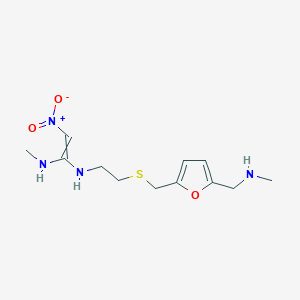
Desmethyl Ranitidine
概要
説明
Desmethyl Ranitidine is a derivative of Ranitidine, a histamine H2-receptor antagonist . It is used to prevent and treat gastric-acid associated conditions, including ulcers, due to its ability to decrease gastric acid secretion . The empirical formula of Desmethyl Ranitidine is C12H20N4O3S .
Synthesis Analysis
The synthesis of Desmethyl Ranitidine involves complex chemical reactions. A study on the LC-MS/MS quantification of NMDA in the drug product, ranitidine, was developed to analyze the synthesis process . The rate of reaction is influenced by heat, humidity, and the crystal morphology .
Molecular Structure Analysis
The molecular formula of Desmethyl Ranitidine is C12H20N4O3S . It contains a total of 40 bonds, including 20 non-H bonds, 8 multiple bonds, 10 rotatable bonds, 3 double bonds, 5 aromatic bonds, 1 five-membered ring, 3 secondary amines (aliphatic), 1 nitro group (aliphatic), 1 sulfide, and 1 Furane .
Chemical Reactions Analysis
Ranitidine undergoes self-decomposition, causing nitrite concentration to increase during storage. The rate of reaction is influenced by heat, humidity, and the crystal morphology . DMA released from ranitidine could also lead to NDMA formation upon contact with nitrosating agents . The destruction of nitrosamines requires strong reaction conditions .
Physical And Chemical Properties Analysis
Desmethyl Ranitidine is a granular substance that is soluble in water . It has a slightly bitter taste and sulfur-like odor . The volume of distribution is about 1.4 L/kg and serum protein binding averages 15% .
科学的研究の応用
Quantification of N-Nitrosamine, NDMA
Desmethyl Ranitidine is used in the development of a sensitive and robust method for the LC-MS/MS quantification of NMDA containing the drug product, ranitidine . This application highlights accurate NDMA quantification, achieving LOQs of 0.75 ppb (11 picograms on-column), using the Waters ACQUITY UPLC H-Class PLUS System and XSelect HSS T3 Column for chromatographic separation .
Risk Assessment of Nitroso Impurities
Desmethyl Ranitidine is used in the risk assessment of nitrosamines or their precursors that may be present in any component of the finished dosage form . As a risk mitigation strategy, components with a high potential to form nitrosamine should be avoided .
Control Strategy for Nitroso Impurities
Desmethyl Ranitidine is used in the development of control strategies to maintain nitrosamines below acceptable intake levels . The formulation can be supplemented with nitrosating inhibitors, such as vitamin C, to stop the generation of nitrosamine .
Study of Self-Decomposition
Desmethyl Ranitidine undergoes self-decomposition, causing nitrite concentration to increase during storage . The rate of reaction is influenced by heat, humidity, and the crystal morphology .
Quantification of Nitrosamine Impurities
Desmethyl Ranitidine is used in the development of a highly sensitive and robust LC-MS/MS quantification method for six nitrosamine impurities (NDMA, NDEA, NEIPA, NDIPA, NDBA, and NMBA) in solutions containing irbesartan, losartan, valsartan (sartans), and ranitidine drug substances .
作用機序
Target of Action
Desmethyl Ranitidine, a metabolite of Ranitidine , primarily targets the histamine H2 receptors found in gastric parietal cells . These receptors play a crucial role in the secretion of gastric acid, which is essential for digestion .
Mode of Action
Desmethyl Ranitidine acts as a competitive inhibitor of histamine at the H2 receptors . By binding to these receptors, it prevents histamine from activating them, thereby inhibiting the secretion of gastric acid . This results in a decrease in gastric acid secretion, gastric volume, and hydrogen ion concentration .
Biochemical Pathways
The primary biochemical pathway affected by Desmethyl Ranitidine is the gastric acid secretion pathway . By inhibiting the H2 receptors, Desmethyl Ranitidine reduces the production of gastric acid, thereby affecting the overall process of digestion .
Pharmacokinetics
It is known that ranitidine, the parent compound, is rapidly absorbed following oral administration . Other metabolites of Ranitidine include S-oxide and desmethyl Ranitidine, with the former being the major metabolite
Result of Action
The primary molecular effect of Desmethyl Ranitidine’s action is the reduction of gastric acid secretion . This can lead to relief from conditions associated with excess gastric acid, such as ulcers and gastroesophageal reflux disease (GERD) . On a cellular level, Desmethyl Ranitidine’s action results in a decrease in the activity of parietal cells, the cells responsible for gastric acid secretion .
Action Environment
The action, efficacy, and stability of Desmethyl Ranitidine can be influenced by various environmental factors. For instance, the presence of nitrosamines or their precursors in any component of the finished dosage form can potentially affect the action of Desmethyl Ranitidine . Furthermore, conditions such as temperature and humidity can influence the stability of Desmethyl Ranitidine, as evidenced by the increase in NDMA levels in Ranitidine powder and tablets subjected to accelerated conditions .
Safety and Hazards
Avoid dust formation, breathing mist, gas or vapors, and contacting with skin and eye. Use personal protective equipment, wear chemical impermeable gloves, ensure adequate ventilation, remove all sources of ignition, evacuate personnel to safe areas, and keep people away from and upwind of spill/leak .
将来の方向性
The FDA has requested manufacturers to withdraw all prescription and over-the-counter ranitidine drugs from the market immediately due to the presence of a contaminant known as N-Nitrosodimethylamine (NDMA) in ranitidine medications . Future research and development efforts may focus on finding alternatives to ranitidine-containing medicines .
特性
IUPAC Name |
(E)-1-N-methyl-1-N'-[2-[[5-(methylaminomethyl)furan-2-yl]methylsulfanyl]ethyl]-2-nitroethene-1,1-diamine | |
---|---|---|
Source | PubChem | |
URL | https://pubchem.ncbi.nlm.nih.gov | |
Description | Data deposited in or computed by PubChem | |
InChI |
InChI=1S/C12H20N4O3S/c1-13-7-10-3-4-11(19-10)9-20-6-5-15-12(14-2)8-16(17)18/h3-4,8,13-15H,5-7,9H2,1-2H3/b12-8+ | |
Source | PubChem | |
URL | https://pubchem.ncbi.nlm.nih.gov | |
Description | Data deposited in or computed by PubChem | |
InChI Key |
WZLBVRXZNDXPPW-XYOKQWHBSA-N | |
Source | PubChem | |
URL | https://pubchem.ncbi.nlm.nih.gov | |
Description | Data deposited in or computed by PubChem | |
Canonical SMILES |
CNCC1=CC=C(O1)CSCCNC(=C[N+](=O)[O-])NC | |
Source | PubChem | |
URL | https://pubchem.ncbi.nlm.nih.gov | |
Description | Data deposited in or computed by PubChem | |
Isomeric SMILES |
CNCC1=CC=C(O1)CSCCN/C(=C/[N+](=O)[O-])/NC | |
Source | PubChem | |
URL | https://pubchem.ncbi.nlm.nih.gov | |
Description | Data deposited in or computed by PubChem | |
Molecular Formula |
C12H20N4O3S | |
Source | PubChem | |
URL | https://pubchem.ncbi.nlm.nih.gov | |
Description | Data deposited in or computed by PubChem | |
Molecular Weight |
300.38 g/mol | |
Source | PubChem | |
URL | https://pubchem.ncbi.nlm.nih.gov | |
Description | Data deposited in or computed by PubChem | |
Product Name |
Desmethyl Ranitidine |
Synthesis routes and methods
Procedure details
Retrosynthesis Analysis
AI-Powered Synthesis Planning: Our tool employs the Template_relevance Pistachio, Template_relevance Bkms_metabolic, Template_relevance Pistachio_ringbreaker, Template_relevance Reaxys, Template_relevance Reaxys_biocatalysis model, leveraging a vast database of chemical reactions to predict feasible synthetic routes.
One-Step Synthesis Focus: Specifically designed for one-step synthesis, it provides concise and direct routes for your target compounds, streamlining the synthesis process.
Accurate Predictions: Utilizing the extensive PISTACHIO, BKMS_METABOLIC, PISTACHIO_RINGBREAKER, REAXYS, REAXYS_BIOCATALYSIS database, our tool offers high-accuracy predictions, reflecting the latest in chemical research and data.
Strategy Settings
Precursor scoring | Relevance Heuristic |
---|---|
Min. plausibility | 0.01 |
Model | Template_relevance |
Template Set | Pistachio/Bkms_metabolic/Pistachio_ringbreaker/Reaxys/Reaxys_biocatalysis |
Top-N result to add to graph | 6 |
Feasible Synthetic Routes
試験管内研究製品の免責事項と情報
BenchChemで提示されるすべての記事および製品情報は、情報提供を目的としています。BenchChemで購入可能な製品は、生体外研究のために特別に設計されています。生体外研究は、ラテン語の "in glass" に由来し、生物体の外で行われる実験を指します。これらの製品は医薬品または薬として分類されておらず、FDAから任何の医療状態、病気、または疾患の予防、治療、または治癒のために承認されていません。これらの製品を人間または動物に体内に導入する形態は、法律により厳格に禁止されています。これらのガイドラインに従うことは、研究と実験において法的および倫理的な基準の遵守を確実にするために重要です。