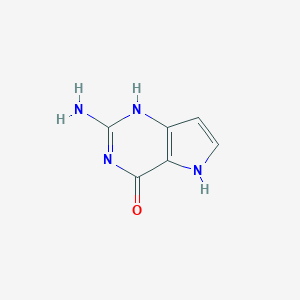
9-Deazaguanine
概要
説明
9-Deazaguanine is a small molecule and an analog of guanine . It exhibits potent inhibitory activity against purine nucleoside phosphorylase (PNP) . It reacts with the hydrogen bond of the enzyme and prevents its reaction with a substrate .
Synthesis Analysis
9-Deazaguanine and its related analogues were designed as multi-substrate analogue inhibitors against PNP . The synthesis involves using benzyloxymethyl group protection, DMF-dimethylacetal treatment, reductive cyclization, ethanolic ammonia treatment, and catalytic hydrogenation for removal of the protecting group .Molecular Structure Analysis
The molecular formula of 9-Deazaguanine is C6H6N4O . It binds to the target enzymes trifluoroacetic acid (TFA) and hydrogen bond interactions with water molecules .Chemical Reactions Analysis
9-Deazaguanine acts as a potent inhibitor of purine nucleoside phosphorylase (PNP) . It reacts with the hydrogen bond of the enzyme and prevents its reaction with a substrate .Physical And Chemical Properties Analysis
9-Deazaguanine has a density of 1.9±0.1 g/cm3, a molar refractivity of 37.0±0.5 cm3, and a molar volume of 80.2±7.0 cm3 . It has 5 H bond acceptors, 4 H bond donors, and 0 freely rotating bonds .科学的研究の応用
Inhibitor of Purine Nucleoside Phosphorylase (PNP)
9-Deazaguanine and its derivatives, such as 9-(5′,5′-Difluoro-5′-phosphonopentyl)-9-deazaguanine (DFPP-DG), have been designed as multi-substrate analogue inhibitors against purine nucleoside phosphorylase (PNP). This is based on the X-ray crystallographic data obtained for the binary complex of 9-(5′,5′-difluoro-5′-phosphonopentyl)guanine (DFPP-G) with calf-spleen PNP .
Synthesis of Antiviral Agents
Being an analogue of guanine, one of the fundamental bases in DNA and RNA, 9-Deazaguanine finds utility in the synthesis of antiviral agents .
Synthesis of Antineoplastic Agents
9-Deazaguanine is also used in the synthesis of antineoplastic agents, which are used in the treatment of cancer .
Therapeutic Agents
The compound is used in the synthesis of therapeutic agents, contributing to the development of new treatments for various diseases .
Nucleic Acid Studies
9-Deazaguanine offers valuable insights into the structure and function of nucleic acids, making it a valuable tool for scientific research .
Drug Development
In the realm of drug development, 9-Deazaguanine plays a significant role. It is employed to study nucleic acid structure and function, develop antiviral and antineoplastic agents, explore nucleic acid-protein interactions, and investigate the impact of mutations on nucleic acid structure and function .
作用機序
Target of Action
The primary target of 9-Deazaguanine is Hypoxanthine-guanine phosphoribosyltransferase (HGPRT) . This enzyme plays a crucial role in the purine salvage pathway, which is essential for nucleotide synthesis.
Mode of Action
9-Deazaguanine is a nucleoside analog and acts as a potent inhibitor of purine nucleoside phosphorylase (PNP) . It is believed to act as a competitive inhibitor of guanine in the formation of nucleic acid structures . Additionally, it is thought to interfere with guanine binding to proteins and other molecules .
Biochemical Pathways
9-Deazaguanine affects the purine salvage pathway by inhibiting the enzyme HGPRT . This disruption can lead to changes in nucleic acid structure formation and disrupt guanine-protein binding . Furthermore, it may impede DNA and RNA replication .
Result of Action
The inhibition of HGPRT by 9-Deazaguanine can lead to a disruption in nucleic acid structure formation and guanine-protein binding . This may result in the impediment of DNA and RNA replication .
Action Environment
Safety and Hazards
将来の方向性
特性
IUPAC Name |
2-amino-3,5-dihydropyrrolo[3,2-d]pyrimidin-4-one | |
---|---|---|
Source | PubChem | |
URL | https://pubchem.ncbi.nlm.nih.gov | |
Description | Data deposited in or computed by PubChem | |
InChI |
InChI=1S/C6H6N4O/c7-6-9-3-1-2-8-4(3)5(11)10-6/h1-2,8H,(H3,7,9,10,11) | |
Source | PubChem | |
URL | https://pubchem.ncbi.nlm.nih.gov | |
Description | Data deposited in or computed by PubChem | |
InChI Key |
FFYPRJYSJODFFD-UHFFFAOYSA-N | |
Source | PubChem | |
URL | https://pubchem.ncbi.nlm.nih.gov | |
Description | Data deposited in or computed by PubChem | |
Canonical SMILES |
C1=CNC2=C1N=C(NC2=O)N | |
Source | PubChem | |
URL | https://pubchem.ncbi.nlm.nih.gov | |
Description | Data deposited in or computed by PubChem | |
Molecular Formula |
C6H6N4O | |
Source | PubChem | |
URL | https://pubchem.ncbi.nlm.nih.gov | |
Description | Data deposited in or computed by PubChem | |
DSSTOX Substance ID |
DTXSID20216202 | |
Record name | 9-Deazaguanine | |
Source | EPA DSSTox | |
URL | https://comptox.epa.gov/dashboard/DTXSID20216202 | |
Description | DSSTox provides a high quality public chemistry resource for supporting improved predictive toxicology. | |
Molecular Weight |
150.14 g/mol | |
Source | PubChem | |
URL | https://pubchem.ncbi.nlm.nih.gov | |
Description | Data deposited in or computed by PubChem | |
Product Name |
9-Deazaguanine | |
CAS RN |
65996-58-9 | |
Record name | 9-Deazaguanine | |
Source | ChemIDplus | |
URL | https://pubchem.ncbi.nlm.nih.gov/substance/?source=chemidplus&sourceid=0065996589 | |
Description | ChemIDplus is a free, web search system that provides access to the structure and nomenclature authority files used for the identification of chemical substances cited in National Library of Medicine (NLM) databases, including the TOXNET system. | |
Record name | 9-Deazaguanine | |
Source | DrugBank | |
URL | https://www.drugbank.ca/drugs/DB04356 | |
Description | The DrugBank database is a unique bioinformatics and cheminformatics resource that combines detailed drug (i.e. chemical, pharmacological and pharmaceutical) data with comprehensive drug target (i.e. sequence, structure, and pathway) information. | |
Explanation | Creative Common's Attribution-NonCommercial 4.0 International License (http://creativecommons.org/licenses/by-nc/4.0/legalcode) | |
Record name | 65996-58-9 | |
Source | DTP/NCI | |
URL | https://dtp.cancer.gov/dtpstandard/servlet/dwindex?searchtype=NSC&outputformat=html&searchlist=344522 | |
Description | The NCI Development Therapeutics Program (DTP) provides services and resources to the academic and private-sector research communities worldwide to facilitate the discovery and development of new cancer therapeutic agents. | |
Explanation | Unless otherwise indicated, all text within NCI products is free of copyright and may be reused without our permission. Credit the National Cancer Institute as the source. | |
Record name | 9-Deazaguanine | |
Source | EPA DSSTox | |
URL | https://comptox.epa.gov/dashboard/DTXSID20216202 | |
Description | DSSTox provides a high quality public chemistry resource for supporting improved predictive toxicology. | |
Record name | 65996-58-9 | |
Source | European Chemicals Agency (ECHA) | |
URL | https://echa.europa.eu/information-on-chemicals | |
Description | The European Chemicals Agency (ECHA) is an agency of the European Union which is the driving force among regulatory authorities in implementing the EU's groundbreaking chemicals legislation for the benefit of human health and the environment as well as for innovation and competitiveness. | |
Explanation | Use of the information, documents and data from the ECHA website is subject to the terms and conditions of this Legal Notice, and subject to other binding limitations provided for under applicable law, the information, documents and data made available on the ECHA website may be reproduced, distributed and/or used, totally or in part, for non-commercial purposes provided that ECHA is acknowledged as the source: "Source: European Chemicals Agency, http://echa.europa.eu/". Such acknowledgement must be included in each copy of the material. ECHA permits and encourages organisations and individuals to create links to the ECHA website under the following cumulative conditions: Links can only be made to webpages that provide a link to the Legal Notice page. | |
Record name | 9-DEAZAGUANINE | |
Source | FDA Global Substance Registration System (GSRS) | |
URL | https://gsrs.ncats.nih.gov/ginas/app/beta/substances/H2O1DD3CAN | |
Description | The FDA Global Substance Registration System (GSRS) enables the efficient and accurate exchange of information on what substances are in regulated products. Instead of relying on names, which vary across regulatory domains, countries, and regions, the GSRS knowledge base makes it possible for substances to be defined by standardized, scientific descriptions. | |
Explanation | Unless otherwise noted, the contents of the FDA website (www.fda.gov), both text and graphics, are not copyrighted. They are in the public domain and may be republished, reprinted and otherwise used freely by anyone without the need to obtain permission from FDA. Credit to the U.S. Food and Drug Administration as the source is appreciated but not required. | |
Retrosynthesis Analysis
AI-Powered Synthesis Planning: Our tool employs the Template_relevance Pistachio, Template_relevance Bkms_metabolic, Template_relevance Pistachio_ringbreaker, Template_relevance Reaxys, Template_relevance Reaxys_biocatalysis model, leveraging a vast database of chemical reactions to predict feasible synthetic routes.
One-Step Synthesis Focus: Specifically designed for one-step synthesis, it provides concise and direct routes for your target compounds, streamlining the synthesis process.
Accurate Predictions: Utilizing the extensive PISTACHIO, BKMS_METABOLIC, PISTACHIO_RINGBREAKER, REAXYS, REAXYS_BIOCATALYSIS database, our tool offers high-accuracy predictions, reflecting the latest in chemical research and data.
Strategy Settings
Precursor scoring | Relevance Heuristic |
---|---|
Min. plausibility | 0.01 |
Model | Template_relevance |
Template Set | Pistachio/Bkms_metabolic/Pistachio_ringbreaker/Reaxys/Reaxys_biocatalysis |
Top-N result to add to graph | 6 |
Feasible Synthetic Routes
Q & A
Q1: What is the primary mechanism of action of 9-Deazaguanine?
A1: 9-Deazaguanine acts as a potent inhibitor of the enzyme purine nucleoside phosphorylase (PNP). [, , , , , ] PNP plays a crucial role in the purine salvage pathway, responsible for the reversible phosphorolytic cleavage of purine ribonucleosides and 2'-deoxyribonucleosides. [] By inhibiting PNP, 9-DG disrupts purine metabolism, ultimately impacting DNA synthesis and cellular processes, particularly in rapidly dividing cells like T-cells and cancer cells. [, , ]
Q2: How does 9-Deazaguanine's inhibition of PNP affect T-cell function?
A2: Inhibition of PNP by 9-DG leads to the accumulation of deoxyguanosine and a decrease in deoxyguanosine triphosphate (dGTP) levels, particularly in T-cells. [] This depletion of dGTP selectively inhibits T-cell proliferation and function, making 9-DG a potential immunosuppressive agent. [, , ]
Q3: What downstream effects on gene expression have been observed in leukemia cells treated with 9-Deazaguanine and its derivatives?
A3: Studies on leukemia cells treated with 9-Deazaguanine derivatives observed altered expression of genes involved in apoptosis and purine metabolism. Notably, a significant decrease in the expression of the PNP gene was observed. [] Additionally, downregulation of pro-apoptotic genes, caspase-3 and p53, was observed in specific leukemia cell lines (HL-60 and MOLT-4) after treatment. []
Q4: What is the molecular formula and weight of 9-Deazaguanine?
A4: The molecular formula of 9-Deazaguanine is C8H7N5O, and its molecular weight is 193.18 g/mol.
Q5: Are there any specific spectroscopic data available for 9-Deazaguanine?
A5: Yes, resonance Raman spectroscopy has been utilized to elucidate the solution structure, protonation states, and predominant tautomers of 9-Deazaguanine and its hypoxanthine analogue, 9-deazahypoxanthine (9DAH), at different pH levels. [] These findings provide valuable insights into the molecular behavior of these compounds under physiological conditions.
Q6: How do structural modifications of the 9-Deazaguanine scaffold affect its inhibitory activity against PNP?
A6: Numerous studies have investigated the structure-activity relationships of 9-Deazaguanine derivatives.
Q7: What is the significance of the flexible loop in the PNP enzyme for 9-Deazaguanine binding?
A7: The flexible loop in the PNP enzyme plays a crucial role in substrate binding and catalysis. [] Studies utilizing site-directed mutagenesis, stop-flow kinetics, chemical modification, and NMR experiments revealed that the conserved Ser73 and Tyr74 residues within this loop are essential for the binding of 9-Deazaguanine and other substrates. [] The loop undergoes conformational changes upon substrate binding, suggesting its involvement in optimizing the active site for catalysis. []
Q8: Have computational methods been employed in the development of 9-Deazaguanine-based PNP inhibitors?
A8: Yes, computer-aided molecular modeling (CAMM) has been instrumental in the design of potent 9-Deazaguanine-based PNP inhibitors. [, ] By leveraging X-ray crystallographic data of PNP-inhibitor complexes, researchers can visualize interactions within the active site. This information guides the design of novel analogues with improved binding affinity and inhibitory potency. [, ]
Q9: Are there QSAR models available for predicting the activity of 9-Deazaguanine derivatives?
A9: Yes, both descriptor- and fragment-based quantitative structure-activity relationship (QSAR) models have been developed for a series of 9-Deazaguanine analogues as inhibitors of Schistosoma mansoni PNP (SmPNP). [] These models exhibit significant statistical parameters, indicating their potential for predicting the inhibitory potency of novel, untested compounds, thereby aiding in the drug discovery process. []
Q10: What is the oral bioavailability of 9-benzyl-9-deazaguanine (BzDAG) in rats?
A11: Studies in rats have shown that BzDAG exhibits an oral bioavailability of 48% at a dose of 5 mg/kg. [] This indicates that BzDAG is absorbed relatively well following oral administration.
Q11: How does 9-benzyl-9-deazaguanine (BzDAG) affect plasma levels of purine nucleosides?
A12: BzDAG administration in rats led to increased plasma levels of endogenous inosine. [] Additionally, when co-administered with 2',3'-dideoxyinosine, BzDAG increased the plasma levels of 2',3'-dideoxyinosine, suggesting an interaction with its metabolism. [] These findings indicate that BzDAG can significantly alter the pharmacokinetics of purine nucleosides in vivo.
Q12: Has the antitumor activity of 9-Deazaguanine derivatives been evaluated in vitro?
A13: Yes, several studies have investigated the antiproliferative effects of 9-Deazaguanine derivatives on various cancer cell lines, including leukemia and solid tumor cells. [, ] Moderate to potent growth inhibitory effects have been observed, with some derivatives demonstrating greater potency against specific cell lines. [, ]
Q13: What are the in vivo effects of 9-Deazaguanine-based PNP inhibitors on immune responses?
A14: Studies employing 9-benzyl-9-deazaguanine (BzDAG) have demonstrated its ability to suppress the human mixed lymphocyte reaction (MLR) in vitro. [] This finding supports the potential of PNP inhibitors like BzDAG as immunosuppressive agents for T-cell-mediated immune responses.
試験管内研究製品の免責事項と情報
BenchChemで提示されるすべての記事および製品情報は、情報提供を目的としています。BenchChemで購入可能な製品は、生体外研究のために特別に設計されています。生体外研究は、ラテン語の "in glass" に由来し、生物体の外で行われる実験を指します。これらの製品は医薬品または薬として分類されておらず、FDAから任何の医療状態、病気、または疾患の予防、治療、または治癒のために承認されていません。これらの製品を人間または動物に体内に導入する形態は、法律により厳格に禁止されています。これらのガイドラインに従うことは、研究と実験において法的および倫理的な基準の遵守を確実にするために重要です。