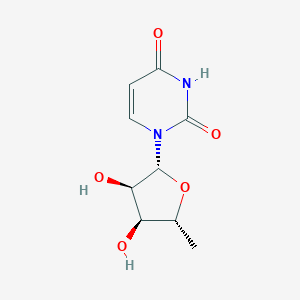
5'-Deoxyuridine
概要
説明
5’-Deoxyuridine is a nucleoside analog that closely resembles the chemical composition of uridine but lacks the 2’ hydroxyl group. It is a derivative of uridine, where the hydroxyl group at the 5’ position is replaced by a hydrogen atom. This compound is significant in various biochemical and pharmacological studies due to its structural similarity to naturally occurring nucleosides.
準備方法
Synthetic Routes and Reaction Conditions
The synthesis of 5’-Deoxyuridine typically involves the selective removal of the 5’ hydroxyl group from uridine. One common method includes the use of protective groups to shield other reactive sites on the uridine molecule, followed by a reduction reaction to remove the hydroxyl group. The reaction conditions often involve the use of reducing agents such as tributyltin hydride in the presence of a radical initiator like azobisisobutyronitrile.
Industrial Production Methods
In industrial settings, the production of 5’-Deoxyuridine can be scaled up using similar synthetic routes but optimized for higher yields and purity. The process may involve continuous flow reactors to ensure consistent reaction conditions and efficient production. The use of advanced purification techniques such as high-performance liquid chromatography (HPLC) ensures the removal of impurities and isolation of the desired product.
化学反応の分析
Types of Reactions
5’-Deoxyuridine undergoes various chemical reactions, including:
Substitution Reactions: It can participate in nucleophilic substitution reactions where the hydrogen atom at the 5’ position is replaced by other functional groups.
Oxidation and Reduction: The compound can be oxidized or reduced under specific conditions to form different derivatives.
Coupling Reactions: It can be involved in coupling reactions such as Suzuki-Miyaura coupling to form more complex nucleoside analogs.
Common Reagents and Conditions
Reducing Agents: Tributyltin hydride, sodium borohydride.
Oxidizing Agents: Potassium permanganate, hydrogen peroxide.
Catalysts: Palladium catalysts for coupling reactions.
Major Products Formed
The major products formed from these reactions include various substituted derivatives of 5’-Deoxyuridine, which can have different biological and chemical properties.
科学的研究の応用
Molecular Biology Applications
5'-Deoxyuridine plays a crucial role in studying DNA synthesis and cell proliferation. It is often used as a precursor in the synthesis of modified nucleotides and as a labeling agent in various assays.
DNA Synthesis Monitoring
This compound can be incorporated into DNA during replication, allowing researchers to monitor DNA synthesis during the S-phase of the cell cycle. This property is exploited in techniques such as:
- Incorporation Studies : this compound is used to trace newly synthesized DNA strands, providing insights into cellular proliferation rates.
- Click Chemistry : The incorporation of this compound can be detected using click chemistry methods, which allow for the attachment of fluorescent tags or biotin for imaging or purification purposes .
Labeling Proliferating Cells
The use of 5-bromo-2'-deoxyuridine (BrdU), a derivative of this compound, has been instrumental in identifying proliferating cells within tissues. BrdU labeling enables researchers to track cell division and fate in various biological contexts, such as cancer research and developmental biology .
Cancer Research Applications
This compound derivatives, particularly 5-fluoro-2'-deoxyuridine (FUdR), are widely utilized in cancer therapy due to their cytotoxic effects on rapidly dividing cells.
Chemotherapeutic Agent
FUdR is an established chemotherapeutic agent used primarily for treating solid tumors and advanced-stage cancers. Its mechanism involves:
- Inhibition of Thymidylate Synthase : FUdR disrupts DNA synthesis by inhibiting thymidylate synthase, leading to cytotoxicity in cancer cells .
- Combination Therapies : Research has shown enhanced efficacy when FUdR is combined with other agents like gemcitabine, improving treatment outcomes for patients with colorectal cancer .
Neurogenesis Research
The application of this compound in neurogenesis studies has provided valuable insights into brain development and regeneration.
Tracing Neuron Development
BrdU labeling has been extensively used to study neurogenesis in various animal models. It allows researchers to:
- Identify Neural Precursors : By incorporating BrdU into dividing neural precursor cells, scientists can trace their lineage and determine their fate during brain development .
- Assess Neurogenesis Under Different Conditions : Studies have shown that environmental factors and treatments can influence neurogenesis rates, which can be monitored using BrdU labeling techniques .
Case Studies and Findings
作用機序
The mechanism of action of 5’-Deoxyuridine involves its incorporation into DNA during replication. Due to its structural similarity to thymidine, it can be incorporated into the DNA strand, leading to the formation of faulty DNA. This can inhibit DNA synthesis and repair, making it useful in studies of cell proliferation and as a potential therapeutic agent.
類似化合物との比較
Similar Compounds
Deoxyuridine: Similar to 5’-Deoxyuridine but retains the 2’ hydroxyl group.
Idoxuridine: A halogenated derivative used as an antiviral agent.
Trifluridine: Another halogenated derivative with antiviral properties.
Uniqueness
5’-Deoxyuridine is unique due to the absence of the 2’ hydroxyl group, which affects its incorporation into DNA and its overall chemical reactivity. This makes it a valuable tool for specific biochemical studies and potential therapeutic applications.
生物活性
5'-Deoxyuridine (5'-dUrd) is a nucleoside analog that has garnered interest in cancer research due to its biological activity, particularly in the context of antitumor effects. This article explores the metabolic pathways, cytotoxicity, and therapeutic implications of 5'-dUrd, drawing on various studies and case reports to provide a comprehensive overview.
This compound is primarily metabolized within cells to produce active metabolites that exert biological effects. The key metabolic pathway involves the conversion of 5'-dUrd to deoxythymidine monophosphate (dTMP), which is subsequently phosphorylated to deoxythymidine triphosphate (dTTP). This conversion is crucial for DNA synthesis and repair.
Key Metabolites and Enzymatic Activity
- Thymidylate Synthase Inhibition : One of the primary mechanisms through which 5'-dUrd exhibits antitumor activity is via the inhibition of thymidylate synthase (TS). This enzyme is essential for dTMP synthesis, and its inhibition leads to a depletion of dTTP, disrupting DNA replication and repair processes.
- Formation of Active Metabolites : Studies have shown that 5'-dUrd can be converted into several active metabolites, including:
- FdUMP : The monophosphate form that forms a complex with TS.
- FdUTP : Incorporates into DNA, leading to strand breaks.
The Km values for nucleoside phosphorylase enzymes indicate the affinity of 5'-dUrd for these metabolic pathways, which is critical for understanding its efficacy in cancer treatment .
Cytotoxicity and Selectivity
Research has demonstrated that 5'-dUrd exhibits selective cytotoxicity towards various human tumor cell lines while showing comparatively lower toxicity towards normal bone marrow stem cells. This selectivity is crucial for minimizing side effects during cancer therapy.
Comparative Cytotoxicity Data
Tumor Cell Line | LD50 (µM) | Comments |
---|---|---|
47-DN Breast Carcinoma | 32 | Highest sensitivity among tested lines |
MCF-7 Breast Carcinoma | 35 | Comparable sensitivity |
MG-63 Osteosarcoma | 41 | Moderate sensitivity |
HCT-8 Colon Tumor | 200 | Intermediate sensitivity |
Colo-357 Pancreatic Tumor | 150 | Intermediate sensitivity |
HL-60 Leukemia | 470 | Lowest sensitivity; poor activity |
Bone Marrow Stem Cells | 580 | Highest LD50 indicating significant toxicity |
The therapeutic ratio calculated from these studies indicates that 5'-dUrd can offer a more favorable balance between efficacy against tumors and safety for normal tissues compared to other fluoropyrimidines like 5-fluorouracil .
Case Studies and Clinical Implications
Several clinical studies have highlighted the potential of 5'-dUrd as an effective treatment option:
- Ehrlich Ascites Tumor Model : In animal studies using Ehrlich ascites tumor cells, 5'-dUrd demonstrated significant antitumor effects with less toxicity compared to other agents. The study reported improved survival rates and reduced tumor growth at various dosages .
- Human Tumor Cell Lines : A comparative study involving multiple human tumor cell lines showed that while 5'-dUrd was less potent than some other fluoropyrimidines, it exhibited a unique profile of selective toxicity that could be beneficial in specific treatment regimens .
特性
IUPAC Name |
1-[(2R,3R,4S,5R)-3,4-dihydroxy-5-methyloxolan-2-yl]pyrimidine-2,4-dione | |
---|---|---|
Source | PubChem | |
URL | https://pubchem.ncbi.nlm.nih.gov | |
Description | Data deposited in or computed by PubChem | |
InChI |
InChI=1S/C9H12N2O5/c1-4-6(13)7(14)8(16-4)11-3-2-5(12)10-9(11)15/h2-4,6-8,13-14H,1H3,(H,10,12,15)/t4-,6-,7-,8-/m1/s1 | |
Source | PubChem | |
URL | https://pubchem.ncbi.nlm.nih.gov | |
Description | Data deposited in or computed by PubChem | |
InChI Key |
WUBAOANSQGKRHF-XVFCMESISA-N | |
Source | PubChem | |
URL | https://pubchem.ncbi.nlm.nih.gov | |
Description | Data deposited in or computed by PubChem | |
Canonical SMILES |
CC1C(C(C(O1)N2C=CC(=O)NC2=O)O)O | |
Source | PubChem | |
URL | https://pubchem.ncbi.nlm.nih.gov | |
Description | Data deposited in or computed by PubChem | |
Isomeric SMILES |
C[C@@H]1[C@H]([C@H]([C@@H](O1)N2C=CC(=O)NC2=O)O)O | |
Source | PubChem | |
URL | https://pubchem.ncbi.nlm.nih.gov | |
Description | Data deposited in or computed by PubChem | |
Molecular Formula |
C9H12N2O5 | |
Source | PubChem | |
URL | https://pubchem.ncbi.nlm.nih.gov | |
Description | Data deposited in or computed by PubChem | |
DSSTOX Substance ID |
DTXSID20332158 | |
Record name | 5'-deoxyuridine | |
Source | EPA DSSTox | |
URL | https://comptox.epa.gov/dashboard/DTXSID20332158 | |
Description | DSSTox provides a high quality public chemistry resource for supporting improved predictive toxicology. | |
Molecular Weight |
228.20 g/mol | |
Source | PubChem | |
URL | https://pubchem.ncbi.nlm.nih.gov | |
Description | Data deposited in or computed by PubChem | |
CAS No. |
15958-99-3 | |
Record name | 5'-deoxyuridine | |
Source | EPA DSSTox | |
URL | https://comptox.epa.gov/dashboard/DTXSID20332158 | |
Description | DSSTox provides a high quality public chemistry resource for supporting improved predictive toxicology. | |
Retrosynthesis Analysis
AI-Powered Synthesis Planning: Our tool employs the Template_relevance Pistachio, Template_relevance Bkms_metabolic, Template_relevance Pistachio_ringbreaker, Template_relevance Reaxys, Template_relevance Reaxys_biocatalysis model, leveraging a vast database of chemical reactions to predict feasible synthetic routes.
One-Step Synthesis Focus: Specifically designed for one-step synthesis, it provides concise and direct routes for your target compounds, streamlining the synthesis process.
Accurate Predictions: Utilizing the extensive PISTACHIO, BKMS_METABOLIC, PISTACHIO_RINGBREAKER, REAXYS, REAXYS_BIOCATALYSIS database, our tool offers high-accuracy predictions, reflecting the latest in chemical research and data.
Strategy Settings
Precursor scoring | Relevance Heuristic |
---|---|
Min. plausibility | 0.01 |
Model | Template_relevance |
Template Set | Pistachio/Bkms_metabolic/Pistachio_ringbreaker/Reaxys/Reaxys_biocatalysis |
Top-N result to add to graph | 6 |
Feasible Synthetic Routes
試験管内研究製品の免責事項と情報
BenchChemで提示されるすべての記事および製品情報は、情報提供を目的としています。BenchChemで購入可能な製品は、生体外研究のために特別に設計されています。生体外研究は、ラテン語の "in glass" に由来し、生物体の外で行われる実験を指します。これらの製品は医薬品または薬として分類されておらず、FDAから任何の医療状態、病気、または疾患の予防、治療、または治癒のために承認されていません。これらの製品を人間または動物に体内に導入する形態は、法律により厳格に禁止されています。これらのガイドラインに従うことは、研究と実験において法的および倫理的な基準の遵守を確実にするために重要です。