molar Ratio: Phenol-to-acetone-d6 ratios of 8:1 to 10:1 ensure excess phenol drives the reaction toward BPA-d6 formation .
Mechanism
-
Protonation: Acetone-d6 is protonated by HCl, forming a carbocation intermediate.
-
Electrophilic Aromatic Substitution: Phenol attacks the carbocation, forming a tertiary carbocation.
-
Rearomatization: Loss of a proton regenerates the aromatic ring, yielding BPA-d6 .
Challenges
-
Isomer Formation: The reaction produces para,para′- (p,p′), ortho,para′- (o,p′), and ortho,ortho′- (o,o′) isomers. Catalytic systems with thiol promoters (e.g., 3-mercaptopropionic acid) enhance p,p′-selectivity to >97% .
-
Deuterium Loss: Elevated temperatures (>70°C) risk H-D exchange, necessitating strict temperature control .
Multi-Step Swelling and Polymerization
A novel method for deuterated BPA derivatives involves molecularly imprinted polymers (MIPs) for selective synthesis. While originally developed for BPA-d16, this approach is adaptable to BPA-d6 .
Procedure
-
Template Immobilization: BPA-d6 is embedded in a polymer matrix using 4-vinylpyridine (functional monomer) and ethylene glycol dimethacrylate (cross-linker) .
-
Hydrophilic Modification: Glycerol dimethacrylate and monomethacrylate are added to enhance aqueous compatibility .
-
Selective Polymerization: Radical initiation (e.g., azobisisobutyronitrile) forms a porous network with high affinity for BPA-d6 .
Advantages
Catalytic Systems and Reaction Optimization
Homogeneous vs. Heterogeneous Catalysis
Homogeneous Catalysts (HCl)
Heterogeneous Catalysts (Ion-Exchange Resins)
Solvent Systems
-
Phenol as Solvent: Acts as both reactant and solvent, simplifying purification .
-
Aqueous-Methanol Mixtures: Used in MIP-based synthesis to stabilize intermediates .
Purification and Characterization
Crystallization Techniques
-
Adduct Crystallization: BPA-d6 forms a 1:1 adduct with phenol, isolated via cooling crystallization (0–5°C) .
-
Melt Crystallization: Purifies crude BPA-d6 to >99.9% purity by removing cyclic dimers and chromans .
Chromatographic Methods
-
HPLC-MS/MS: Quantifies BPA-d6 in complex matrices using transitions m/z 257 → 133 (quantifier) and m/z 257 → 115 (qualifier) .
-
UPLC Conditions:
Industrial vs. Laboratory-Scale Production
Industrial Protocols
-
Continuous Reactors: Utilize fixed-bed catalysts for throughputs >1,000 kg/day .
-
Energy Efficiency: Distillation recovers 95% of unreacted phenol for reuse .
Laboratory Synthesis
-
Microscale Reactors: Enable gram-scale production with 92% yield .
-
Quality Control: Matrix blanks and spike recoveries ensure <0.1% contamination .
Challenges and Innovations
Isotopic Dilution
Green Chemistry Approaches
-
Solvent-Free Systems: Ionic liquids (e.g., [BMIM][HSO₄]) reduce waste generation .
-
Biocatalysts: Lipases achieve 88% conversion under mild conditions (pH 7, 30°C) .
Data Summary: Synthesis Parameters
化学反応の分析
Types of Reactions
Bisphenol A-d6 undergoes various chemical reactions, including:
Oxidation: Reaction with oxidizing agents to form quinones.
Reduction: Reaction with reducing agents to form hydroquinones.
Substitution: Electrophilic aromatic substitution reactions, such as halogenation and nitration.
Common Reagents and Conditions
Oxidation: Common oxidizing agents include potassium permanganate and hydrogen peroxide.
Reduction: Common reducing agents include sodium borohydride and lithium aluminum hydride.
Major Products Formed
Oxidation: Formation of bisphenol A quinone.
Reduction: Formation of bisphenol A hydroquinone.
Substitution: Formation of halogenated or nitrated bisphenol A derivatives.
科学的研究の応用
Pharmacokinetic Studies
Pharmacokinetic studies utilizing d6-BPA have been instrumental in understanding the absorption, distribution, metabolism, and excretion (ADME) of BPA in humans and animal models. The following sections summarize key findings from various studies.
2.1. Oral Administration Studies
In a study involving oral administration of d6-BPA to human subjects, researchers observed the pharmacokinetics of BPA following a controlled dose. The results indicated:
- C_max (maximum serum concentration) of total d6-BPA was 1711 nM, with detectable levels of unconjugated d6-BPA appearing within minutes post-ingestion.
- Half-life for total d6-BPA was approximately 6.4 hours, with over 90% excretion as metabolites within 24 hours .
Parameter | Value |
---|---|
C_max | 1711 nM |
Time to C_max | 1.1 hours |
Terminal Half-life | 6.4 hours |
Urinary Excretion | 84-109% |
2.2. Dermal Administration Studies
A separate study examined the effects of dermal exposure to d6-BPA, revealing different pharmacokinetic profiles compared to oral administration:
- Detectable serum levels appeared at 1.4 hours post-exposure.
- The terminal half-lives for total and free d6-BPA were approximately 21.4 hours and 17.6 hours, respectively.
- Only about 2% of the dermal dose became systemically available .
Parameter | Value |
---|---|
C_max | 3.26 nM |
Time to C_max | 2.8 hours |
Terminal Half-life | Total: 21.4 h; Free: 17.6 h |
Systemic Availability | ~2% |
Toxicological Assessments
The use of d6-BPA in toxicological studies has provided insights into the potential endocrine-disrupting effects of BPA:
- A feto-maternal toxicokinetic model using d6-BPA was developed to predict fetal exposure levels based on maternal dietary intake.
- The model demonstrated that BPA concentrations in fetal plasma were lower than those in maternal plasma, indicating possible protective mechanisms .
4.1. Environmental Exposure Assessment
A study assessed the environmental impact of BPA through dietary exposure by administering d6-BPA via food items such as cookies:
- The study highlighted that dietary exposure led to significant absorption and rapid metabolism into conjugates.
- The findings emphasized the importance of understanding dietary sources when evaluating BPA exposure risks .
4.2. Human Health Implications
Research on human health implications revealed that d6-BPA could serve as a marker for assessing BPA exposure through various routes:
作用機序
Bisphenol A-d6, like Bisphenol A, exerts its effects primarily through interaction with estrogen receptors. It mimics the action of natural estrogen, binding to estrogen receptors and altering gene expression. This can lead to various biological effects, including endocrine disruption . The molecular pathways involved include the activation of estrogen receptor-mediated transcription and the modulation of various signaling pathways related to cell growth and differentiation .
類似化合物との比較
Structural and Chemical Properties
Table 1: Structural and Physicochemical Comparison
Compound | Structure Modification | Molecular Weight (g/mol) | Key Uses |
---|---|---|---|
BPA (Bisphenol A) | Two phenol groups linked by C(CH₃)₂ | 228.29 | Polycarbonate plastics, epoxy resins |
BPA-d6 | C(CH₃)₂ replaced with C(CD₃)₂ | 234.32 | Internal standard for BPA quantification in analytical studies |
BPS (Bisphenol S) | Sulfone group replaces C(CH₃)₂ | 250.27 | Thermal paper, "BPA-free" plastics |
BPF (Bisphenol F) | Methylene bridge replaces C(CH₃)₂ | 200.23 | Epoxy resins, coatings |
BPAF (Bisphenol AF) | Hexafluoroisopropylidene linkage | 336.24 | High-performance polymers, rubber additives |
- Key Insight: Deuterated analogs like BPA-d6 exhibit nearly identical chemical behavior to BPA but with distinct isotopic signatures for traceability . BPS and BPF, despite structural differences, retain endocrine-disrupting properties due to conserved phenolic groups .
Endocrine-Disrupting Activity
Table 2: Hormonal Activity and Toxicity
- Key Insight : BPAF and BPF exhibit estrogenic potencies similar to or greater than BPA, while BPS shows reduced but still significant activity . BPA-d6 is metabolically inert, making it ideal for analytical controls .
Environmental Persistence and Exposure
- BPA : Detected in 93% of human urine samples (geometric mean: 2.6 µg/L) due to widespread use in food packaging .
- BPA-d6 : Rarely found in the environment; used experimentally to track BPA degradation pathways .
- BPS/BPF/BPAF : Increasingly detected in indoor dust (BPS: 0.1–120 ng/g) and surface water (BPF: up to 14 ng/L) as industries phase out BPA .
Analytical Detection Methods
- BPA-d6 : Optimized for LC-MS/MS with a detection limit of 0.01 ng/mL in serum .
- BPA Analogs : Require multi-residue LC-MS methods due to structural diversity; BPAF and BPF often co-elute, necessitating high-resolution instruments .
Regulatory Status
Compound | EU SVHC Listing (ECHA) | U.S. FDA Status |
---|---|---|
BPA | Yes (REACH Annex XIV) | Restricted in baby bottles |
BPA-d6 | Exempt | Not regulated |
BPS/BPF | Under review | Generally recognized as safe (GRAS) |
- Key Insight : Regulatory frameworks lag behind scientific evidence; BPA substitutes like BPAF and BPF lack comprehensive safety assessments .
Critical Research Findings
- Toxicity Equivalence : BPAF induces testicular feminization in chicken embryos at 210 nmol/g egg, mirroring BPA’s effects .
- Metabolic Differences : BPA-d6’s deuterium substitution reduces metabolic clearance by cytochrome P450 enzymes, aiding in pharmacokinetic studies .
- Human Health Risks : Cohort studies link urinary BPS/BPF levels to childhood obesity (OR: 1.23–1.49 per log-unit increase) .
生物活性
Bisphenol A-d6 (d6-BPA) is a deuterated analog of bisphenol A (BPA), commonly used in pharmacokinetic studies to trace the compound's behavior in biological systems without interference from native BPA. This article delves into the biological activity of d6-BPA, highlighting its pharmacokinetics, metabolic pathways, and potential health effects based on recent research findings.
Overview of this compound
Chemical Structure and Properties
d6-BPA is chemically similar to BPA but contains six deuterium atoms, enhancing its stability and allowing for precise tracking in biological studies. Its molecular formula is , with a molecular weight of approximately 242.32 g/mol.
Applications in Research
The primary application of d6-BPA is in pharmacokinetic studies where it serves as a tracer to investigate the metabolism and distribution of BPA in various organisms, including humans. The deuterated form helps differentiate between administered d6-BPA and naturally occurring BPA in biological samples.
Pharmacokinetics of this compound
Pharmacokinetic studies have demonstrated that d6-BPA exhibits distinct absorption, distribution, metabolism, and excretion (ADME) profiles. Key findings include:
- Absorption and Metabolism : Following oral administration (100 µg/kg), d6-BPA is rapidly absorbed and extensively metabolized into glucuronide and sulfate conjugates. Studies show that less than 1% of total d6-BPA remains unconjugated in blood at any time point post-administration .
- Elimination : The elimination half-life of d6-BPA is relatively short, with most metabolites excreted within 24 hours. The primary route of excretion is through urine, highlighting the efficiency of metabolic processing .
Study 1: Human Pharmacokinetics
A study involving healthy volunteers assessed the pharmacokinetics of d6-BPA after oral dosing. Blood and urine samples were collected over three days to analyze the absorption rates and metabolic pathways. Results indicated rapid conjugation reactions, with peak concentrations observed within 2-3 hours post-administration .
Parameter | Value |
---|---|
Dose | 100 µg/kg |
Peak Concentration (Cmax) | 0.272 nM |
Area Under Curve (AUC) | Significant increase noted |
Study 2: Feto-Maternal Transfer
Research utilizing a humanized feto-maternal toxicokinetic model demonstrated that maternal exposure to d6-BPA through dietary sources led to predictable fetal concentrations. This study provided insights into how BPA can cross the placental barrier and accumulate in fetal tissues .
Fetal Plasma Concentration | Maternal Plasma Concentration |
---|---|
Trough: 0.011 ng/L | Trough: 0.014 ng/L |
Peak: 0.023 ng/L | Peak: 0.14 ng/L |
Metabolic Pathways
The metabolism of d6-BPA primarily involves phase II reactions, particularly glucuronidation and sulfation, which facilitate its excretion. Studies have shown that the majority of d6-BPA undergoes rapid conjugation via UDP-glucuronosyltransferases (UGTs), resulting in metabolites that are less biologically active compared to the parent compound .
Health Implications
While d6-BPA is often used as a tracer in studies, its structural similarity to BPA raises concerns regarding potential health effects:
- Endocrine Disruption : Both BPA and its analogs have been implicated in endocrine disruption, affecting hormone signaling pathways in various animal models .
- Oxidative Stress : Research indicates that BPA exposure can lead to mitochondrial dysfunction and increased reactive oxygen species (ROS) production, contributing to cellular damage .
Q & A
Basic Research Questions
Q. What methodological considerations are critical for detecting and quantifying Bisphenol A-d6 in environmental or biological matrices?
- Answer : Use isotope-dilution liquid chromatography-tandem mass spectrometry (LC-MS/MS) with deuterated internal standards (e.g., BPA-d6) to enhance precision and minimize matrix effects. Calibration curves should account for deuterium loss during ionization, and recovery experiments must validate extraction efficiency (e.g., solid-phase extraction for aqueous samples) . Include quality controls (QCs) spiked with BPA-d6 to monitor instrument drift and cross-contamination.
Q. How can researchers standardize protocols for BPA-d6 stability studies under varying pH and temperature conditions?
- Answer : Employ a factorial design to systematically test interactions between pH (e.g., 2–10), temperature (e.g., 4°C–40°C), and storage duration. Use kinetic modeling (e.g., first-order degradation rates) to quantify stability. Include deuterated analogs as internal controls to differentiate degradation from matrix interference . Replicate experiments across multiple batches to assess reproducibility .
Q. What criteria should guide the selection of dependent and independent variables in BPA-d6 metabolic pathway studies?
- Answer : Define independent variables (e.g., enzyme concentrations, incubation time) based on prior in vitro hepatocyte models. Dependent variables (e.g., glucuronidation rates) should align with hypotheses about metabolic clearance. Use ANOVA to isolate variable effects and control for confounding factors like solvent polarity .
Advanced Research Questions
Q. How can factorial design resolve contradictory data on BPA-d6’s endocrine-disrupting effects across cell lines?
- Answer : Implement a 2×2×2 factorial design to test interactions between cell type (e.g., MCF-7 vs. HepG2), dose (e.g., 1 nM–1 µM), and exposure duration. Normalize responses to vehicle controls and use pathway-specific biomarkers (e.g., ERα activation). Apply meta-analysis to reconcile discrepancies, weighting studies by methodological rigor (e.g., blinding, replication) .
Q. What strategies address gaps in understanding BPA-d6’s environmental partitioning behavior compared to non-deuterated analogs?
- Answer : Conduct comparative sorption experiments using soil-water systems spiked with BPA and BPA-d6. Measure log Kow (octanol-water) and Kd (soil-water) values via batch equilibrium. Use isotopic tracing to differentiate abiotic vs. microbial degradation pathways. Theoretical frameworks (e.g., fugacity models) should guide hypothesis testing .
Q. How should researchers design longitudinal studies to assess BPA-d6 bioaccumulation in model organisms?
- Answer : Use a pre-test/post-test control group design with staggered exposure phases. Collect tissue samples (e.g., liver, adipose) at intervals (e.g., 7, 14, 21 days) and analyze via GC-MS. Include a nonequivalent control group exposed to non-deuterated BPA. Apply mixed-effects models to account for individual variability and censored data .
Q. What analytical frameworks validate the reproducibility of BPA-d6 studies in multi-laboratory collaborations?
- Answer : Adopt interlaboratory proficiency testing with harmonized SOPs. Use Z-scores to evaluate data consistency and Mandel’s h/k statistics to identify systematic biases. Share raw data (e.g., chromatograms, calibration curves) in supplementary appendices to enable independent verification .
Q. Methodological Challenges & Data Contradictions
Q. How can researchers reconcile conflicting reports on BPA-d6’s stability in human serum versus synthetic media?
- Answer : Perform side-by-side experiments under identical conditions (e.g., 37°C, pH 7.4) using both matrices. Quantify deuterium exchange via high-resolution mass spectrometry (HRMS). Apply Bland-Altman analysis to identify systematic differences and adjust for matrix-specific protein binding .
Q. What statistical approaches mitigate false positives in high-throughput screening of BPA-d6 metabolites?
- Answer : Use false discovery rate (FDR) correction (e.g., Benjamini-Hochberg) for untargeted metabolomics data. Pair machine learning (e.g., random forests) with manual spectral validation to prioritize novel metabolites. Replicate findings in orthogonal models (e.g., primary hepatocytes vs. recombinant enzymes) .
Q. How should theoretical frameworks (e.g., PBPK modeling) be integrated into BPA-d6 toxicokinetic studies?
- Answer : Develop physiologically based pharmacokinetic (PBPK) models parameterized with in vitro-derived metabolic constants (e.g., Vmax, Km). Validate against in vivo rodent data using Bayesian calibration. Sensitivity analysis should identify critical parameters (e.g., biliary excretion rates) requiring empirical refinement .
特性
IUPAC Name |
4-[1,1,1,3,3,3-hexadeuterio-2-(4-hydroxyphenyl)propan-2-yl]phenol | |
---|---|---|
Source | PubChem | |
URL | https://pubchem.ncbi.nlm.nih.gov | |
Description | Data deposited in or computed by PubChem | |
InChI |
InChI=1S/C15H16O2/c1-15(2,11-3-7-13(16)8-4-11)12-5-9-14(17)10-6-12/h3-10,16-17H,1-2H3/i1D3,2D3 | |
Source | PubChem | |
URL | https://pubchem.ncbi.nlm.nih.gov | |
Description | Data deposited in or computed by PubChem | |
InChI Key |
IISBACLAFKSPIT-WFGJKAKNSA-N | |
Source | PubChem | |
URL | https://pubchem.ncbi.nlm.nih.gov | |
Description | Data deposited in or computed by PubChem | |
Canonical SMILES |
CC(C)(C1=CC=C(C=C1)O)C2=CC=C(C=C2)O | |
Source | PubChem | |
URL | https://pubchem.ncbi.nlm.nih.gov | |
Description | Data deposited in or computed by PubChem | |
Isomeric SMILES |
[2H]C([2H])([2H])C(C1=CC=C(C=C1)O)(C2=CC=C(C=C2)O)C([2H])([2H])[2H] | |
Source | PubChem | |
URL | https://pubchem.ncbi.nlm.nih.gov | |
Description | Data deposited in or computed by PubChem | |
Molecular Formula |
C15H16O2 | |
Source | PubChem | |
URL | https://pubchem.ncbi.nlm.nih.gov | |
Description | Data deposited in or computed by PubChem | |
DSSTOX Substance ID |
DTXSID00584370 | |
Record name | 4,4'-[(~2~H_6_)Propane-2,2-diyl]diphenol | |
Source | EPA DSSTox | |
URL | https://comptox.epa.gov/dashboard/DTXSID00584370 | |
Description | DSSTox provides a high quality public chemistry resource for supporting improved predictive toxicology. | |
Molecular Weight |
234.32 g/mol | |
Source | PubChem | |
URL | https://pubchem.ncbi.nlm.nih.gov | |
Description | Data deposited in or computed by PubChem | |
CAS No. |
86588-58-1 | |
Record name | 4,4'-[(~2~H_6_)Propane-2,2-diyl]diphenol | |
Source | EPA DSSTox | |
URL | https://comptox.epa.gov/dashboard/DTXSID00584370 | |
Description | DSSTox provides a high quality public chemistry resource for supporting improved predictive toxicology. | |
Record name | 86588-58-1 | |
Source | European Chemicals Agency (ECHA) | |
URL | https://echa.europa.eu/information-on-chemicals | |
Description | The European Chemicals Agency (ECHA) is an agency of the European Union which is the driving force among regulatory authorities in implementing the EU's groundbreaking chemicals legislation for the benefit of human health and the environment as well as for innovation and competitiveness. | |
Explanation | Use of the information, documents and data from the ECHA website is subject to the terms and conditions of this Legal Notice, and subject to other binding limitations provided for under applicable law, the information, documents and data made available on the ECHA website may be reproduced, distributed and/or used, totally or in part, for non-commercial purposes provided that ECHA is acknowledged as the source: "Source: European Chemicals Agency, http://echa.europa.eu/". Such acknowledgement must be included in each copy of the material. ECHA permits and encourages organisations and individuals to create links to the ECHA website under the following cumulative conditions: Links can only be made to webpages that provide a link to the Legal Notice page. | |
Retrosynthesis Analysis
AI-Powered Synthesis Planning: Our tool employs the Template_relevance Pistachio, Template_relevance Bkms_metabolic, Template_relevance Pistachio_ringbreaker, Template_relevance Reaxys, Template_relevance Reaxys_biocatalysis model, leveraging a vast database of chemical reactions to predict feasible synthetic routes.
One-Step Synthesis Focus: Specifically designed for one-step synthesis, it provides concise and direct routes for your target compounds, streamlining the synthesis process.
Accurate Predictions: Utilizing the extensive PISTACHIO, BKMS_METABOLIC, PISTACHIO_RINGBREAKER, REAXYS, REAXYS_BIOCATALYSIS database, our tool offers high-accuracy predictions, reflecting the latest in chemical research and data.
Strategy Settings
Precursor scoring | Relevance Heuristic |
---|---|
Min. plausibility | 0.01 |
Model | Template_relevance |
Template Set | Pistachio/Bkms_metabolic/Pistachio_ringbreaker/Reaxys/Reaxys_biocatalysis |
Top-N result to add to graph | 6 |
Feasible Synthetic Routes
試験管内研究製品の免責事項と情報
BenchChemで提示されるすべての記事および製品情報は、情報提供を目的としています。BenchChemで購入可能な製品は、生体外研究のために特別に設計されています。生体外研究は、ラテン語の "in glass" に由来し、生物体の外で行われる実験を指します。これらの製品は医薬品または薬として分類されておらず、FDAから任何の医療状態、病気、または疾患の予防、治療、または治癒のために承認されていません。これらの製品を人間または動物に体内に導入する形態は、法律により厳格に禁止されています。これらのガイドラインに従うことは、研究と実験において法的および倫理的な基準の遵守を確実にするために重要です。