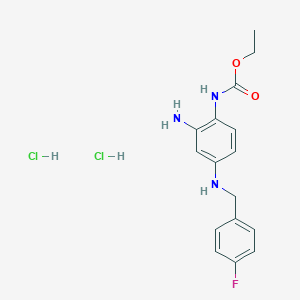
Retigabine Dihydrochloride
概要
説明
準備方法
レチガビン二塩酸塩は、複数段階の化学プロセスによって合成されます。合成には、遊離塩基の調製が含まれ、次にそれが二塩酸塩に変換されます。 合成経路には、一般的に以下の手順が含まれます :
中間体の形成: 合成は、2-アミノ-4-(4-フルオロベンジルアミノ)フェノールとクロロギ酸エチルを反応させて、中間体エチルN-(2-アミノ-4-(4-フルオロベンジルアミノ)フェニル)カルバメートを形成することから始まります。
遊離塩基への変換: 次に、中間体を塩基で処理して、レチガビンの遊離塩基を得ます。
二塩酸塩の形成: 最後に、遊離塩基を塩酸と反応させて、レチガビン二塩酸塩を形成します。
化学反応の分析
レチガビン二塩酸塩は、次のような様々な化学反応を受けます。
これらの反応に使用される一般的な試薬と条件には、脱プロトン化のための塩基と塩形成のための酸が含まれます。 これらの反応で生成される主な生成物は、レチガビンの遊離塩基と二塩酸塩です .
科学研究への応用
レチガビン二塩酸塩は、次のような幅広い科学研究への応用があります。
科学的研究の応用
Clinical Applications
-
Epilepsy Treatment
- Retigabine has been approved for use as an adjunctive therapy in adults with partial-onset seizures. Clinical trials have demonstrated its efficacy in reducing seizure frequency and severity across various epilepsy models, including those resistant to other antiepileptic drugs (AEDs) .
- Its unique mechanism allows it to be effective against generalized tonic-clonic seizures and absence seizures, making it versatile in treating different forms of epilepsy .
-
Psychiatric Disorders
- Recent studies indicate potential antipsychotic effects of retigabine through modulation of dopaminergic pathways. It has shown promise in reducing drug-seeking behavior in models of addiction by affecting mesolimbic circuitry . This suggests its potential utility in treating conditions like schizophrenia or substance use disorders.
- Neuropathic Pain
Research Applications
Retigabine is widely used in preclinical research to study neuronal excitability and the pathophysiology of epilepsy:
- In Vitro Studies
- In Vivo Models
- Animal models have been critical for understanding the pharmacodynamics of retigabine. For instance, studies on mice have shown that doses as low as 0.01 mg/kg can effectively reduce seizure activity . Additionally, retigabine's ability to synergize with other AEDs in these models highlights its potential for combination therapies .
Case Study 1: Efficacy in Drug-Resistant Epilepsy
A study involving patients with drug-resistant focal epilepsy demonstrated that adding retigabine to their treatment regimen resulted in a statistically significant reduction in seizure frequency compared to baseline measurements. The patients reported improved quality of life metrics alongside the reduction in seizure episodes.
Case Study 2: Behavioral Effects on Addiction Models
In a rodent model assessing cocaine addiction, retigabine administration significantly decreased the frequency and intensity of drug-seeking behavior. This effect was attributed to its action on the KCNQ channels within the mesolimbic pathway, suggesting a novel approach for addiction treatment .
Data Summary
作用機序
レチガビン二塩酸塩は、電位依存性カリウムチャネル、特にKv7.2-Kv7.5を選択的に活性化することで作用します . これらのチャネルは、M電流を生成します。M電流は、膜電位を安定させ、神経興奮性を制御する、閾値以下のカリウム電流です . レチガビンは、これらのチャネルを開くことによって、神経興奮性を低下させ、発作の発生を防ぎます .
類似化合物の比較
レチガビン二塩酸塩は、カリウムチャネル開口薬としての作用機序により、抗てんかん薬の中ではユニークな存在です . 類似の化合物には、次のようなものがあります。
フルピルチン: レチガビンの構造的に近い類似体であり、主に鎮痛作用のために使用されます.
リノピルジン: レチガビンと構造的に類似しているKCNQブロッカー.
XE-991: 類似の化学構造を持つ別のKCNQブロッカー.
これらの化合物と比較して、レチガビンのカリウムチャネルを活性化する能力と、てんかんと神経障害性疼痛の動物モデルにおける幅広いスペクトルを持つ活性は、レチガビンをユニークで価値のある治療薬にしています .
類似化合物との比較
Retigabine dihydrochloride is unique among antiepileptic drugs due to its mechanism of action as a potassium channel opener . Similar compounds include:
Flupirtine: A close structural analogue of retigabine, used mainly for its analgesic properties.
Linopirdine: A KCNQ blocker that shares structural similarities with retigabine.
XE-991: Another KCNQ blocker with a similar chemical structure.
Compared to these compounds, retigabine’s ability to activate potassium channels and its broad-spectrum activity in animal models of epilepsy and neuropathic pain make it a unique and valuable therapeutic agent .
生物活性
Retigabine dihydrochloride, also known as ezogabine, is an antiepileptic drug primarily recognized for its ability to modulate voltage-gated potassium channels, specifically the Kv7.2–7.5 subfamily. This compound has garnered attention due to its unique mechanism of action, which differentiates it from traditional antiepileptic medications. The following sections provide a comprehensive overview of the biological activity of retigabine, including its pharmacodynamics, efficacy in clinical settings, and emerging research findings.
Retigabine enhances potassium (K+) currents through Kv7 channels, leading to hyperpolarization of neuronal membranes and a reduction in excitability. This modulation is crucial in controlling seizure activity and has implications for treating various neurological conditions.
- Kv7 Channel Activation : Retigabine acts as a positive allosteric modulator of Kv7.2 and Kv7.3 channels, facilitating K+ conductance and shifting the voltage-dependent activation to more hyperpolarized potentials .
- Additional Interactions : It also exhibits weak inhibitory effects on sodium and calcium channels and interacts with GABA receptors, contributing to its antiepileptic properties .
Pharmacokinetics
Retigabine is characterized by the following pharmacokinetic properties:
- Bioavailability : Approximately 50–60% when administered orally.
- Metabolism : Primarily metabolized through N-glucuronidation and N-acetylation, resulting in inactive metabolites.
- Protein Binding : About 80% bound to plasma proteins.
- Half-life : Ranges from 8 to 11 hours .
Clinical Efficacy
Retigabine has been evaluated in various clinical trials, particularly for its efficacy as an adjunctive therapy for partial-onset seizures.
- Seizure Frequency Reduction : Placebo-controlled trials reported a reduction in seizure frequency by 23.4% to 44.3%, with responder rates varying from 23.2% to 47% depending on dosage .
- Adverse Effects : Common side effects include dizziness, fatigue, confusion, and urinary tract infections, with blue-gray skin discoloration noted as a rare but significant adverse effect .
Research Findings
Recent studies have expanded the understanding of retigabine's biological activity beyond epilepsy treatment.
Case Studies
- Cocaine Self-Administration : A study demonstrated that retigabine reduces the motivation for cocaine self-administration in rats, suggesting potential applications in treating substance use disorders. The compound decreased dopamine release from mesencephalic dopaminergic neurons and reduced locomotor activity induced by psychostimulants .
- Cardiovascular Effects : Research indicated that retigabine influences cardiovascular responses by modulating vascular regulation through Kv7 channels. In conscious rat models, retigabine administration affected heart rate and blood pressure responses under hypertensive conditions .
Data Summary
The following table summarizes key findings related to retigabine's biological activity:
Parameter | Details |
---|---|
Mechanism of Action | Kv7 channel activation; enhances K+ conductance |
Bioavailability | 50–60% |
Half-life | 8–11 hours |
Common Side Effects | Dizziness, confusion, urinary tract infections |
Efficacy in Seizures | 23.4% - 44.3% reduction in seizure frequency |
Research Applications | Reduces cocaine self-administration; affects cardiovascular responses |
Q & A
Basic Research Questions
Q. What experimental models are most suitable for evaluating Retigabine Dihydrochloride's efficacy in modulating potassium channels?
Answer: this compound’s primary mechanism involves activating neuronal Kv7.2-7.5 (KCNQ2-5) potassium channels, which can be studied using:
- In vitro electrophysiology : Utilize whole-cell patch-clamp recordings to measure hyperpolarization effects on membrane potential and action potential duration. Standard protocols include using intracellular solutions with 140 mM KCl and extracellular solutions with 5 mM KCl, as described in electrophysiological studies .
- Ex vivo muscle preparations : For muscle hyperexcitability studies, isolated muscle fibers from murine models (e.g., Clcn1 mutant mice) can assess retigabine’s ability to shorten myotonic discharges, as demonstrated in studies measuring force transients .
Q. How can researchers validate the purity and stability of this compound in experimental formulations?
Answer:
- Chromatographic methods : Use HPLC or GC-FID with optimized mobile phases (e.g., acetonitrile-phosphate buffer) to quantify retigabine and detect degradation products. For example, a GC-FID method validated for related dihydrochloride compounds (e.g., Tilorone Dihydrochloride) employs a DB-5 column and splitless injection mode .
- Spectroscopic characterization : NMR (¹H/¹³C) and high-resolution mass spectrometry (HRMS) confirm structural integrity. For novel batches, compare with reference spectra from authoritative databases (e.g., PubChem or peer-reviewed syntheses) .
Q. What are the key considerations for designing dose-response studies with this compound in vivo?
Answer:
- Species-specific pharmacokinetics : Mice typically require doses of 5–15 mg/kg intraperitoneally to achieve CNS penetration, as shown in myotonia congenita models .
- Temporal effects : Monitor time-to-peak plasma concentration (Tmax ~30–60 minutes post-injection) and duration of K<sup>+</sup> current activation (up to 4 hours).
- Control for off-target effects : Include vehicle controls and co-administer selective KCNQ blockers (e.g., XE991) to isolate retigabine-specific effects .
Advanced Research Questions
Q. How can contradictory findings between in vitro and in vivo efficacy of this compound be resolved?
Answer: Contradictions often arise from differences in tissue penetration, metabolite activity, or compensatory mechanisms. Strategies include:
- Pharmacokinetic-pharmacodynamic (PK-PD) modeling : Correlate plasma/tissue concentrations with electrophysiological outcomes. For example, in vivo retigabine may require higher doses due to blood-brain barrier efflux transporters .
- Tissue-specific profiling : Use microdialysis or MALDI imaging to map retigabine distribution in target tissues (e.g., skeletal muscle vs. brain) .
- Genetic knockdown models : Silence alternative K<sup>+</sup> channels (e.g., Kv1.1) in knockout mice to isolate KCNQ-dependent effects .
Q. What methodological approaches address this compound’s limited CNS efficacy despite robust muscle effects?
Answer:
- CNS-targeted delivery : Formulate retigabine in nanoparticles or liposomes to enhance brain bioavailability. For example, PEGylated liposomes can bypass P-glycoprotein efflux .
- Combination therapies : Co-administer retigabine with Na<sup>+</sup> channel blockers (e.g., mexiletine) to synergistically reduce hyperexcitability in both muscle and neurons .
- Behavioral assays : Use rotarod or grip-strength tests in murine models to differentiate peripheral vs. central side effects .
Q. How should researchers optimize analytical protocols for detecting this compound degradation products in environmental samples?
Answer:
- Accelerated degradation studies : Expose retigabine to stress conditions (e.g., UV light, acidic/basic hydrolysis) and identify byproducts via LC-MS/MS. For soil samples, employ solid-phase extraction (SPE) with C18 cartridges before analysis .
- Stability-indicating assays : Validate methods using ICH guidelines (e.g., Q2(R1)) to ensure specificity for retigabine amid degradation peaks .
Q. Data Contradiction Analysis
Q. What statistical frameworks are recommended for reconciling variability in this compound’s electrophysiological data?
Answer:
- Mixed-effects models : Account for repeated measurements (e.g., pre/post-retigabine recordings in the same cell) and inter-subject variability. Use software like R (lme4 package) or Prism .
- Non-parametric tests : Apply Wilcoxon signed-rank tests for non-normal distributions (common in small-sample electrophysiology datasets) .
Q. How can conflicting reports on this compound’s pro-apoptotic vs. neuroprotective effects be investigated?
Answer:
- Dose-response meta-analysis : Pool data from multiple studies to identify threshold concentrations where neuroprotection transitions to toxicity.
- Cell-type-specific assays : Use primary neuron cultures vs. immortalized cell lines (e.g., SH-SY5Y) to isolate context-dependent effects .
Q. Tables for Methodological Reference
Table 1: Comparison of Analytical Techniques for this compound
Method | Sensitivity (LOD) | Key Applications | Limitations | Reference |
---|---|---|---|---|
HPLC-UV | 0.1 µg/mL | Purity analysis, stability testing | Low resolution for isomers | |
GC-FID | 0.05 µg/mL | Soil/environmental detection | Requires derivatization | |
LC-MS/MS | 0.01 ng/mL | Metabolite profiling | High equipment cost |
Table 2: In Vitro vs. In Vivo Outcomes of this compound
Parameter | In Vitro (Patch-Clamp) | In Vivo (Murine Model) | Contradiction Resolution Strategy |
---|---|---|---|
KCNQ activation | EC50 ~1–5 µM | EC50 ~10–20 µM | Adjust for protein binding |
Duration of effect | 10–30 minutes | 2–4 hours | Metabolite activity assays |
CNS penetration | N/A | Limited (brain/plasma ratio 0.2) | Nanoparticle formulation |
特性
IUPAC Name |
ethyl N-[2-amino-4-[(4-fluorophenyl)methylamino]phenyl]carbamate;dihydrochloride | |
---|---|---|
Source | PubChem | |
URL | https://pubchem.ncbi.nlm.nih.gov | |
Description | Data deposited in or computed by PubChem | |
InChI |
InChI=1S/C16H18FN3O2.2ClH/c1-2-22-16(21)20-15-8-7-13(9-14(15)18)19-10-11-3-5-12(17)6-4-11;;/h3-9,19H,2,10,18H2,1H3,(H,20,21);2*1H | |
Source | PubChem | |
URL | https://pubchem.ncbi.nlm.nih.gov | |
Description | Data deposited in or computed by PubChem | |
InChI Key |
WSGFOWNASITQHJ-UHFFFAOYSA-N | |
Source | PubChem | |
URL | https://pubchem.ncbi.nlm.nih.gov | |
Description | Data deposited in or computed by PubChem | |
Canonical SMILES |
CCOC(=O)NC1=C(C=C(C=C1)NCC2=CC=C(C=C2)F)N.Cl.Cl | |
Source | PubChem | |
URL | https://pubchem.ncbi.nlm.nih.gov | |
Description | Data deposited in or computed by PubChem | |
Molecular Formula |
C16H20Cl2FN3O2 | |
Source | PubChem | |
URL | https://pubchem.ncbi.nlm.nih.gov | |
Description | Data deposited in or computed by PubChem | |
DSSTOX Substance ID |
DTXSID00164616 | |
Record name | Ezogabine dihydrochloride | |
Source | EPA DSSTox | |
URL | https://comptox.epa.gov/dashboard/DTXSID00164616 | |
Description | DSSTox provides a high quality public chemistry resource for supporting improved predictive toxicology. | |
Molecular Weight |
376.2 g/mol | |
Source | PubChem | |
URL | https://pubchem.ncbi.nlm.nih.gov | |
Description | Data deposited in or computed by PubChem | |
CAS No. |
150812-13-8 | |
Record name | Ezogabine dihydrochloride | |
Source | ChemIDplus | |
URL | https://pubchem.ncbi.nlm.nih.gov/substance/?source=chemidplus&sourceid=0150812138 | |
Description | ChemIDplus is a free, web search system that provides access to the structure and nomenclature authority files used for the identification of chemical substances cited in National Library of Medicine (NLM) databases, including the TOXNET system. | |
Record name | Ezogabine dihydrochloride | |
Source | EPA DSSTox | |
URL | https://comptox.epa.gov/dashboard/DTXSID00164616 | |
Description | DSSTox provides a high quality public chemistry resource for supporting improved predictive toxicology. | |
Record name | EZOGABINE DIHYDROCHLORIDE | |
Source | FDA Global Substance Registration System (GSRS) | |
URL | https://gsrs.ncats.nih.gov/ginas/app/beta/substances/WV9W9019AP | |
Description | The FDA Global Substance Registration System (GSRS) enables the efficient and accurate exchange of information on what substances are in regulated products. Instead of relying on names, which vary across regulatory domains, countries, and regions, the GSRS knowledge base makes it possible for substances to be defined by standardized, scientific descriptions. | |
Explanation | Unless otherwise noted, the contents of the FDA website (www.fda.gov), both text and graphics, are not copyrighted. They are in the public domain and may be republished, reprinted and otherwise used freely by anyone without the need to obtain permission from FDA. Credit to the U.S. Food and Drug Administration as the source is appreciated but not required. | |
Retrosynthesis Analysis
AI-Powered Synthesis Planning: Our tool employs the Template_relevance Pistachio, Template_relevance Bkms_metabolic, Template_relevance Pistachio_ringbreaker, Template_relevance Reaxys, Template_relevance Reaxys_biocatalysis model, leveraging a vast database of chemical reactions to predict feasible synthetic routes.
One-Step Synthesis Focus: Specifically designed for one-step synthesis, it provides concise and direct routes for your target compounds, streamlining the synthesis process.
Accurate Predictions: Utilizing the extensive PISTACHIO, BKMS_METABOLIC, PISTACHIO_RINGBREAKER, REAXYS, REAXYS_BIOCATALYSIS database, our tool offers high-accuracy predictions, reflecting the latest in chemical research and data.
Strategy Settings
Precursor scoring | Relevance Heuristic |
---|---|
Min. plausibility | 0.01 |
Model | Template_relevance |
Template Set | Pistachio/Bkms_metabolic/Pistachio_ringbreaker/Reaxys/Reaxys_biocatalysis |
Top-N result to add to graph | 6 |
Feasible Synthetic Routes
試験管内研究製品の免責事項と情報
BenchChemで提示されるすべての記事および製品情報は、情報提供を目的としています。BenchChemで購入可能な製品は、生体外研究のために特別に設計されています。生体外研究は、ラテン語の "in glass" に由来し、生物体の外で行われる実験を指します。これらの製品は医薬品または薬として分類されておらず、FDAから任何の医療状態、病気、または疾患の予防、治療、または治癒のために承認されていません。これらの製品を人間または動物に体内に導入する形態は、法律により厳格に禁止されています。これらのガイドラインに従うことは、研究と実験において法的および倫理的な基準の遵守を確実にするために重要です。