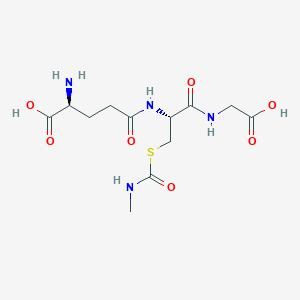
S-(N-Methylcarbamoyl)glutathione
説明
S-(N-Methylcarbamoyl)glutathione is a chemically-reactive glutathione conjugate . It has been isolated from the bile of rats administered methyl isocyanate and characterized, as its N-benzyloxycarbonyl dimethylester derivative, by tandem mass spectrometry .
Synthesis Analysis
The synthesis of S-(N-Methylcarbamoyl)glutathione involves the conjugation of methyl isocyanate with glutathione . This reaction occurs in vivo and results in a reactive S-linked product . The glutathione adduct reacts readily with cysteine in buffered aqueous media (pH 7.4, 37 degrees C), and after 2 hours, 42.5% of the substrate existed in the form of S-(N-methylcarbamoyl)cysteine . The reverse reaction, i.e., between the cysteine adduct and free glutathione, also takes place readily under these conditions .
Molecular Structure Analysis
The molecular formula of S-(N-Methylcarbamoyl)glutathione is C12H20N4O7S . Its molecular weight is 364.38 g/mol . The IUPAC name is (2S)-2-amino-5-[[ (2R)-1-(carboxymethylamino)-3-(methylcarbamoylsulfanyl)-1-oxopropan-2-yl]amino]-5-oxopentanoic acid .
Chemical Reactions Analysis
S-(N-Methylcarbamoyl)glutathione has the ability to donate an N-methylcarbamoyl moiety to the free -SH group of cysteine . This reaction was evaluated in vitro with the aid of a highly specific thermospray LC/MS assay procedure .
科学的研究の応用
Drug Discovery and Development
“S-(N-Methylcarbamoyl)glutathione” plays a significant role in drug discovery and development . It is a metabolite of the antineoplastic agent N-methylformamide . The detection of chemically-reactive, electrophilic metabolites poses a particular problem in the discovery and development of drug candidates in pharmaceutical research . Therefore, understanding the behavior of “S-(N-Methylcarbamoyl)glutathione” can help in the development of safer drugs .
Reactive Metabolite Assessment
“S-(N-Methylcarbamoyl)glutathione” is used in the assessment of reactive metabolites in drug discovery and development . Reactive metabolites contribute to drug-induced liver injury and other immune-mediated serious adverse drug reactions . Therefore, studying “S-(N-Methylcarbamoyl)glutathione” can help in mitigating the risk of idiosyncratic drug toxicity .
Carbamoylation of Peptides and Proteins
“S-(N-Methylcarbamoyl)glutathione” is involved in the carbamoylation of peptides and proteins . This process is crucial in understanding the behavior of certain drugs and their metabolites .
Glutathione Production
While not directly related to “S-(N-Methylcarbamoyl)glutathione”, the production of glutathione (GSH) is an important area of research . As “S-(N-Methylcarbamoyl)glutathione” is a glutathione adduct , understanding the production of GSH can provide insights into the behavior and formation of “S-(N-Methylcarbamoyl)glutathione” and similar compounds .
作用機序
Target of Action
S-(N-Methylcarbamoyl)glutathione is a chemically-reactive glutathione conjugate . It primarily targets glutathione and cysteine in the body . Glutathione is a crucial antioxidant in cells, protecting them from damage by reactive oxygen species. Cysteine is an amino acid that plays a significant role in protein synthesis and metabolic functions .
Mode of Action
The compound has the ability to donate an N-methylcarbamoyl moiety to the free -SH group of cysteine . This interaction results in the formation of S-(N-methylcarbamoyl)cysteine . The reverse reaction, i.e., between the cysteine adduct and free glutathione, also takes place readily .
Biochemical Pathways
The compound is involved in the glutathione metabolic pathway . It is formed as a result of the conjugation of methyl isocyanate with glutathione . This conjugation affords a reactive S-linked product which displays the potential to carbamoylate nucleophilic amino acids .
Pharmacokinetics
It has been isolated from the bile of rats administered methyl isocyanate , suggesting that it may undergo biliary excretion
Result of Action
The various systemic toxicities associated with exposure of animals or humans to methyl isocyanate could be due to the release of the isocyanate from its glutathione conjugate . This suggests that S-(N-Methylcarbamoyl)glutathione may serve as a vehicle for the transport of methyl isocyanate in vivo .
Action Environment
The action of S-(N-Methylcarbamoyl)glutathione is influenced by the physiological environment. For instance, the compound reacted readily with cysteine in buffered aqueous media (pH 7.4, 37 degrees C) . .
特性
IUPAC Name |
(2S)-2-amino-5-[[(2R)-1-(carboxymethylamino)-3-(methylcarbamoylsulfanyl)-1-oxopropan-2-yl]amino]-5-oxopentanoic acid | |
---|---|---|
Source | PubChem | |
URL | https://pubchem.ncbi.nlm.nih.gov | |
Description | Data deposited in or computed by PubChem | |
InChI |
InChI=1S/C12H20N4O7S/c1-14-12(23)24-5-7(10(20)15-4-9(18)19)16-8(17)3-2-6(13)11(21)22/h6-7H,2-5,13H2,1H3,(H,14,23)(H,15,20)(H,16,17)(H,18,19)(H,21,22)/t6-,7-/m0/s1 | |
Source | PubChem | |
URL | https://pubchem.ncbi.nlm.nih.gov | |
Description | Data deposited in or computed by PubChem | |
InChI Key |
ROWIKVIWEBGFSY-BQBZGAKWSA-N | |
Source | PubChem | |
URL | https://pubchem.ncbi.nlm.nih.gov | |
Description | Data deposited in or computed by PubChem | |
Canonical SMILES |
CNC(=O)SCC(C(=O)NCC(=O)O)NC(=O)CCC(C(=O)O)N | |
Source | PubChem | |
URL | https://pubchem.ncbi.nlm.nih.gov | |
Description | Data deposited in or computed by PubChem | |
Isomeric SMILES |
CNC(=O)SC[C@@H](C(=O)NCC(=O)O)NC(=O)CC[C@@H](C(=O)O)N | |
Source | PubChem | |
URL | https://pubchem.ncbi.nlm.nih.gov | |
Description | Data deposited in or computed by PubChem | |
Molecular Formula |
C12H20N4O7S | |
Source | PubChem | |
URL | https://pubchem.ncbi.nlm.nih.gov | |
Description | Data deposited in or computed by PubChem | |
DSSTOX Substance ID |
DTXSID90191537 | |
Record name | S-(N-Methylcarbamoyl)glutathione | |
Source | EPA DSSTox | |
URL | https://comptox.epa.gov/dashboard/DTXSID90191537 | |
Description | DSSTox provides a high quality public chemistry resource for supporting improved predictive toxicology. | |
Molecular Weight |
364.38 g/mol | |
Source | PubChem | |
URL | https://pubchem.ncbi.nlm.nih.gov | |
Description | Data deposited in or computed by PubChem | |
CAS RN |
38126-73-7 | |
Record name | S-(N-Methylcarbamoyl)glutathione | |
Source | ChemIDplus | |
URL | https://pubchem.ncbi.nlm.nih.gov/substance/?source=chemidplus&sourceid=0038126737 | |
Description | ChemIDplus is a free, web search system that provides access to the structure and nomenclature authority files used for the identification of chemical substances cited in National Library of Medicine (NLM) databases, including the TOXNET system. | |
Record name | S-(N-Methylcarbamoyl)glutathione | |
Source | EPA DSSTox | |
URL | https://comptox.epa.gov/dashboard/DTXSID90191537 | |
Description | DSSTox provides a high quality public chemistry resource for supporting improved predictive toxicology. | |
Record name | S-(N-METHYLCARBAMOYL)GLUTATHIONE | |
Source | FDA Global Substance Registration System (GSRS) | |
URL | https://gsrs.ncats.nih.gov/ginas/app/beta/substances/FH7D8R2R6E | |
Description | The FDA Global Substance Registration System (GSRS) enables the efficient and accurate exchange of information on what substances are in regulated products. Instead of relying on names, which vary across regulatory domains, countries, and regions, the GSRS knowledge base makes it possible for substances to be defined by standardized, scientific descriptions. | |
Explanation | Unless otherwise noted, the contents of the FDA website (www.fda.gov), both text and graphics, are not copyrighted. They are in the public domain and may be republished, reprinted and otherwise used freely by anyone without the need to obtain permission from FDA. Credit to the U.S. Food and Drug Administration as the source is appreciated but not required. | |
Retrosynthesis Analysis
AI-Powered Synthesis Planning: Our tool employs the Template_relevance Pistachio, Template_relevance Bkms_metabolic, Template_relevance Pistachio_ringbreaker, Template_relevance Reaxys, Template_relevance Reaxys_biocatalysis model, leveraging a vast database of chemical reactions to predict feasible synthetic routes.
One-Step Synthesis Focus: Specifically designed for one-step synthesis, it provides concise and direct routes for your target compounds, streamlining the synthesis process.
Accurate Predictions: Utilizing the extensive PISTACHIO, BKMS_METABOLIC, PISTACHIO_RINGBREAKER, REAXYS, REAXYS_BIOCATALYSIS database, our tool offers high-accuracy predictions, reflecting the latest in chemical research and data.
Strategy Settings
Precursor scoring | Relevance Heuristic |
---|---|
Min. plausibility | 0.01 |
Model | Template_relevance |
Template Set | Pistachio/Bkms_metabolic/Pistachio_ringbreaker/Reaxys/Reaxys_biocatalysis |
Top-N result to add to graph | 6 |
Feasible Synthetic Routes
Q & A
Q1: How is S-(N-methylcarbamoyl)glutathione formed in vivo?
A1: SMG is formed through a metabolic pathway involving cytochrome P450 2E1 (CYP2E1) [, ]. This enzyme catalyzes the oxidation of NMF and DMF, leading to the formation of reactive intermediates. These intermediates, proposed to be N-alkyl isocyanates, react with glutathione to yield SMG. Studies using deuterated isotopomers of DMF, as well as specific inhibitors and inducers of CYP2E1, have confirmed the involvement of this enzyme in SMG formation [, ].
Q2: Why is S-(N-methylcarbamoyl)glutathione considered a toxic metabolite?
A2: SMG is considered a reactive carbamoylating agent []. This means it can transfer its N-methylcarbamoyl group to nucleophilic sites on proteins and peptides []. This carbamoylation process can disrupt protein structure and function, potentially leading to cellular damage and toxicity.
Q3: What evidence supports the carbamoylating activity of S-(N-methylcarbamoyl)glutathione?
A3: In vitro studies have demonstrated that SMG can covalently modify peptides and proteins []. For instance, incubation of SMG with bovine serum albumin (BSA) resulted in the covalent binding of radiolabeled SMG to the protein []. Importantly, this binding was not prevented by blocking the free thiol group on BSA, suggesting SMG can modify other amino acid residues besides cysteine.
Q4: What analytical techniques are used to identify and quantify S-(N-methylcarbamoyl)glutathione?
A4: Early identification of SMG relied on techniques like fast atom bombardment mass spectrometry (FAB-MS) [, ]. Further advancements led to the use of liquid chromatography coupled with thermospray mass spectrometry (LC/TSP-MS) for the identification and quantification of SMG and its cysteine analogue, SMC []. These techniques allow for sensitive detection and structural characterization of these metabolites in biological samples.
Q5: How does deuterium substitution in N-methylformamide affect its toxicity and metabolism?
A5: Replacing the formyl hydrogen in NMF with deuterium significantly reduces its hepatotoxicity in mice []. This protective effect is attributed to a large kinetic isotope effect observed during the metabolism of deuterated NMF [, ]. This suggests that the rate-limiting step in NMF bioactivation, likely the formyl oxidation catalyzed by CYP2E1, is significantly slowed down by deuterium substitution, thus decreasing the formation of toxic metabolites like SMG.
Q6: Does depletion of glutathione exacerbate the toxicity of N-methylformamide?
A6: Yes, depleting glutathione levels, either chemically with buthionine sulfoximine (BSO) or through NMF metabolism itself, exacerbates NMF cytotoxicity in isolated mouse hepatocytes []. This observation further supports the role of glutathione conjugation as a detoxification pathway for reactive intermediates formed during NMF metabolism.
Q7: Is there evidence of S-(N-methylcarbamoyl)glutathione formation in humans exposed to N,N-dimethylformamide?
A7: While direct evidence from human exposure studies is limited, the metabolic pathways leading to SMG formation are conserved across species, including humans [, ]. In vitro studies using human liver microsomes have shown that DMF is metabolized to HMMF, a precursor to SMG, and that this metabolism is catalyzed by CYP2E1, the same enzyme responsible for SMG formation from NMF [, ]. This suggests that SMG formation is a plausible metabolic pathway for DMF in humans.
試験管内研究製品の免責事項と情報
BenchChemで提示されるすべての記事および製品情報は、情報提供を目的としています。BenchChemで購入可能な製品は、生体外研究のために特別に設計されています。生体外研究は、ラテン語の "in glass" に由来し、生物体の外で行われる実験を指します。これらの製品は医薬品または薬として分類されておらず、FDAから任何の医療状態、病気、または疾患の予防、治療、または治癒のために承認されていません。これらの製品を人間または動物に体内に導入する形態は、法律により厳格に禁止されています。これらのガイドラインに従うことは、研究と実験において法的および倫理的な基準の遵守を確実にするために重要です。