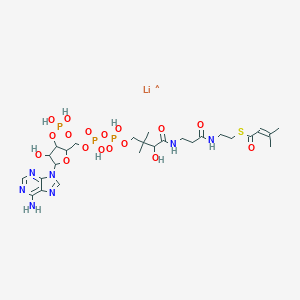
(C5:1) Coenzyme A lithium salt
説明
“(C5:1) Coenzyme A lithium salt” is also known as “Tiglyl coenzyme A (C5:1) lithium salt” or "trans-2-Methyl-2-butenoyl Coenzyme A" . It is a derivative of coenzyme A, a coenzyme known for its role in the synthesis and oxidation of fatty acids .
Molecular Structure Analysis
The molecular formula of “(C5:1) Coenzyme A lithium salt” is C26H43LiN7O17P3S . Its molecular weight is 857.58 .Physical And Chemical Properties Analysis
“(C5:1) Coenzyme A lithium salt” has a molecular weight of 857.58 . The storage temperature is -20°C .科学的研究の応用
Biochemical Research
Tiglyl coenzyme A is often used in biochemical research due to its role as an essential cofactor in enzymatic acetyl transfer reactions . It’s involved in various metabolic processes, including the synthesis and oxidation of fatty acids.
Metabolite Profiling
In metabolomics, Tiglyl coenzyme A can be quantified to provide insights into intracellular metabolic processes . For instance, it can be used in LC-MS/MS-based metabolite profiling for the absolute quantification of intracellular coenzyme A and short-chain acyl-coenzyme A thioesters .
Study of Metabolic Pathways
This compound can be used to study metabolic pathways, particularly those involving coenzyme A and its derivatives . It can help researchers understand how these pathways are regulated and how they contribute to various biological processes.
Industrial Biotechnology
In the field of industrial biotechnology, Tiglyl coenzyme A can be used in the engineering of microbial cell factories . By manipulating specific enzymatic reactions involving this compound, scientists can endow microorganisms with desirable properties for organic acid, amino acid, antibiotics, and vitamin production .
Safety And Hazards
特性
InChI |
InChI=1S/C26H42N7O17P3S.Li/c1-14(2)9-17(35)54-8-7-28-16(34)5-6-29-24(38)21(37)26(3,4)11-47-53(44,45)50-52(42,43)46-10-15-20(49-51(39,40)41)19(36)25(48-15)33-13-32-18-22(27)30-12-31-23(18)33;/h9,12-13,15,19-21,25,36-37H,5-8,10-11H2,1-4H3,(H,28,34)(H,29,38)(H,42,43)(H,44,45)(H2,27,30,31)(H2,39,40,41); | |
---|---|---|
Source | PubChem | |
URL | https://pubchem.ncbi.nlm.nih.gov | |
Description | Data deposited in or computed by PubChem | |
InChI Key |
XFSTZOBMDWRSLY-UHFFFAOYSA-N | |
Source | PubChem | |
URL | https://pubchem.ncbi.nlm.nih.gov | |
Description | Data deposited in or computed by PubChem | |
Canonical SMILES |
[Li].CC(=CC(=O)SCCNC(=O)CCNC(=O)C(C(C)(C)COP(=O)(O)OP(=O)(O)OCC1C(C(C(O1)N2C=NC3=C(N=CN=C32)N)O)OP(=O)(O)O)O)C | |
Source | PubChem | |
URL | https://pubchem.ncbi.nlm.nih.gov | |
Description | Data deposited in or computed by PubChem | |
Molecular Formula |
C26H42LiN7O17P3S | |
Source | PubChem | |
URL | https://pubchem.ncbi.nlm.nih.gov | |
Description | Data deposited in or computed by PubChem | |
DSSTOX Substance ID |
DTXSID90585215 | |
Record name | beta-Methylcrotonyl coenzyme A lithium salt | |
Source | EPA DSSTox | |
URL | https://comptox.epa.gov/dashboard/DTXSID90585215 | |
Description | DSSTox provides a high quality public chemistry resource for supporting improved predictive toxicology. | |
Molecular Weight |
856.6 g/mol | |
Source | PubChem | |
URL | https://pubchem.ncbi.nlm.nih.gov | |
Description | Data deposited in or computed by PubChem | |
Product Name |
(C5:1) Coenzyme A lithium salt | |
CAS RN |
108347-83-7 | |
Record name | beta-Methylcrotonyl coenzyme A lithium salt | |
Source | EPA DSSTox | |
URL | https://comptox.epa.gov/dashboard/DTXSID90585215 | |
Description | DSSTox provides a high quality public chemistry resource for supporting improved predictive toxicology. | |
Record name | β-Methylcrotonyl coenzyme� A lithium salt | |
Source | European Chemicals Agency (ECHA) | |
URL | https://echa.europa.eu/information-on-chemicals | |
Description | The European Chemicals Agency (ECHA) is an agency of the European Union which is the driving force among regulatory authorities in implementing the EU's groundbreaking chemicals legislation for the benefit of human health and the environment as well as for innovation and competitiveness. | |
Explanation | Use of the information, documents and data from the ECHA website is subject to the terms and conditions of this Legal Notice, and subject to other binding limitations provided for under applicable law, the information, documents and data made available on the ECHA website may be reproduced, distributed and/or used, totally or in part, for non-commercial purposes provided that ECHA is acknowledged as the source: "Source: European Chemicals Agency, http://echa.europa.eu/". Such acknowledgement must be included in each copy of the material. ECHA permits and encourages organisations and individuals to create links to the ECHA website under the following cumulative conditions: Links can only be made to webpages that provide a link to the Legal Notice page. | |
Retrosynthesis Analysis
AI-Powered Synthesis Planning: Our tool employs the Template_relevance Pistachio, Template_relevance Bkms_metabolic, Template_relevance Pistachio_ringbreaker, Template_relevance Reaxys, Template_relevance Reaxys_biocatalysis model, leveraging a vast database of chemical reactions to predict feasible synthetic routes.
One-Step Synthesis Focus: Specifically designed for one-step synthesis, it provides concise and direct routes for your target compounds, streamlining the synthesis process.
Accurate Predictions: Utilizing the extensive PISTACHIO, BKMS_METABOLIC, PISTACHIO_RINGBREAKER, REAXYS, REAXYS_BIOCATALYSIS database, our tool offers high-accuracy predictions, reflecting the latest in chemical research and data.
Strategy Settings
Precursor scoring | Relevance Heuristic |
---|---|
Min. plausibility | 0.01 |
Model | Template_relevance |
Template Set | Pistachio/Bkms_metabolic/Pistachio_ringbreaker/Reaxys/Reaxys_biocatalysis |
Top-N result to add to graph | 6 |
Feasible Synthetic Routes
試験管内研究製品の免責事項と情報
BenchChemで提示されるすべての記事および製品情報は、情報提供を目的としています。BenchChemで購入可能な製品は、生体外研究のために特別に設計されています。生体外研究は、ラテン語の "in glass" に由来し、生物体の外で行われる実験を指します。これらの製品は医薬品または薬として分類されておらず、FDAから任何の医療状態、病気、または疾患の予防、治療、または治癒のために承認されていません。これらの製品を人間または動物に体内に導入する形態は、法律により厳格に禁止されています。これらのガイドラインに従うことは、研究と実験において法的および倫理的な基準の遵守を確実にするために重要です。