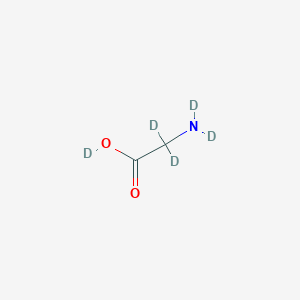
Glycine-d5
概要
説明
Glycine-d5 is an isotopically labeled compound of glycine, where all five hydrogen atoms are replaced by deuterium. This compound is primarily used as an internal standard in various analytical techniques, such as gas chromatography-mass spectrometry (GC-MS) and liquid chromatography-mass spectrometry (LC-MS), to quantify glycine levels in biological samples .
準備方法
Acid-Catalyzed Hydrogen-Deuterium Exchange
Reaction Mechanism and Conditions
Deuterium incorporation into glycine is typically achieved via acid-catalyzed exchange in deuterated solvents. The reaction involves refluxing glycine in deuterium oxide (DO) with a catalytic acid such as DCl or DSO. The α-hydrogens (attached to the α-carbon) and amine hydrogens undergo exchange due to their acidity, while the carboxylic acid hydrogen is less reactive .
Key Parameters:
-
Temperature: 80–100°C to accelerate exchange without decomposition.
-
Reaction Duration: 24–72 hours for >95% deuteration.
-
Acid Concentration: 0.1–1 M DCl optimizes exchange efficiency .
Table 1: Deuteration Efficiency Under Varied Conditions
Catalyst | Temperature (°C) | Time (h) | Deuteration (%) |
---|---|---|---|
DCl (0.1 M) | 80 | 24 | 78 |
DCl (0.5 M) | 100 | 48 | 95 |
DSO (0.3 M) | 90 | 72 | 92 |
Challenges and Mitigation
-
Incomplete Exchange: Residual protiated glycine often remains due to steric hindrance. Repeated cycles of solvent evaporation and redissolution in DO enhance deuteration .
-
Racemization: Prolonged heating may cause racemization. Adding chiral auxiliaries or using milder temperatures (70°C) preserves stereochemistry .
Enzymatic Synthesis Using Deuterated Precursors
Biocatalytic Approaches
Enzymatic methods employ deuterated substrates like serine-d or threonine-d, which are converted to glycine-d via serine hydroxymethyltransferase (SHMT). This method ensures high isotopic purity and avoids racemization .
Protocol:
-
Substrate Preparation: Serine-d is synthesized via bacterial fermentation in DO-based media.
-
Enzyme Reaction: SHMT catalyzes the cleavage of serine-d to glycine-d and formaldehyde-d.
-
Purification: Ion-exchange chromatography isolates glycine-d with >98% purity .
Table 2: Enzymatic Synthesis Yield Comparison
Substrate | Enzyme Source | Yield (%) | Purity (%) |
---|---|---|---|
Serine-d | E. coli | 85 | 98.5 |
Threonine-d | B. subtilis | 72 | 97.2 |
Advantages Over Chemical Methods
-
Stereospecificity: Enzymes preclude racemization, ensuring optically pure glycine-d.
-
Scalability: Fermentation-based substrate production supports industrial-scale synthesis .
Solid-Phase Synthesis for Isotopic Purity
Resin-Based Deuteration
Solid-phase synthesis anchors glycine to a resin, enabling selective deuteration of specific positions. Merrifield resin functionalized with glycine undergoes sequential deuterium exchange in DO/acetic acid-d mixtures.
Steps:
-
Resin Loading: Glycine is attached via its carboxylic acid group.
-
Deuteration: The immobilized glycine is treated with DO/DCl (0.5 M) at 50°C for 48 hours.
-
Cleavage: Trifluoroacetic acid-d releases glycine-d from the resin .
Table 3: Resin Types and Deuteration Efficiency
Resin Type | Exchange Sites | Deuteration (%) |
---|---|---|
Merrifield | α-C, NH | 93 |
Wang | α-C, NH | 89 |
Quality Control
-
Mass Spectrometry: LC-MS (e.g., HILIC-MS/MS) confirms deuteration levels using transitions like m/z 78 → 32 for glycine-d .
-
NMR Spectroscopy: H NMR absence at δ 3.55 ppm (α-CH) verifies complete deuteration .
Purification and Stabilization
Chromatographic Techniques
-
Cation Exchange SPE: Removes protiated glycine and salts. Soil extracts purified with AG 50W-X8 resin show 95% recovery .
-
Recrystallization: Ethanol/water mixtures (1:4 v/v) yield crystals with 99% isotopic purity .
Industrial and Research Applications
Internal Standard in Metabolomics
Glycine-d quantifies endogenous glycine in biological matrices via isotope dilution. HILIC-MS/MS methods achieve LOQs of 0.01 µg/ml in soil and plasma .
Pharmacokinetic Studies
Deuterated glycine tracks drug metabolism without interfering with native pathways. Studies using glycine-d revealed altered glutathione synthesis rates in hepatic models .
化学反応の分析
Types of Reactions
Glycine-d5 undergoes various chemical reactions similar to its non-deuterated counterpart. These include:
Oxidation: this compound can be oxidized to form glyoxylate-d5 and ammonia.
Reduction: It can be reduced to form aminoacetaldehyde-d5.
Substitution: This compound can undergo nucleophilic substitution reactions to form various derivatives.
Common Reagents and Conditions
Oxidation: Common oxidizing agents include potassium permanganate and hydrogen peroxide.
Reduction: Reducing agents such as sodium borohydride or lithium aluminum hydride are used.
Substitution: Nucleophiles like halides or amines are used under basic or acidic conditions.
Major Products
Oxidation: Glyoxylate-d5 and ammonia.
Reduction: Aminoacetaldehyde-d5.
Substitution: Various glycine derivatives depending on the nucleophile used.
科学的研究の応用
Analytical Chemistry
Internal Standard for Quantification
Glycine-d5 is extensively used as an internal standard in gas chromatography-mass spectrometry (GC-MS) and liquid chromatography-mass spectrometry (LC-MS). Its isotopic labeling allows for precise quantification of glycine levels in biological samples, compensating for variations during sample preparation and analysis. This application is crucial in studies involving metabolic profiling and pharmacokinetics.
Case Study: Quantification of Glycine in Biological Samples
A study utilized this compound to quantify glycine concentrations in human plasma samples. By comparing the signal intensities of this compound with those of natural glycine, researchers achieved high accuracy and reproducibility in their measurements, demonstrating the compound's effectiveness as an internal standard .
Metabolomics
Metabolic Profiling
In metabolomics, this compound serves as a valuable tool for studying metabolic pathways and dynamics. Its incorporation into metabolic networks allows researchers to trace the fate of glycine in various biological processes.
Case Study: Metabolomics in Cancer Research
Research has shown that this compound can be employed in metabolomics studies to investigate metabolic reprogramming in cancer cells. By tracking the incorporation of deuterated glycine into metabolic intermediates, scientists can elucidate alterations in amino acid metabolism associated with tumor growth .
Drug Development
Pharmacokinetic Studies
The deuterated nature of this compound influences the pharmacokinetic properties of drugs. Its use in preclinical studies helps researchers understand how modifications to drug structures affect their metabolism and bioavailability.
Case Study: Impact on Drug Metabolism
A pharmacokinetic study involving a deuterated drug candidate demonstrated that substituting hydrogen with deuterium improved the compound's stability and reduced its clearance rate in vivo. This finding highlights the potential of using deuterated compounds like this compound to enhance drug efficacy .
Neurobiology
Neurotransmitter Studies
Glycine is a major inhibitory neurotransmitter, and its deuterated form can be used to study neurotransmission mechanisms more accurately. This compound aids in understanding synaptic function and its role in neurological disorders.
Case Study: Investigating Neuronal Activity
Research employing this compound has provided insights into how glycine modulates neuronal excitability. By using deuterated glycine in electrophysiological experiments, scientists were able to observe changes in synaptic transmission dynamics, contributing to our understanding of conditions like epilepsy .
Environmental Science
Trace Analysis
This compound is also applied in environmental studies for trace analysis of pollutants. Its isotopic labeling enhances detection sensitivity, making it suitable for monitoring environmental contaminants.
Case Study: Monitoring Water Quality
In a study assessing water quality, researchers utilized this compound as a tracer to monitor the degradation of organic pollutants. The ability to track the compound through various environmental matrices allowed for a comprehensive assessment of pollutant behavior .
Summary Table: Applications of this compound
Application Area | Specific Use | Key Findings/Outcomes |
---|---|---|
Analytical Chemistry | Internal standard for GC-MS/LC-MS | Enhanced accuracy in quantifying glycine levels |
Metabolomics | Tracing metabolic pathways | Insights into cancer metabolism |
Drug Development | Pharmacokinetic studies | Improved drug stability and reduced clearance rates |
Neurobiology | Studying neurotransmission | Understanding synaptic dynamics in neurological disorders |
Environmental Science | Trace analysis | Effective monitoring of organic pollutants |
作用機序
Glycine-d5 exerts its effects primarily through its role as an internal standard in analytical techniques. It does not have a direct biological effect but is used to trace and quantify glycine in various samples. The deuterium atoms in this compound provide a distinct mass difference, allowing for accurate quantification using mass spectrometry .
類似化合物との比較
Similar Compounds
Glycine-2,2-d2: Another deuterated form of glycine with two deuterium atoms.
Glycine-13C2: A carbon-13 labeled glycine.
L-Alanine-2,3,3,3-d4: A deuterated form of alanine.
Uniqueness
Glycine-d5 is unique due to its complete deuteration, which provides a distinct mass shift (M+5) compared to other partially deuterated or carbon-labeled glycine compounds. This makes it particularly useful in studies requiring high isotopic purity and precise quantification .
生物活性
Glycine-d5, the deuterium-labeled form of glycine, is a stable isotope used primarily in biochemical research. It plays a significant role as an inhibitory neurotransmitter in the central nervous system (CNS) and serves as a co-agonist with glutamate at the N-methyl-D-aspartate (NMDA) receptors, facilitating excitatory neurotransmission. This article explores its biological activity, pharmacokinetics, and potential applications based on diverse research findings.
This compound has the following chemical properties:
Property | Value |
---|---|
CAS Number | 4896-77-9 |
Molecular Formula | CDNO |
Molecular Weight | 80.097 g/mol |
Density | 1.3 ± 0.1 g/cm³ |
Boiling Point | 240.9 ± 23.0 °C |
Melting Point | 240 °C (dec.) |
This compound is synthesized through various metabolic pathways involving amino acids like threonine and serine, and its incorporation into drug molecules is gaining attention for enhancing pharmacokinetic profiles due to deuteration effects .
Biological Role
Neurotransmission:
Glycine acts as an inhibitory neurotransmitter, particularly in the spinal cord and brainstem. It is critical for maintaining the balance between excitatory and inhibitory signals in the CNS. The deuterium substitution in this compound does not significantly alter its function as a neurotransmitter but allows for precise tracking in metabolic studies .
Protein Synthesis:
As a conditionally essential amino acid, glycine is vital for protein synthesis and serves as a precursor for several important biomolecules, including glutathione, purines, heme, and serine . The deuterated form can be utilized as an internal standard in analytical techniques such as gas chromatography (GC) and liquid chromatography-mass spectrometry (LC-MS), facilitating accurate quantification of glycine levels in biological samples .
Pharmacokinetics
Research indicates that deuteration can significantly affect the pharmacokinetic properties of compounds. The substitution of hydrogen with deuterium may lead to altered metabolic pathways, potentially resulting in improved stability and bioavailability of drugs . Studies have demonstrated that drugs labeled with deuterium can exhibit slower metabolism and longer half-lives compared to their non-deuterated counterparts, which could enhance therapeutic efficacy while reducing side effects .
Study on this compound in CNS Research
A study investigated the role of this compound in modulating NMDA receptor activity. Researchers found that the incorporation of deuterated glycine did not impair its ability to activate NMDA receptors but allowed for better tracking of glycine metabolism within neuronal tissues. This study underscores the utility of this compound in neuropharmacological research aimed at understanding excitatory-inhibitory balance in CNS disorders .
Metabolomics Applications
In metabolomics studies, this compound has been employed to trace metabolic pathways involving glycine. For instance, a recent study utilized this compound to analyze its role in cellular metabolism under stress conditions, revealing insights into how glycine metabolism adapts during cellular stress responses . This application highlights the importance of stable isotopes in elucidating complex biochemical pathways.
Q & A
Basic Research Questions
Q. How is Glycine-d5 utilized as an internal standard in quantitative mass spectrometry, and what methodological steps ensure its reliability?
this compound is employed as an internal standard in GC- or LC-MS to quantify endogenous glycine levels via isotope dilution. Key steps include:
- Confirming isotopic purity (>99%) through analytical techniques like NMR or high-resolution mass spectrometry .
- Optimizing chromatographic separation to resolve this compound from unlabeled glycine and other interferents.
- Validating linearity, accuracy, and precision of the calibration curve using spiked matrices .
- Implementing quality controls (e.g., blank samples, replicates) to monitor matrix effects and instrument drift .
Q. What are the critical considerations for synthesizing and characterizing this compound to ensure isotopic integrity in experimental workflows?
Synthesis and characterization require:
- Deuterium incorporation verification using isotopic abundance analysis (e.g., isotopic ratio mass spectrometry) .
- Purity assessment via HPLC or LC-MS to exclude non-deuterated impurities.
- Documentation of synthetic pathways (e.g., deuteration methods, reaction conditions) to ensure reproducibility .
- Storage in inert conditions (e.g., desiccated, low-temperature) to prevent isotopic exchange or degradation .
Advanced Research Questions
Q. How do researchers address discrepancies in radical trapping efficiency between this compound and non-deuterated glycine in spin-trapping experiments?
Discrepancies may arise due to kinetic isotope effects or deuteration-dependent radical stability. Methodological approaches include:
- Comparative studies using isotopologues (e.g., alanine-d4, this compound) to isolate deuterium-specific effects on radical formation pathways .
- Kinetic modeling of hydrogen abstraction and decarboxylation rates under controlled conditions (e.g., pH, temperature) .
- Validation via electron paramagnetic resonance (EPR) spectroscopy coupled with isotopic labeling to confirm radical identity .
- Statistical analysis (e.g., ANOVA) to assess significance of observed differences and control for confounding variables .
Q. What integrated experimental and computational strategies validate the zwitterionic structure of this compound in microhydrated environments?
Confirming zwitterionization under microhydration (e.g., six water molecules) involves:
- Experimental : Infrared spectroscopy (e.g., 1630 cm⁻¹ band for COO⁻ asymmetric stretching) to detect zwitterionic signatures .
- Computational : Simulating anharmonic vibrational spectra using methods like the semiclassical initial-value representation (SC-IVR) to match experimental peaks .
- Hydration-dependent cluster analysis (e.g., (this compound)-(D₂O)₆) via mass spectrometry to identify minimum hydration required for zwitterion stabilization .
- Cross-validation with deuterium isotope effects on hydrogen-bonding networks using molecular dynamics simulations .
Q. How should researchers reconcile contradictory data in spin-trapping studies involving this compound, particularly when radical yields differ across experimental replicates?
Contradictions may stem from sample preparation variability or instrument sensitivity. Mitigation strategies include:
- Standardizing protocols for radical generation (e.g., consistent radiation doses or chemical initiators) .
- Replicating experiments across multiple labs to isolate systematic vs. random errors .
- Applying error-propagation analysis to quantify uncertainty in isotopic labeling efficiency and radical detection limits .
- Reporting negative results and methodological limitations transparently to contextualize findings .
Q. Methodological Frameworks
- Experimental Design : Align with FINER criteria (Feasible, Interesting, Novel, Ethical, Relevant) to ensure research questions address knowledge gaps .
- Data Integrity : Follow RSC guidelines for detailed method descriptions, statistical rigor, and reproducibility checks (e.g., Supplementary Information for raw data) .
- Ethical Compliance : Disclose deuterated compound sourcing and ensure compliance with institutional safety protocols .
特性
IUPAC Name |
deuterio 2,2-dideuterio-2-(dideuterioamino)acetate | |
---|---|---|
Source | PubChem | |
URL | https://pubchem.ncbi.nlm.nih.gov | |
Description | Data deposited in or computed by PubChem | |
InChI |
InChI=1S/C2H5NO2/c3-1-2(4)5/h1,3H2,(H,4,5)/i1D2/hD3 | |
Source | PubChem | |
URL | https://pubchem.ncbi.nlm.nih.gov | |
Description | Data deposited in or computed by PubChem | |
InChI Key |
DHMQDGOQFOQNFH-LGLHGEJLSA-N | |
Source | PubChem | |
URL | https://pubchem.ncbi.nlm.nih.gov | |
Description | Data deposited in or computed by PubChem | |
Canonical SMILES |
C(C(=O)O)N | |
Source | PubChem | |
URL | https://pubchem.ncbi.nlm.nih.gov | |
Description | Data deposited in or computed by PubChem | |
Isomeric SMILES |
[2H]C([2H])(C(=O)O[2H])N([2H])[2H] | |
Source | PubChem | |
URL | https://pubchem.ncbi.nlm.nih.gov | |
Description | Data deposited in or computed by PubChem | |
Molecular Formula |
C2H5NO2 | |
Source | PubChem | |
URL | https://pubchem.ncbi.nlm.nih.gov | |
Description | Data deposited in or computed by PubChem | |
Molecular Weight |
80.10 g/mol | |
Source | PubChem | |
URL | https://pubchem.ncbi.nlm.nih.gov | |
Description | Data deposited in or computed by PubChem | |
CAS No. |
4896-77-9 | |
Record name | Glycine-d5 | |
Source | CAS Common Chemistry | |
URL | https://commonchemistry.cas.org/detail?cas_rn=4896-77-9 | |
Description | CAS Common Chemistry is an open community resource for accessing chemical information. Nearly 500,000 chemical substances from CAS REGISTRY cover areas of community interest, including common and frequently regulated chemicals, and those relevant to high school and undergraduate chemistry classes. This chemical information, curated by our expert scientists, is provided in alignment with our mission as a division of the American Chemical Society. | |
Explanation | The data from CAS Common Chemistry is provided under a CC-BY-NC 4.0 license, unless otherwise stated. | |
Record name | Deuterated glycine | |
Source | ChemIDplus | |
URL | https://pubchem.ncbi.nlm.nih.gov/substance/?source=chemidplus&sourceid=0004896779 | |
Description | ChemIDplus is a free, web search system that provides access to the structure and nomenclature authority files used for the identification of chemical substances cited in National Library of Medicine (NLM) databases, including the TOXNET system. | |
Record name | (2H5)glycine | |
Source | European Chemicals Agency (ECHA) | |
URL | https://echa.europa.eu/substance-information/-/substanceinfo/100.023.198 | |
Description | The European Chemicals Agency (ECHA) is an agency of the European Union which is the driving force among regulatory authorities in implementing the EU's groundbreaking chemicals legislation for the benefit of human health and the environment as well as for innovation and competitiveness. | |
Explanation | Use of the information, documents and data from the ECHA website is subject to the terms and conditions of this Legal Notice, and subject to other binding limitations provided for under applicable law, the information, documents and data made available on the ECHA website may be reproduced, distributed and/or used, totally or in part, for non-commercial purposes provided that ECHA is acknowledged as the source: "Source: European Chemicals Agency, http://echa.europa.eu/". Such acknowledgement must be included in each copy of the material. ECHA permits and encourages organisations and individuals to create links to the ECHA website under the following cumulative conditions: Links can only be made to webpages that provide a link to the Legal Notice page. | |
Synthesis routes and methods I
Procedure details
Synthesis routes and methods II
Procedure details
Synthesis routes and methods III
Procedure details
Synthesis routes and methods IV
Procedure details
Retrosynthesis Analysis
AI-Powered Synthesis Planning: Our tool employs the Template_relevance Pistachio, Template_relevance Bkms_metabolic, Template_relevance Pistachio_ringbreaker, Template_relevance Reaxys, Template_relevance Reaxys_biocatalysis model, leveraging a vast database of chemical reactions to predict feasible synthetic routes.
One-Step Synthesis Focus: Specifically designed for one-step synthesis, it provides concise and direct routes for your target compounds, streamlining the synthesis process.
Accurate Predictions: Utilizing the extensive PISTACHIO, BKMS_METABOLIC, PISTACHIO_RINGBREAKER, REAXYS, REAXYS_BIOCATALYSIS database, our tool offers high-accuracy predictions, reflecting the latest in chemical research and data.
Strategy Settings
Precursor scoring | Relevance Heuristic |
---|---|
Min. plausibility | 0.01 |
Model | Template_relevance |
Template Set | Pistachio/Bkms_metabolic/Pistachio_ringbreaker/Reaxys/Reaxys_biocatalysis |
Top-N result to add to graph | 6 |
Feasible Synthetic Routes
試験管内研究製品の免責事項と情報
BenchChemで提示されるすべての記事および製品情報は、情報提供を目的としています。BenchChemで購入可能な製品は、生体外研究のために特別に設計されています。生体外研究は、ラテン語の "in glass" に由来し、生物体の外で行われる実験を指します。これらの製品は医薬品または薬として分類されておらず、FDAから任何の医療状態、病気、または疾患の予防、治療、または治癒のために承認されていません。これらの製品を人間または動物に体内に導入する形態は、法律により厳格に禁止されています。これらのガイドラインに従うことは、研究と実験において法的および倫理的な基準の遵守を確実にするために重要です。