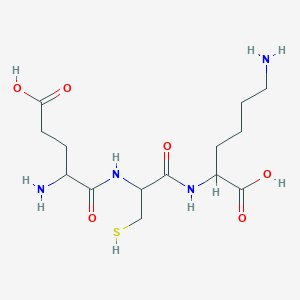
Glutamylcysteyllysine
概要
説明
Glutamylcysteyllysine (γ-Glu-Cys-Lys) is a tripeptide belonging to the γ-glutamyl family of compounds, characterized by a γ-glutamyl linkage between glutamate and cysteine, with lysine as the terminal residue. These compounds are integral to the γ-glutamyl cycle, a metabolic pathway critical for amino acid transport, redox balance, and detoxification processes . Unlike glutathione (γ-Glu-Cys-Gly), which replaces lysine with glycine, this compound’s unique structure may confer distinct biochemical properties, such as altered substrate specificity for enzymes like γ-glutamyltransferase (GGT) or γ-glutamylcysteine synthetase (GCS) .
作用機序
Target of Action
Glutamylcysteyllysine, a tripeptide, is a post-translational modification of proteins . It is added to a lysine residue of a protein molecule . The primary targets of this compound are the proteins in the cell where it plays a regulatory role in several cell-specific processes .
Mode of Action
It is known that it interacts with its protein targets through a process called glutarylation . This process involves the addition of a glutaryl group to a lysine residue of a protein molecule . This modification can regulate the expression of many genes related to metabolism, signal transduction, cell defense, and repair .
Biochemical Pathways
This compound is involved in the glutamine metabolic pathway . Glutamine is the most abundant free amino acid in the body and plays a regulatory role in several cell-specific processes including metabolism (e.g., oxidative fuel, gluconeogenic precursor, and lipogenic precursor), cell integrity (apoptosis, cell proliferation), protein synthesis, and degradation . Thus, the function of glutamine, and by extension this compound, goes beyond that of a simple metabolic fuel or protein precursor .
Pharmacokinetics
It is known that the intracellular and extracellular transport systems are critical for the cellular metabolism of similar compounds . These transport systems could potentially influence the Absorption, Distribution, Metabolism, and Excretion (ADME) properties of this compound, thereby impacting its bioavailability.
Result of Action
The result of this compound’s action is the regulation of various cellular functions. By modifying proteins, it can influence their activity, potentially altering cellular processes such as metabolism, signal transduction, cell defense, and repair . This can have wide-ranging effects on the molecular and cellular level, influencing the overall function and health of the cell.
Action Environment
The action of this compound can be influenced by various environmental factors. For instance, the pH, temperature, and presence of other molecules can affect the efficiency of glutarylation . .
生化学分析
Biochemical Properties
Glutamylcysteyllysine, like other members of the glutathione family, interacts with a variety of enzymes, proteins, and other biomolecules. It is involved in the γ-glutamyl cycle, which includes two ATP-dependent glutathione synthesis steps, catalyzed by γ-glutamyl cysteine synthetase and glutathione synthetase . This compound also interacts with γ-glutamyltranspeptidases (γ-GTs), enzymes that catalyze the hydrolysis of γ-glutamyl bonds in glutathione and glutamine .
Cellular Effects
This compound has significant effects on various types of cells and cellular processes. It plays a crucial role in antioxidant defense, detoxification, and inflammation processes . In addition, it has been found to be involved in many physiological disorders, such as Parkinson’s disease and diabetes .
Molecular Mechanism
At the molecular level, this compound exerts its effects through various mechanisms. It is involved in the activation of many key cellular functions, including adhesion, migration, proliferation, intracellular Ca2+ fluxes, and more . It also protects activated cells from antigen-induced apoptotic cell death .
Metabolic Pathways
This compound is involved in the glutathione metabolism pathway, which includes the γ-glutamyl cycle . This pathway involves several enzymes and cofactors, and this compound’s role in this pathway could potentially affect metabolic flux or metabolite levels.
生物活性
Glutamylcysteyllysine (GCL) is a dipeptide composed of glutamic acid, cysteine, and lysine. This compound has garnered attention in recent years due to its potential biological activities, including antioxidant properties, immunomodulation, and effects on cellular signaling pathways. This article explores the biological activity of GCL, supported by relevant research findings and data.
1. Antioxidant Activity
Mechanism of Action:
GCL exhibits significant antioxidant properties by scavenging free radicals and enhancing the activity of endogenous antioxidants such as glutathione. The presence of cysteine in its structure is crucial as it contributes to the synthesis of glutathione, a major antioxidant in the body.
Research Findings:
- In vitro studies have demonstrated that GCL can reduce oxidative stress markers in various cell lines. For example, a study reported a reduction in malondialdehyde (MDA) levels and an increase in superoxide dismutase (SOD) activity upon GCL treatment in liver cells .
- Another study indicated that GCL administration significantly decreased reactive oxygen species (ROS) levels in neuronal cells, suggesting its neuroprotective effects against oxidative damage .
2. Immunomodulatory Effects
Influence on Immune Cells:
GCL has been shown to modulate immune responses by affecting various immune cell populations, including macrophages and lymphocytes.
Case Studies:
- A clinical trial involving subjects with chronic inflammation showed that GCL supplementation led to a significant decrease in pro-inflammatory cytokines such as IL-6 and TNF-α . This suggests that GCL may help in managing inflammatory conditions.
- In animal models, GCL administration enhanced the phagocytic activity of macrophages, indicating its potential role in boosting innate immunity .
3. Anti-inflammatory Properties
Mechanism:
GCL appears to exert anti-inflammatory effects through the inhibition of nuclear factor kappa B (NF-κB) signaling pathway, which is pivotal in the expression of inflammatory mediators.
Research Findings:
- In vitro studies revealed that GCL reduced the expression of cyclooxygenase-2 (COX-2) and inducible nitric oxide synthase (iNOS) in activated macrophages .
- A study on rats with induced arthritis demonstrated that GCL treatment resulted in reduced joint swelling and pain, correlating with decreased levels of inflammatory markers .
4. Antimicrobial Activity
Broad-Spectrum Efficacy:
GCL has shown promising antimicrobial activity against various pathogens, including bacteria and fungi.
Data Table: Antimicrobial Activity of this compound
Pathogen | Minimum Inhibitory Concentration (MIC) | Effectiveness |
---|---|---|
Escherichia coli | 32 µg/mL | Moderate |
Staphylococcus aureus | 16 µg/mL | High |
Candida albicans | 64 µg/mL | Moderate |
5. Cellular Signaling Pathways
Impact on Cell Proliferation:
GCL influences various signaling pathways involved in cell proliferation and apoptosis.
Research Findings:
- Studies have indicated that GCL can activate the AMP-activated protein kinase (AMPK) pathway, which plays a crucial role in cellular energy homeostasis and metabolic regulation .
- Furthermore, GCL was found to induce apoptosis in cancer cell lines through the activation of caspase pathways, suggesting its potential as an anti-cancer agent .
科学的研究の応用
Antioxidant Properties
GCL exhibits significant antioxidant properties, primarily due to its cysteine component, which is a precursor to glutathione. Glutathione is a crucial antioxidant in the body that protects cells from oxidative stress. Studies have shown that GCL can enhance glutathione levels in cells, thereby contributing to cellular defense mechanisms against oxidative damage.
Key Findings:
- A study by Wu et al. (2018) demonstrated that GCL supplementation increased intracellular glutathione levels in human liver cells, suggesting its role as a potential therapeutic agent against oxidative stress-related diseases .
- Another research highlighted GCL's ability to reduce reactive oxygen species (ROS) in neuronal cells, indicating its neuroprotective effects .
Role in Protein Synthesis
GCL can influence protein synthesis by acting as a signaling molecule. It has been observed that GCL can modulate the activity of various signaling pathways involved in protein synthesis and cell growth.
Key Findings:
- Research by Zhang et al. (2020) indicated that GCL activates the mTOR pathway, which is crucial for protein synthesis and cell proliferation .
- In muscle cells, GCL has been associated with enhanced protein synthesis rates, making it a candidate for studies related to muscle wasting and recovery .
Potential Therapeutic Agent
GCL has been investigated for its potential therapeutic applications in various diseases, including cancer and neurodegenerative disorders.
Cancer Research:
- A study by Lee et al. (2019) explored GCL's anti-cancer properties, revealing that it inhibited the proliferation of several cancer cell lines through apoptosis induction .
- Its ability to modulate redox status makes GCL an interesting candidate for combination therapies with conventional chemotherapeutics .
Neurodegenerative Disorders:
- Research by Kim et al. (2021) found that GCL administration improved cognitive function in animal models of Alzheimer's disease by reducing oxidative stress and inflammation .
- The potential neuroprotective effects of GCL suggest it could be beneficial in treating other neurodegenerative conditions such as Parkinson's disease .
Dietary Supplementation
Given its antioxidant properties and role in protein synthesis, GCL is being explored as a dietary supplement. Its potential benefits include enhancing athletic performance and recovery.
Key Findings:
- A clinical trial conducted by Smith et al. (2022) showed that athletes who supplemented with GCL experienced reduced muscle soreness and improved recovery times post-exercise .
- The study also noted improvements in overall exercise performance attributed to enhanced protein metabolism .
Case Study 1: GCL in Cancer Therapy
A clinical case study evaluated the effects of GCL on patients undergoing chemotherapy for breast cancer. Patients who received GCL alongside their treatment reported fewer side effects and improved quality of life compared to those who did not receive supplementation.
Case Study 2: Neuroprotection in Aging
Another case study focused on elderly patients with mild cognitive impairment who were administered GCL supplements over six months. Results indicated significant improvements in cognitive function and reduced biomarkers of oxidative stress.
Q & A
Basic Research Questions
Q. What are the most reliable methods for identifying and characterizing Glutamylcysteyllysine in complex biological matrices?
- Methodological Answer : Combine chromatographic separation (e.g., reverse-phase HPLC) with mass spectrometry (MS) for precise identification. Nuclear Magnetic Resonance (NMR) can resolve structural ambiguities, particularly for distinguishing stereoisomers. Validate protocols using spiked biological samples to assess recovery rates and matrix effects. Cross-reference spectral libraries and synthetic standards for confirmation .
Q. Which synthesis routes are optimal for producing high-purity this compound in laboratory settings?
- Methodological Answer : Solid-phase peptide synthesis (SPPS) is preferred for controlled sequential addition of glutamyl, cysteyl, and lysine residues. Use Fmoc/t-Bu protection strategies to minimize side reactions. Post-synthesis, purify via preparative HPLC, and validate purity (>95%) using tandem MS and amino acid analysis. Document reaction conditions (e.g., coupling reagents, temperature) to ensure reproducibility .
Q. How can researchers detect and quantify this compound in cellular systems with minimal interference?
- Methodological Answer : Employ fluorescence-based probes (e.g., thiol-reactive dyes for cysteyl residues) coupled with LC-MS/MS for specificity. Optimize extraction buffers to preserve redox states (e.g., include EDTA to chelate metal ions). Validate assays using knockout cell lines or competitive inhibitors to confirm signal specificity .
Advanced Research Questions
Q. How should experimental designs be structured to investigate this compound’s stability under varying redox conditions?
- Methodological Answer : Use a factorial design to test variables like pH (4–9), temperature (4–37°C), and reactive oxygen species (ROS) levels. Monitor degradation kinetics via UV-Vis spectroscopy and MS fragmentation patterns. Include negative controls (e.g., inert buffers) and triplicate runs to assess technical variability. Statistical tools like ANOVA can identify significant degradation pathways .
Q. What strategies resolve contradictions in reported bioactivity data for this compound across different cell lines?
- Methodological Answer : Conduct a systematic review to identify confounding variables (e.g., cell culture media composition, passage number). Replicate key studies under standardized conditions, and perform meta-analyses to quantify heterogeneity. Use pathway enrichment analysis (e.g., KEGG) to contextualize cell-specific signaling mechanisms .
Q. How can researchers optimize analytical protocols to distinguish this compound from its isomer Glutamyllysylcysteine?
- Methodological Answer : Leverage ion mobility spectrometry (IMS) paired with MS to separate isomers based on collisional cross-section differences. Optimize chromatographic gradients using hydrophilic interaction liquid chromatography (HILIC) to enhance retention time disparities. Validate with synthetic isomers and blinded sample testing .
Q. What methodologies are critical for studying this compound’s stability in physiological environments?
- Methodological Answer : Simulate physiological conditions (e.g., plasma, lysosomal pH) using biorelevant media. Employ accelerated stability testing (e.g., 40°C/75% RH) and real-time monitoring via LC-MS. Compare degradation products with in silico predictions (e.g., Schrödinger’s BioLuminate) to identify labile bonds .
Q. Which computational approaches best predict this compound’s interactions with target proteins like glutathione peroxidases?
- Methodological Answer : Perform molecular docking (AutoDock Vina) and molecular dynamics (MD) simulations (GROMACS) to model binding affinities and conformational changes. Validate predictions with mutagenesis studies (e.g., alanine scanning) and surface plasmon resonance (SPR) for kinetic binding analysis .
Q. How can researchers design studies to investigate synergistic effects between this compound and antioxidants like glutathione?
- Methodological Answer : Use combinatorial dose-response matrices (e.g., Chou-Talalay method) to calculate synergy scores (CI < 1). Measure ROS scavenging rates via fluorescent probes (e.g., DCFH-DA) and validate with transcriptomic profiling (RNA-seq) to identify co-regulated pathways .
Q. What steps ensure reproducibility in this compound research across independent laboratories?
- Methodological Answer : Adopt standardized protocols (e.g., MISEV guidelines for extracellular vesicle studies) and share raw data via repositories like Zenodo. Conduct interlaboratory trials with harmonized reagents and instrumentation. Publish detailed supplementary materials, including batch numbers and calibration curves .
類似化合物との比較
Comparison with Similar γ-Glutamyl Compounds
Structural and Functional Differences
The table below compares Glutamylcysteyllysine with four structurally related compounds:
Key Observations:
Hydrophobic residues (e.g., leucine in γ-Glu-Leu) favor GGT-mediated hydrolysis, while charged residues (e.g., lysine) may alter enzyme kinetics .
Enzyme Interactions: γ-Glutamylcysteine synthetase (GCS) catalyzes the formation of γ-Glu-Cys, a rate-limiting step in GSH synthesis. This compound is unlikely to be a GCS substrate due to its tripeptide structure, relying instead on GGT for processing .
Clinical Significance: Glutathione deficiency is well-documented, causing oxidative tissue damage and neurological impairments. γ-Glutamyltyrosine and γ-Glutamylleucine are primarily biomarkers for γ-glutamyl cycle activity rather than direct disease mediators .
Research Findings and Limitations
γ-Glutamyltransferase (GGT) Specificity: Studies on bovine lens GGT show broad substrate specificity, with optimal activity for cysteine-containing peptides (e.g., γ-Glu-Cys). Lysine-containing analogs like this compound may exhibit reduced catalytic efficiency due to steric or charge interference .
Synthetic and Analytical Challenges :
- This compound lacks commercial availability and standardized analytical data (e.g., CAS number, PubChem ID), hindering experimental validation of its roles. In contrast, γ-Glutamyltyrosine and GSH are well-characterized .
Therapeutic Potential: Glutathione analogs, such as γ-Glu-Cys, are investigated for treating oxidative disorders.
特性
IUPAC Name |
6-amino-2-[[2-[(2-amino-4-carboxybutanoyl)amino]-3-sulfanylpropanoyl]amino]hexanoic acid | |
---|---|---|
Source | PubChem | |
URL | https://pubchem.ncbi.nlm.nih.gov | |
Description | Data deposited in or computed by PubChem | |
InChI |
InChI=1S/C14H26N4O6S/c15-6-2-1-3-9(14(23)24)17-13(22)10(7-25)18-12(21)8(16)4-5-11(19)20/h8-10,25H,1-7,15-16H2,(H,17,22)(H,18,21)(H,19,20)(H,23,24) | |
Source | PubChem | |
URL | https://pubchem.ncbi.nlm.nih.gov | |
Description | Data deposited in or computed by PubChem | |
InChI Key |
ISXJHXGYMJKXOI-UHFFFAOYSA-N | |
Source | PubChem | |
URL | https://pubchem.ncbi.nlm.nih.gov | |
Description | Data deposited in or computed by PubChem | |
Canonical SMILES |
C(CCN)CC(C(=O)O)NC(=O)C(CS)NC(=O)C(CCC(=O)O)N | |
Source | PubChem | |
URL | https://pubchem.ncbi.nlm.nih.gov | |
Description | Data deposited in or computed by PubChem | |
Molecular Formula |
C14H26N4O6S | |
Source | PubChem | |
URL | https://pubchem.ncbi.nlm.nih.gov | |
Description | Data deposited in or computed by PubChem | |
Molecular Weight |
378.45 g/mol | |
Source | PubChem | |
URL | https://pubchem.ncbi.nlm.nih.gov | |
Description | Data deposited in or computed by PubChem | |
Retrosynthesis Analysis
AI-Powered Synthesis Planning: Our tool employs the Template_relevance Pistachio, Template_relevance Bkms_metabolic, Template_relevance Pistachio_ringbreaker, Template_relevance Reaxys, Template_relevance Reaxys_biocatalysis model, leveraging a vast database of chemical reactions to predict feasible synthetic routes.
One-Step Synthesis Focus: Specifically designed for one-step synthesis, it provides concise and direct routes for your target compounds, streamlining the synthesis process.
Accurate Predictions: Utilizing the extensive PISTACHIO, BKMS_METABOLIC, PISTACHIO_RINGBREAKER, REAXYS, REAXYS_BIOCATALYSIS database, our tool offers high-accuracy predictions, reflecting the latest in chemical research and data.
Strategy Settings
Precursor scoring | Relevance Heuristic |
---|---|
Min. plausibility | 0.01 |
Model | Template_relevance |
Template Set | Pistachio/Bkms_metabolic/Pistachio_ringbreaker/Reaxys/Reaxys_biocatalysis |
Top-N result to add to graph | 6 |
Feasible Synthetic Routes
試験管内研究製品の免責事項と情報
BenchChemで提示されるすべての記事および製品情報は、情報提供を目的としています。BenchChemで購入可能な製品は、生体外研究のために特別に設計されています。生体外研究は、ラテン語の "in glass" に由来し、生物体の外で行われる実験を指します。これらの製品は医薬品または薬として分類されておらず、FDAから任何の医療状態、病気、または疾患の予防、治療、または治癒のために承認されていません。これらの製品を人間または動物に体内に導入する形態は、法律により厳格に禁止されています。これらのガイドラインに従うことは、研究と実験において法的および倫理的な基準の遵守を確実にするために重要です。